Synaptic Transmission 2023-4 - CNS PHYS
Document Details
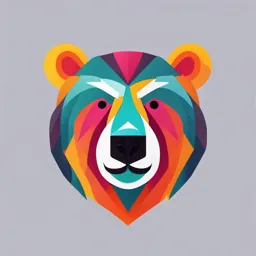
Uploaded by FormidablePennywhistle
RCSI (Royal College of Surgeons in Ireland)
null
Dr. Niamh Connolly, Dr. Colin Greengrass
Tags
Summary
This document is lecture notes on synaptic transmission for a central nervous system course. It describes the anatomy and function of synaptic terminals, common neurotransmitters and receptors, and the mechanisms of excitatory and inhibitory postsynaptic potentials. It also explains action potential generation and synaptic neurotransmission, including co-transmission.
Full Transcript
RCSI Royal College of Surgeons in Ireland Coláiste Ríoga na Máinleá in Éirinn Synaptic Transmission Class Med Year 2 Semester 1 Module Central Nervous System Code CNS Title Synaptic Transmission Lecturer Dr. Niamh Connolly (RCSI-IE): Dr. Colin Greengrass (RCSI-BH)...
RCSI Royal College of Surgeons in Ireland Coláiste Ríoga na Máinleá in Éirinn Synaptic Transmission Class Med Year 2 Semester 1 Module Central Nervous System Code CNS Title Synaptic Transmission Lecturer Dr. Niamh Connolly (RCSI-IE): Dr. Colin Greengrass (RCSI-BH) [email protected] [email protected] Date 31.10.2023 Learning Objectives Describe the function and anatomy of the synaptic terminal Describe common neurotransmitters and their receptors in the CNS Describe the mechanisms that generate excitatory and inhibitory postsynaptic potentials Describe the mechanisms and characteristics of an action potential Explain how summation results in action potential generation Describe the process of synaptic neurotransmission and co-transmission Characteristics Cell Body (Soma) of Neurons Contains nucleus and organelles Integrates incoming signals from dendrites. Dendrites Receive incoming signals from other Tree-like extensions Main receptive surfaces of the neuron. neurons Axon: A long projection extends from cell body. Transmits action potentials to other cells Synaptic Terminals The ends of axons form synapses Contain synaptic vesicles Synaptic Vesicles Store neurotransmitters released into the synaptic cleft in Small, membrane-bound sacs response to an action potential. Neurotransmitters Chemical messengers stored in synaptic vesicles. THIS IS THE SHAPE OF A GENERALISED NEURONE AT THE DENDRITE OF THE NEURONE, AT THE SYNAPSE OF THE NEURONE, THERE ARE LIGAND GATED SODIUM THERE ARE VOLTAGE GATED CALCIUM CHANNELS CHANNELS EACH OF THESE IS A PROTEIN STRUCTURE THE NEURONE AS AN EXAMPLE OF A NEED FOR PROTEIN SORTING AND AT THE AXON OF THE NEURONE, TRANSPORT THERE ARE VOLTAGE GATED SODIUM AND POTASSIUM CHANNELS & NA +/K+ PUMPS PROXIMAL REGIONS CELL BODY OF DENDRITES NUCLEOLUS (SOMA) NUCLEUS MITOCHONDRIA EXCEPT FOR THE GOLGI APP. SHAPE, NEURONAL CELL BODIES ARE MICROFILAMENT ALMOST IDENTICAL TO ENDOPLASMIC NORMAL CELLS RETICULUM MICROTUBULES HOWEVER, THEY HAVE NEUROFILAMENTS WHICH GIVE SHAPE TO AXON HILLOCK THE AXON AND NEUROFILAMENTS DENDRITES AXON Definition of a Action potential Membrane potential Synaptic Terminal The synaptic terminal is the distal end of an axon. It plays a crucial role in transmitting signals from one neuron to another or from a neuron to its target effector