Cell Division Cycle, Mitosis and Meiosis PDF
Document Details
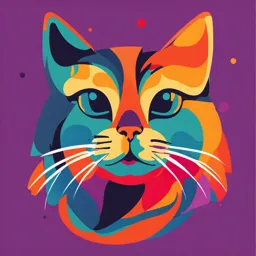
Uploaded by GratifyingRetinalite6029
Tags
Summary
This document describes the cell division cycle, mitosis and meiosis. It includes diagrams and explanations of chromosomes, cell division processes, and concepts of genetic inheritance. It also briefly covers Mendel's laws of inheritance.
Full Transcript
The cell-division cycle Cells reproduce by duplicating their contents and then dividing in two. This cell-division cycle is the fundamental means by which all living things are propagated. In unicellular species, such as bacteria and yeasts, each c...
The cell-division cycle Cells reproduce by duplicating their contents and then dividing in two. This cell-division cycle is the fundamental means by which all living things are propagated. In unicellular species, such as bacteria and yeasts, each cell division produces an additional organism. In multicellular species many rounds of cell division are required to make a new individual, and cell division is needed in the adult body, too, to replace cells that are lost by wear and tear or by programmed cell death. The details of the cell cycle may vary, but certain requirements are universal. First and foremost, to produce a pair of genetically identical daughter cells, the DNA must be faithfully replicated, and the replicated chromosomes must be segregated into two separate cells. The vast majority of cells also double their mass and duplicate all their cytoplasmic organelles in each cell cycle. Thus a complex set of cytoplasmic and nuclear processes have to be coordinated with one another during the cell cycle. The four successive phases of a standard eucaryotic cell cycle The successive phases of the cell cycle are: Interphase: This is the period between cell divisions and includes: G1 phase (Gap 1): The cell grows in size and synthesizes proteins and organelles. It prepares for DNA replication. This phase includes a checkpoint (G₁/S checkpoint) to ensure the cell is ready to enter the next phase. S phase (Synthesis): DNA replication occurs, producing two identical copies of each chromosome. Centrosomes (in animal cells) also duplicate in preparation for mitosis. G2 phase (Gap 2): Further cell growth and production of proteins required for mitosis. The cell checks for DNA replication errors and repairs them. A G₂/M checkpoint ensures the cell is ready to proceed to mitosis. M phase: Mitosis: The duplicated chromosomes are separated into two identical sets, forming two new nuclei. Cytokinesis: The cell divides into two daughter cells. During interphase the cell grows continuously; during M phase it divides. DNA replication is confined to the part of Cells in G1, if they have not yet committed themselves to interphase known as S phase. G1 phase is DNA replication, can pause in their progress around the the gap between M phase and S phase; G2 cycle and enter a specialized resting state, often called is the gap between S phase and M phase. G0(G zero), where they can remain for days, weeks, or even years before resuming proliferation. Checkpoints and inputs of regulatory information to the cell-cycle control system M-phase-promoting factors: cyclins and Cdk The cell-cycle control system is based on two key families of proteins. The first is the family of cyclin- dependent protein kinases (Cdk for short), which induce downstream processes by phosphorylating selected proteins on serines and threonines. The second is a family of specialized activating proteins, called cyclins, that bind to Cdk molecules and control their ability to phosphorylate appropriate target proteins. Cyclins are so called because they undergo a cycle of synthesis and degradation in each division cycle of the cell. There are two main classes of cyclins: mitotic cyclins, which bind to Cdk molecules during G2 and are required for entry into mitosis, and G1 cyclins, which bind to Cdk molecules during G1 and are required for entry into S phase. The events that drive the cell into mitosis are as follows: Mitotic cyclin accumulates gradually during G2 and binds to Cdk to form a complex known as M-phase- promoting factor (MPF). This complex is at first inactive, but through the action of other enzymes that phosphorylate and dephosphorylate it, it is converted to an active form. The ultimate activation of MPF is almost explosive. MPF is inactivated equally suddenly by the degradation of mitotic cyclin at the metaphase-anaphase boundary, enabling the cell to exit from mitosis. Quality control check points The G₁/S checkpoint: Cdk regulated by inhibitory proteins Inhibitory proteins controls entry in S-phase Several Cdk inhibitors negatively regulate the activity of cyclin-Cdk complexes to prevent premature progression through the G₁/S checkpoint. The G₂/M checkpoint: For M-Cdk