cells. Neurotransmitters are endogenous chemicals that transmit signals from a neuron to a target cell or another neuron across a synapse Neurotransmitters are contained in synaptic vesicles Voltage-gated Calcium Channels (VGCCs) Allow calcium ions to enter the synapse to cause neurotransmitter release. Anatomy of a Synaptic Synaptic Vesicles Terminal Mitochondria: provide the necessary ATP for the functions of the synapse Small, membrane-bound sacs filled with neurotransmitters. Active Zones Specialized regions where synaptic vesicles dock and prepare for release. SNARE Proteins Mediate fusion of synaptic vesicles with the presynaptic membrane Synaptic Cleft Allows diffusion of released neurotransmitters to the postsynaptic neuron. Neurotransmitter Receptors Bind to the neurotransmitters that have been released – initiate postsynaptic events Reuptake Transporters Protein channels located on the presynaptic membrane or nearby glial cells Neurotransmitter-Degrading Enzymes Enzymes located in the synaptic cleft and metabolise neurotransmitters Synaptic neurotransmission Electrochemical coupling at the synapse Sherwood, Intro to Human Physiology, Ch 4 IONOTROPIC VS METABOTROPIC RECEPTORS IONOTROPIC METABOTROPIC RAPID ACTION SLOWER ACTION LONGER DURATION – MEDIATE SHORT AND LONG-TERM EFFECTS SHORT LIVED AMPLIFIED RESPONSE LINKED TO ION CHANNELS CAUSE IONIC FLUX MULTIPLE ACTIONS IE. CHANGES TO GENE EXPRESSION SYNAPTIC CLEFT G PROTEIN POSTSYNAPTIC CELL CYTOPLASM Neurotransmitters Amino Acids Neurotransmitters are Acetylcholine (ACh) molecules in the nervous system that transmit Monoamines messages between neurons. Peptides Neurotransmitters can be Purines broadly categorized based Gaseous neurotransmitters on their structure and function. Trace Amines Neurotransmitters – Amino Acids Amino Acids are the primary excitatory and inhibitory neurotransmitters in the central nervous system. Gamma- Glycine: An aminobutyric inhibitory acid (GABA): The neurotransmitter primary , mainly found in inhibitory the spinal cord neurotransmitter and brainstem. in the CNS Glutamate: The Aspartate: Has primary an excitatory role excitatory but is less neurotransmitter widespread than in the CNS glutamate. Major CNS Neurotransmitters Neurotransmitter Receptors CNS Functions CNS pathologies Ionotropic Metabotropic Acetylcholine (ACh) Nicotinic Muscarinic Consciousness Alzheimer’s (NM, NN) (M1-M5) Cognitive processes Dopamine (DA) - D1-like (D1,D5) Intentional motor skills Parkinson’s, Depression, D2-like (D2,D3,D4) Reward-motivation Schizophrenia, Drug addiction GABA GABAA GABAB Inhibition Epilepsy, Anxiety Glutamate NMDA, AMPA, mGluR1-8 Excitation Excitotoxicity, Alzheimer’s, Kainate, Delta Synaptic plasticity, Schizophrenia learning, memory (Nor)adrenaline (NA) - α1, α2 Attention, arousal, mood Depression, ADHD, PTSD β1,β2 Serotonin (5-HT) 5-HT3 5-HT1,2,4-7 Mood, appetite, sleep Depression, Anxiety, OCD GLUTAMATE IN THE BRAIN Glutamate is the most important transmitter for brain function In many brain regions, 50-80% of neurons are glutamatergic Essential neurotransmitter in synaptic plasticity, learning and memory, and higher processing Excessive glutamate signalling can cause seizures and excitotoxicity https://doi.org/10.1093/brain/awr028 PET images of 11C NMDA-R MARKER uptake from a healthy volunteer, showing cortical (A), basal ganglia (B), and cerebellar (C) uptake Neurotransmitters – Glutamate Receptors Ionotropic receptors: NMDA, AMPA, and kainate receptors (ion channel) Metabotropic receptors: mGluR (G-protein linked) NMDA RECEPTORS A SINGLE MAGNESIUM ION SITS INSIDE THE CHANNEL GLUTAMATE AND GLYCINE MOLECULES BIND TO SPECIFIC BINDING SITES ON THE RECEPTOR Only under conditions of local membrane depolarization, Mg2+ is displaced THE MAGNESIUM ION IS BLOCKING THE CHANNEL Mg2+ CAN BE REMOVED IF THE INTERCELLULAR CURRENT IS +++ TAKING A STEP BACK – AMPA RECEPTORS AMPA NMDA Sodium ions (or at least a positive charge) is required to displace the magnesium ion from the NMDA receptor This influx of sodium ions comes from activation of AMPA receptors which are usually found to coexist with NMDA receptors Activated AMPA receptors permit entry of Na+ Activated AMPA receptors permit entry of Na+ Ca2+ within the postsynaptic neuron acts as a second messenger to activate intracellular signaling cascades Neurotransmitters – GABA Function Gamma-Aminobutyric Acid or GABA A GABA is the primary inhibitory neurotransmitter in the CNS, playing key roles in learning and memory GABAA receptors have multiple pharmacological roles GABA A (ionotropic, chloride channels) GABA B (metabotropic, G-protein coupled). Neurotransmitters – GABA Receptors Neurotransmitters – Acetylcholine (ACh) Function Acetylcholine plays several key roles in the peripheral nervous system and central nervous system. Acetylcholine is involved in muscle contraction, arousal, attention, learning, and memory. Nicotinic receptors are ion Neurotransmitters – channel linked and when Acetylcholine (ACh) Receptors activated, control the influx of sodium ions + Nicotinic Muscarinic Ion G-protein channels coupled Neurotransmitters – Monoamines Monoamines are derived from aromatic amino acids and include: Catecholamines Indolamines Dopamine (DA) Serotonin (5-HT) Noradrenaline (NA) Histamine Adrenaline (A) Dopamine (DA) Function: Involved in mood, reward, pleasure, and motor function. Receptors: Five types, all G-protein coupled, split into D1-like (D1 and D5) and D2-like (D2, D3, D4) Noradrenaline (NA) or Norepinephrine (NE) Function: Involved in arousal, attention, mood, and stress responses. Receptors: Alpha (α1, α2) and Beta (β1, β2, β3) adrenergic receptors. Serotonin (5-HT) Function: Involved in mood regulation, appetite, sleep, and learning. Receptors: Numerous types (e.g., 5-HT1, 5-HT2), most are G- protein coupled except for 5- HT3, which is ionotropic. Histamine Function: Involved in wakefulness and inflammatory responses. Receptors: H1, H2, H3, and H4 receptors. CCK Neurotransmitters – Neuropeptides Vasopressin Cholecystokinin Endorphins Enkephalins Substance P Neuropeptide Y Somatostatin Oxytocin (Antidiuretic (CCK) Hormone, ADH) Modulates Involved in appetite Regulates Modulates Involved in pain social water satiety modulation behaviour balance Involved in Inhibits the Modulate pain Modulates release of pain transmission circadian many perception in the spinal rhythms hormones