to be active, inhibitory phosphates must be removed The key regulator of this transition is M- Cdk, which drives the cell from G₂ phase into M phase. For M-Cdk to become active M cyclin binds to M Cdk, forming the inactive M-Cdk complex. Wee1 kinase adds inhibitory phosphates on Cdk1, keeping it inactive. Cdc25 phosphatase removes these inhibitory phosphates, activating M-Cdk. DNA damage keeps Cdc25 inactive and stops cell from proceeding to M-phase. The Metaphase checkpoint: Cyclin proteolysis triggered by ubiquitinylation by Anaphase Promoting Factor (APC) allows exit from M- phase APC is activated once all chromosomes are properly aligned. The events of cell division as seen under a microscope https://www.youtube.com/watch?v=DwAFZb8juMQ Cohesins and Condensins The chromosome condensation is required for the subsequent organized segregation of the chromosomes into daughter cells, and it is accompanied by extensive phosphorylation of histone H1 molecules (up to six phosphates per molecule). Since histone H1 is present at a concentration of about one molecule per nucleosome and is known to be involved in packing nucleosomes together, its phosphorylation by MPF at the onset of the M phase is thought to contribute to chromosome condensation. Cohesins tie together the two adjacent sister chromatids in each duplicated chromosome. They are thought to form large protein rings that surround the sister chromatids, preventing them from coming apart, until the rings are broken late in mitosis. Condensin help coil each sister chromatid (or each DNA double helix) into a smaller, more compact structure that can be more easily segregated during mitosis. The cytoskeleton in M phase Chromosome condensation is the prelude to two distinct mechanical processes: (1) mitosis – the segregation of the chromosomes and the formation of two nuclei in place of one - and (2) cytokinesis - the splitting of the cell as a whole into two. These processes are carried out by two distinct cytoskeletal structures that appear transiently in M phase. The first to form is a bipolar mitotic spindle, composed of microtubules and their associated proteins. The mitotic spindle aligns the replicated chromosomes in a plane that bisects the cell; each chromosome then separates into two daughter chromosomes, which are moved by the spindle to opposite spindle poles. The second cytoskeletal structure required in M phase in animal cells is a contractile ring of actin filaments and myosin-II that forms slightly later, just beneath the plasma membrane in a plane perpendicular to the axis of the spindle; as the ring contracts it pulls the membrane inward so as to divide the cell in two, thereby ensuring that each daughter cell receives not only one complete set of chromosomes but also half of the cytoplasmic constituents in the parental cell. The two cytoskeletal structures contain different sets of proteins and can be formed independently of each other in some specialized cells. Their formation is usually closely coordinated, however, so that cytoplasmic division (cytokinesis) occurs immediately after the end of nuclear division (mitosis). Sexual Life Cycle The sexual life cycle of cells involves alternating between haploid and diploid stages, with meiosis producing haploid gametes and fertilization fusing them to form a diploid zygote, which then undergoes mitosis to develop into a multicellular organism. Haploid: Cells with a single set of chromosomes (n). Diploid: Cells with two sets of chromosomes (2n). Meiosis: A type of cell division that reduces the number of chromosomes in half, producing haploid gametes (sperm and egg cells). Fertilization: The fusion of two haploid gametes (sperm and egg) to form a diploid zygote. Zygote Development: The zygote undergoes mitosis (cell division) and differentiation to develop into a multicellular organism. Examples of Sexual Life Cycles: Diploid-dominant: Most animals, including humans, have a life cycle where the multicellular diploid stage is the most prominent. Haploid-dominant: Fungi and some algae have a life cycle where the multicellular haploid stage is the most prominent. Alternation of Generations: Plants and some algae have a life cycle that alternates between a multicellular diploid (sporophyte) and haploid (gametophyte) stage. With the exception of the chromosomes that determine sex (the sex chromosomes), a diploid nucleus contains two closely similar versions of each of the other chromosomes (the autosomes), one from the male parent (paternal chromosome) and one from the female parent (maternal chromosome). The two versions are called homologues, and in most cells they maintain a completely separate existence as independent chromosomes. When each chromosome is duplicated by DNA replication, the twin copies of the fully replicated chromosome at first remain closely associated