in cord the brain Involved in Involved in Produce Involved in childbirth, social euphoria Involved in anxiety and neonatal behaviour anxiety bonding Neurotransmitters – Purines Purines play a role in a variety of body functions. Adenosine Adenosine triphosphate (ATP) Gaseous Neurotransmitters Gaseous neurotransmitters are unique because they are not stored in synaptic vesicles and are produced on demand Nitric Oxide Carbon Hydrogen (NO) Monoxide (CO) Sulphide (H2S) Neurotransmitters – Trace Amines Trace Amines Beta-phenylethylamine (PEA) are related to the classic monoamines Tyramine but are present in the brain at Tryptamine much lower levels. Octopamine NEUROTRANSMITTER SYNTHESIS Synthesised locally within the axon terminal Precursors necessary for synthesis are either: taken up by selective transporters on the membrane of the terminal readily available by-products of cellular processes that take place within the neuron itself Enzymes to synthesise neurotransmitters from these precursors are usually produced in the cell body and transported to the terminal by slow axonal transport THE MEMBRANE POTENTIAL THE RESTING POTENTIAL IS ACTUALLY MEASURED AS A NEGATIVE VALUE. THIS IS BECAUSE THERE IS A HIGHER CONCENTRATION OF NEGATIVELY CHARGED IONS (ANIONS) INSIDE THE CELL m 0mV –70mV ORGANIC ANIONS PHOSPHATE ANIONS THE MEMBRANE POTENTIAL MEASURE THE DIFFERENCE IN CHARGES BETWEEN THE INSIDE OF THE CELL AND THE OUTSIDE. AT REST, THE INSIDE OF THE CELL IS MUCH MORE NEGATIVELY CHARGED THAT THE OUTSIDE, AROUND –70mV (MILLIVOLTS) – ALTHOUGH THIS VALUE VARIES MARKEDLY BETWEEN NEURONES THE RESTING POTENTIAL m 0 mV EXTRACELLULAR -70 mV INTRACELLULAR Because there are many organic anions and PO4 inside and there are more sodium ions outside The difference in voltage inside compared to outside is –70mv (this is called potential difference) THE RESTING POTENTIAL this refers to the membrane potential when the cell is at rest. the membrane potential is controlled by differing ion concentrations on each side of the membrane Potential difference is the different in charge between one side of the membrane and the other At rest, there are more sodium ions (Na +) outside the cell and more potassium ions (K+) inside. Ions usually will diffuse from an area of their higher The number of positive concentration to an area of the lower charges of Na+ and K+ are concentration but here there are no ion channels almost in equilibrium in the open so ions cannot cross the membrane resting neurone THE AXON THE AXON CONSISTS OF AN EXTENDED PROCESS, ON ITS MEMBRANE IS A HIGH CONCENTRATION OF VOLTAGE GATED ION CHANNELS POTASSIUM CHANNEL SODIUM CHANNEL SODIUM/POTASSIUM THE AXOLEMMA PUMP THE CYTOPLASM OF THE AXON CONTAINS ELEMENT OF THE CYTOSKELETON WHICH GIVES SHAPE TO THE AXON AND PROVIDES A TRANSPORT NETWORK Concentration and Electrochemical Gradients in Membrane Transport Extracellular Intracellular The concentration gradient drives ions across a membrane through open channels, facilitating their movement K+ 5 mM 150 mM K+ from areas of higher concentration to areas of lower concentration. The electrochemical gradient Na+ 145 mM 15 mM Na+ − considers the electrical ORGANIC potential across the membrane. ANIONS For example, positively − charged ions would be attracted towards the side Cl- 115 mM 10 mM Cl- of the membrane with a more negative electrical potential => Ion concentration gradients + ion charge gradients => electrochemical gradient across the membrane => membrane potential ▪ MORE POSITIVE MORE NEGATIVE 10 -90 -70 -50 -30 -10 ▪ Membrane Potential (mV) DECREASED POTENTIAL DIFFERENCE. WHAT WILL HAPPEN TO THE POTENTIAL DIFFERENCE IF POTASSIUM CHANNELS OPEN? 10 ▪ POTASSIUM IONS WILL LEAVE THE CELL Potential (mV) DOWN THEIR CONCENTRATION -10 Membrane INCREASED POT. DIFF. GRADIENT -30 -50 -70 -90 ▪ THE CONCENTRATION OF POTASSIUM IONS INSIDE THE CELL WILL DECREASE ▪ When there is an action potential and the outside of the neuron becomes more negative ▪ THE POTENTIAL DIFFERENCE WILL than the inside, potassium ions will also leave INCREASE AGAIN the cell down their electrochemical gradient EXCITATORY AND INHIBITORY SYNAPSES Positively Negatively ACETYLCHOLINE charged charged GABA sodium ion chloride ion + − SUMMATION AND THE INITIATION OF THE ACTION POTENTIAL WHEN LIGAND GATED SODIUM ION CHANNELS ARE OPEN BY A CHEMICAL NEUROTRANSMITTER, Na+ Ca2+ Na+ ENTERS THE NEURONE AND DIFFUSES THROUGH THE LIGAND GATED VOLTAGE GATED VOLTAGE GATED VOLTAGE GATED CYTOPLASM Na+ CHANNEL Na+ CHANNEL K+ CHANNEL Ca2+ CHANNEL SYNAPSE AXON HILLOCK AXON CELL BODY ACTIVATION OF VOLTAGE GATED Na+ CHANNELS, CAUSES AN INFLUX OF Na+ WHICH CAN IN TURN ACTIVATE ADJACENT VOLTAGE GATED Na+ DENDRITES CHANNELS ALTHOUGH Na+ ENTERING THE AXON, WILL DIFFUSE IN ALL IF ENOUGH Na+ DIFFUSE TO THE AXON HILLOCK, DIRECTIONS. THE ION CHANNELS TO THE LEFT (CELL BODY END) ARE DEACTIVATED AND ONLY CHANNELS LEADING TO THE SYNAPTIC WHERE THERE IS A HIGH CONCENTRATION OF VOLTAGE GATED Na+ CHANNELS, THESE TERMINAL CAN BE ACTIVATED THIS ENSURES THAT THE ACTION POTENTIAL TRAVELS IN ONE CHANNELS CAN THEN BE ACTIVATED DIRECTION ONLY EXCITATORY AND INHIBITORY SYNAPSES Neurotransmitter binding Neurotransmitters at an excitatory synapse synaptic cleft opens Na+ channel depolarise the post-synaptic membrane -70 -60 -56- Binding of Acetylcholine (ACh) to its receptors Postsynaptic - -56 -60 -70 on the post-synaptic membrane activates membrane Na+ Na+ ligand-gated Na+ channels Na+ Na+ influx depolarises the post-synaptic membrane potential Neurotransmitters at an inhibitory synapse hyperpolarise the post-synaptic membrane Neurotransmitter binding synaptic cleft opens Cl- channel Binding of GABA to GABAA receptors on the post-synaptic membrane activates ligand- Postsynaptic -70 -70 -80- - -80 -70 -70 gated Chloride (Cl-) channels membrane Cl- Cl- Cl- influx hyperpolarises the post-synaptic Cl- membrane potential Excitatory post-synaptic potential (ePSP) Ligand-gated ion channel (Na+) synaptic cleft + + + + + + + Resting membrane Postsynaptic - - - - - - - potential membrane Neurotransmitter ( ) binding Mechanism: opens Na+ ( ) channel Neurotransmitters (e.g., glutamate) bind to Small postsynaptic receptors (e.g., AMPA or NMDA receptors). -70 -60 -56- - -56 -60 -70 depolarisation This binding leads to the opening of ion channels that allow positively charged ions Na+ Na+ (like Na⁺ or Ca²⁺) to flow