and are called sister chromatids. In an ordinary cell division, the sister chromatids line up on the spindle during mitosis with their kinetochore fibers pointing toward opposite poles. The sister chromatids then separate from each other at anaphase to become individual chromosomes. In this manner each daughter cell formed by ordinary cell division inherits one copy of each paternal chromosome and one copy of each maternal chromosome. In contrast, a haploid gamete produced by the divisions of a diploid cell during meiosis must contain half the original number of chromosomes - only one chromosome in place of each homologous pair of chromosomes - so that the gamete is endowed with either the maternal or the paternal copy of each gene but not both. This requirement makes an extra demand on the machinery for cell division. The mechanism that has evolved to accomplish the additional sorting requires that homologues recognize each other and become physically paired before they line up on the spindle. This pairing of the maternal and the paternal copy of each chromosome is unique to meiosis. Each chromosome replicates to produce two sister chromatids as in an ordinary cell division. Rather than separating, the sister chromatids behave as a unit, as if chromosome duplication had not occurred: each duplicated homologue pairs with its partner, forming a structure called a bivalent, which contains four chromatids. The pairing allows genetic recombination to occur, whereby a fragment of a maternal chromatid may be exchanged for a corresponding fragment of a homologous paternal chromatid. The bivalents line up on the spindle, and at anaphase the two duplicated homologues (each consisting of two sister chromatids) separate and move to opposite poles. Because the joined sister chromatids behave as a unit, each daughter cell inherits two copies of one of the two homologues when the meiotic cell divides; these two copies are identical except where genetic recombination has occurred. The two progeny of this division (division I of meiosis) therefore contain a diploid amount of DNA but differ from normal diploid cells in two ways: (1) both of the two DNA copies of each chromosome derive from only one of the two homologous chromosomes in the original cell (except where there has been genetic recombination), and (2) these two copies are inherited as closely associated sister chromatids, as if they were a single chromosome. Formation of the actual gamete nuclei can now proceed simply through a second cell division, division II of meiosis, without further DNA replication. The chromosomes align on a second spindle and the sister chromatids separate, as in normal mitosis, to produce cells with a haploid DNA content. Meiosis thus consists of two cell divisions following a single phase of DNA replication, so that four haploid cells are produced from each cell that enters meiosis. Genetic Reassortment Is Enhanced by Crossing-over Between Homologous Nonsister Chromatids Unless they are identical twins, which develop from a single zygote, no two offspring of the same parents are genetically the same. This is because, long before the two gametes fuse, two kinds of randomizing genetic reassortment have occurred during meiosis. One kind of reassortment is a consequence of the random distribution of the maternal and paternal homologues between the daughter cells at meiotic division I, as a result of which each gamete acquires a different mixture of maternal and paternal chromosomes. From this process alone, one individual could, in principle, produce 2n genetically different gametes, where n is the haploid number of chromosomes. In humans, for example, each individual can produce at least 223 = 8.4 x 106 genetically different gametes. But the actual number of variants is very much greater than this because a second type of reassortment, called chromosomal crossing-over, occurs during meiosis. It takes place during the long prophase of meiotic division I, in which parts of homologous chromosomes are exchanged. On average, between two and three crossover events occur on each pair of human chromosomes during meiotic division I. This process scrambles the genetic constitution of each of the chromosomes in gametes. Chromosomal crossing-over involves breaking the DNA double helix in a maternal chromatid and in a homologous paternal chromatid so as to exchange fragments between the two nonsister chromatids in a reciprocal fashion by a process known as general genetic recombination. The consequences of each crossover event can be observed cytologically at the latest stages of prophase of meiotic division I, when the chromosomes are highly condensed. At this stage the sister chromatids are tightly apposed along their entire length. The two duplicated homologues (maternal and paternal) are seen to be physically