into the neuron. This influx of positive ions causes a Na+ depolarization, making the neuron more likely to generate an action potential. Significance: EPSPs increase the likelihood that the postsynaptic neuron will fire an action potential. The summation of EPSPs from various presynaptic inputs can lead the postsynaptic neuron to reach its threshold and fire an action potential. inhibitory post-synaptic potential (iPSP) Ligand-gated ion channel synaptic cleft + + + + + + + Resting membrane Postsynaptic - - - - - - - potential membrane Neurotransmitter binding opens K+ channel Hyper- -70 -70 -80- -80 -70 -70 polarisation Inhibitory Postsynaptic Potentials (IPSPs): K+ K+- K+ Mechanism: Neurotransmitters (e.g., GABA for GABA_A receptors or glycine for glycine receptors) bind to specific postsynaptic receptors. Neurotransmitter binding This binding results in the opening of ion opens Cl- channel channels that either allow the influx of Hyper- negatively charged ions (like Cl⁻) or the efflux of positively charged ions (like K⁺). -70 -70 -80- - -80 -70 -70 polarisation This movement of ions causes a hyperpolarization, making the neuron less Cl- Cl- Cl- likely to generate an action potential. Significance: IPSPs decrease the likelihood that the postsynaptic neuron will fire an action potential. They counteract the effects of EPSPs and regulate the overall excitability of the neuron. Action Potential 50 Action Potential 40 30 Membrane Potential (mV) 20 Excitatory Postsynaptic Potential (EPSP) Repolarisation +40 to -70 mV 10 If depolarization (with EPSPs) Depolarisation-70 to +40 mV Inhibitory Postsynaptic reaches the action potential 0 threshold (-55 mV) an action Potential (IPSP) potential is generated at the -10 axon hillock -20 -30 -40 Action Potential Threshold -50 -60 Resting Membrane Potential IPSP -70 EPSP EPSP EPSP -80 Hyperpolarisation -90 0 0.2 0.4 0.6 0.8 1 1.2 Time (m/s) THE ACTION POTENTIAL – WHAT IS HAPPENING TO CHANNELS/PUMPS AT EACH STAGE 50 Membrane Potential (mV) 30 Na+ K+ PUMP LIGAND GATED VOLTAGE GATED VOLTAGE GATED 10 ACTIVE Na CHANNEL Na+ CHANNEL + K+ CHANNEL OPEN OPEN OPEN -10 -30 LIGAND GATED VOLTAGE GATED VOLTAGE GATED Na+ CHANNEL Na+ CHANNEL K+ CHANNEL CLOSED CLOSED CLOSED -50 RESTING STATE RESTING STATE -70 -90 HYPERPOLARISATION THE ACTION POTENTIAL – WHAT IS HAPPENING TO IONS Na+ K+ 50 Na+ ENTERS THE NEURONE INCREASING THE K+ LEAVES THE NEURONE INTRACELLULAR DECREASING THE CONCENTRATION OF INTRACELLULAR 30 POSITIVE IONS CONCENTRATION OF POSITIVE IONS 10 -10 -30 -50 RESTING STATE RESTING STATE -70 -90 HYPERPOLARISATION VOLTAGE GATED SODIUM ION CHANNELS AT THE RESTING POTENTIAL + POSITIVELY SODIUM CHARGED ION + + + + + + + + + + + 0mV RESTING POTENTIAL + ++ ++ -70mV + ++ POSITIVELY CHARGED POSITIVELY CHARGED INACTIVATION GATE AMINO ACIDS INITIATION OF THE ACTION POTENTIAL IN NEURONS SPECIFICALLY AT THE AXON HILLOCK; THE CELL IS EXCITED BY INCREASING [SODIUM ION] (HOW THIS HAPPENS WE WILL SEE LATER) + + + + + + + + + + + 0mV ACTION POTENTIAL + + + + + -55mV + + + + + + SOME POSITIVE SODIUM IONS INSIDE THE NEURONE CAUSE ION CHANNELS TO OPEN THIS CAUSES POSITIVE SODIUM IONS FROM OUTSIDE TO ENTER THE NEURON THROUGH THE ION CHANNEL DOWN