connected at specific points. Each connection, called a chiasma (plural chiasmata), corresponds to a crossover between two nonsister chromatids. At this stage of meiosis, each pair of duplicated homologues, or bivalent, is held together by at least one chiasma. Many bivalents contain more than one chiasma, indicating that multiple crossovers can occur between homologues. During meiosis I, non sister chromatids in each bivalent swap segments of DNA A nick in a single DNA strand frees the strand, which then invades a homologous DNA double helix to form a short pairing region with one of the strands in the second helix. Only two DNA molecules that are complementary in nucleotide sequence can base-pair in this way and thereby initiate a general recombination event. During meiosis, heteroduplex joints are formed at the sites of crossing-over between homologous maternal and paternal chromosomes. If the maternal and paternal DNA sequences are slightly different, the heteroduplex joint may include some mismatched base pairs. The resulting mismatch in the double helix may then be corrected by the DNA repair machinery, which either can erase nucleotides on the paternal strand and replace them with nucleotides that match the maternal strand or vice versa. The consequence of this mismatch repair will be a gene conversion. Gene conversion can also take place by a number of other mechanisms, but they all require some type of general recombination event that brings two copies of a closely related DNA sequence together. Because an extra copy of one of the two DNA sequences is generated, a limited amount of DNA synthesis must also be involved. Genetic studies show that usually only small sections of DNA undergo gene conversion, and in many cases only part of a gene is changed. Prophase I Prophase I is the first phase of meiosis I, a crucial stage where homologous chromosomes pair, undergo synapsis (forming tetrads), and exchange genetic material through crossing over, leading to increased genetic diversity. Prophase I is further divided into five substages: leptotene, zygotene, pachytene, diplotene, and diakinesis, each characterized by specific events in chromosome pairing and recombination. Leptotene: Chromosomes become visible as thin threads. Zygotene: Homologous chromosomes pair up and synapsis begins. Pachytene: Synapsis is completed, and crossing over occurs. Diplotene: Homologous chromosomes begin to separate but remain attached at chiasmata. Diakinesis: Chromosomes condense further, and the nuclear envelope breaks down. Synaptonemal Complex The synaptonemal complex is a protein structure that holds homologous chromosomes together during meiosis. It helps in the exchange of genetic material between the chromosomes. It starts to form during the leptotene stage of meiosis. It is fully formed in the zygotene phase of Prophase I of Meiosis I. It starts to disappear by the end of the pachytene stage. It is a ladder-like structure with two lateral elements and a central region. It resembles railroad tracks. It helps in chromosome condensation, pairing, and transverse filament assembly. It prevents double-strand breaks from entering recombination pathways that involve sister chromatids. It holds homologous chromosomes together for crossing over to take place Chromosome alignment (at metaphase) and separation (at anaphase) in meiotic division I and meiotic division II. There are at least two major differences in the way chromosomes separate in meiotic division I and in normal mitosis. (1) During normal mitosis (and in meiotic division II, which resembles a normal mitosis) the sister chromatids are held together only at the centromere; the kinetochores (protein complexes associated with the centromeres) on each sister chromatid have attached kinetochore fibers pointing in opposite directions, so that the chromatids are drawn into different daughter cells at anaphase. At metaphase I of meiosis, by contrast, the kinetochores on both sister chromatids appear to have fused so that their attached kinetochore fibers all point in the same direction and the arms of the sister chromatids are closely apposed; moreover, the homologous maternal and paternal chromosomes are held together at the chiasmata. (2) During normal mitosis (and meiotic division II) the movement of chromatids to the poles is triggered by a mechanism that detaches the two sister kinetochores from each other (thus beginning anaphase), allowing the sister chromatids to segregate into different daughter cells. In anaphase I of meiosis, however, movement to the poles is initiated by the disruption of the poorly understood forces keeping the arms of sister chromatids together and by the simultaneous dissolution of the chiasmata linking the homologous maternal and paternal chromosomes; consequently, the sister chromatids remain paired, but the maternal and paternal homologues segregate into different daughter cells. Mendel and the laws of inheritence Mendel’s research showed that traits are inherited as discrete (individual) units: Traits are distinguishing characteristics that are inherited. (eye color, hair color). Genetics is the study of biological inheritance patterns and variation. Gregor Mendel showed that traits are inherited as discrete units. Like different colored marbles mixed together that can still be picked out separately. Mendel crossed many pea plants and observed seven traits: Mendel crossed many pea plants and observed seven traits. His results led to important conclusions. The same gene can have many versions: A gene is a piece of DNA that directs a cell to make a certain protein. Each gene has a locus, a specific position on a pair of homologous chromosomes. Each parent donates one allele for every gene: An allele is any alternative form of a gene occurring at a specific locus on a chromosome. (gene=pea shape, alleles= wrinkled or smooth). Each parent donates one allele for every gene. Genotype: The genetic makeup of a specific set of genes Phenotype: The physical characteristics of an individual – what you actually SEE- Homozygous (purebred) describes two alleles that are the same at a specific locus. Ex: (RR or rr). Heterozygous (Hybrid) describes two alleles that are different at a specific locus Alleles can be represented using letters: A dominant allele is expressed as a phenotype (visible trait) when at least one allele is dominant. A recessive allele is expressed as a phenotype (visible trait) only when two copies are present. Dominant alleles are represented by uppercase letters; recessive alleles by lowercase letters. Mendel used pollen to fertilize selected pea plants: Ex.: Purple Flowers X White Flowers. He crossed the P (parent) generation to produce F1 (first filial or first set of offspring) generation. He interrupted the self-pollination process in the plants by removing male flower parts. Mendel controlled the fertilization of his pea plants by removing the male parts, or stamens. He then fertilized the female part, or pistil, with pollen from a different pea plant. Mendel then allowed the resulting plants to self-pollinate: Among the F1 generation, all plants had purple flowers – this is the phenotype (describes physical traits, what we can see). F1 plants are all heterozygous this is the genotype (describes the internal makeup of the genes, what we cannot see). Punnett squares illustrate genetic crosses: The Punnett square is a grid system for predicting all possible genotypes resulting from a cross. The axes represent the possible gametes of each parent.The boxes show the possible genotypes of the offspring. The Punnett square yields the ratio of possible genotypes and phenotypes. A monohybrid cross involves one trait: Monohybrid crosses examine the inheritance of only one specific trait. Phenotype (F1 Offspring): 100% Purple flowers ;Genotype (F1 Offspring): 100% Heterozygous Among the F2 generation (when the F1 generation is crossed with themselves), some plants had purple flowers and some had white F2 Offsprings: Phenotype: 75% Purple, 25% White (3:1 Purple:White); Genotype: 25% Homozygous Dominant (FF); 50% Heterozygous (Ff); 25% Homozygous Recessive (ff); Ratio: 1:2:1 Homo Dom: Hetero: Homo Rec; ¼ Ho Do, ¼ Ho Rec, 2/4 Hetero. Both homozygous dominant and heterozygous genotypes yield a dominant phenotype: Ex: FF and Ff are both purple. The only combination that shows the recessive phenotype is when homozygous recessive is the genotype Ex: ff is white. Mendel drew three important conclusions: 1. Traits are inherited as discrete units. 2. Organisms inherit two copies of each gene, one from each parent. 3. The two copies segregate during gamete formation. The last two conclusions are called the law of segregation. Seven traits of Mendel Recessive alleles all follow the same Mendelian laws of inheritence Linked blocks of polymorphisms have been passed down from our ancestors When we compare the sequences of multiple human genomes, we find that any two individuals will differ in roughly 1 nucleotide pair in 1000. Most of these variations are common and relatively harmless. When 2 sequence variants coexist in the population and both are common, the variants are called polymorphisms. The majority of polymorphisms are due to substitution of a single nucleotide, called single nucleotide polymorphism (SNP). The rest are largely due to insertions and deletions – called indels, when the change is small, or copy number variations (CNVs) when it is large. Although these common variants can be found throughout the genome, they are not scattered randomly – or even independently. Instead they travel in groups called haplotype blocks – combinations of polymorphism that are inherited as a unit.