THEIR CONCENTRATION GRADIENT + + + + + + + + 0mV ++ + ─ ─ ─ ─ ++ + + + +20mV ACTION POTENTIAL + + + THE ACTION POTENTIAL THE ACTION POTENTIAL THE INACTIVATION GATE CLOSES THE CHANNEL + + + + + + ACTION POTENTIAL 0mV ++ ─ ─ ++ ─ ─ + + +40mV + + + + + + + A HIGH [SODIUM ION] PUSHES THE INACTIVATION GATE TO SHUT THE CHANNEL THE ACTION POTENTIAL THE INACTIVATION GATE CLOSES THE CHANNEL ▪ A HIGH [SODIUM ION] PUSHES THE INACTIVATION GATE TO SHUT THE CHANNEL – THIS GATE REMAINS SHUT FOR SOME TIME + + + + + + 0mV + + + ─ ─ + ─ ─ +50mV ACTION POTENTIAL + + + + + + + + + ▪ AS THE INACTIVATION GATE CONTAINS MANY POSITIVELY CHARGED AMINO ACIDS, ▪ IT IS REPELLED BY THE INCREASED POSITIVE CHARGE INSIDE THE CELL ▪ IT IS ATTRACTED A LITTLE BY THE NEGATIVE CHARGES INSIDE THE CHANNEL PORE VOLTAGE GATED SODIUM ION CHANNELS HOW THEY WORK (concept) INTRACELLULAR POSITIVE CHARGE INCREASES ION CHANNEL IS ION CHANNEL IS CLOSED ION CHANNEL OPENS DEACTIVATED OTHER VOLTAGE GATED ION CHANNELS HOW THEY WORK (CONCEPTUAL BASIS) POTASSIUM AND CALCIUM CHANNELS DO NOT HAVE A DEACTIVATION GATE INTRACELLULAR POSITIVE CHARGE DECREASES ION CHANNEL IS ION CHANNEL IS CLOSED ION CHANNEL OPENS CLOSED ACTION POTENTIALS ARE UNIDIRECTIONAL 1. DEPOLARISATION AT A OPENED THE CHANNEL CAUSING INFLUX OF SODIUM IONS 2. THESE IONS DIFFUSED IN ALL DIRECTIONS 3. SOME OF THESE IONS REACHED THE ION CHANNEL B AND CAUSED IT TO OPEN 4. IN THE MEANTIME CHANNEL A IS CLOSED VIA THE ACTIVATION GATE 5. IONS FROM B ENTER AND SPREAD BY DIFFUSION IN ALL DIRECTIONS 6. HOWEVER THEY CANNOT ACTIVATE A AS THIS IS CURRENTLY DEACTIVATED | THEY CAN ONLY ACTIVATE C + + + + + + + + + + + + A + + B + C + + + + + + + + + + + + + + ++ ++ + + + + + + + + + + + + + + + + + DIRECTION OF ACTION POTENTIAL +50mV 0mV -70mV HYPERPOLARISATION m 0 mV EXTRACELLULAR -90 mV INTRACELLULAR VOLTAGE GATED SODIUM ION + POSITIVELY SODIUM CHARGED ION CHANNELS AT THE HYPERPOLARISED POTENTIAL + POSITIVELY POTASSIUM CHARGED ION + + + + + + + + + + + 0MV RESTING POTENTIAL + + ++ ++ -90MV + + ++ + POSITIVELY CHARGED POSITIVELY CHARGED INACTIVATION GATE AMINO ACIDS AT HYPERPOLARISATION THE CONCENTRATION GRADIENT IS NO LONGER PRESENT. HYPERPOLARISATIO IN ORDER TO MAKE ANOTHER ACTION POTENTIAL THIS GRADIENT MUST BE RESTORED N K+ K+ K+ K+ Na Na Na Na + + + + Na Na Na Na K+ K+ K+ K+ + + + + WHAT WE HAVE WHAT WE NEED Na Na Na Na Na Na K+ K+ + + + + + + Na Na K+ K+ K+ K+ K+ K+ + + SODIUM POTASSIUM PUMP THE SODIUM POTASSIUM THIS IS NEEDED IN ORDER THAT PUMP USES ATP TO RESTORE OTHER ACTION POTENTIALS THE CONCENTRATION GRADIENT OF SODIUM AND CAN HAPPEN POTASSIUM IONS AT RESTING POTENTIAL THE PUMP, SODIUM/POTASSIUM ATPASE, EXCHANGES SODIUM (OUT) WITH POTASSIUM (IN) Refractory period Refractory Periods: Absolute Refractory Period: A period during which a second action potential is impossible to elicit, regardless of the stimulus strength. Voltage-gated Na+ channels are in an inactive state Relative Refractory Period: A period during which a stronger- than-usual stimulus is needed to initiate another action potential MRI MAP OF MYELINATED NEURONE DENSITY IN THE BRAIN THE PROCESS OF MYELINATION For effective transmission of neuronal current, like a package of positive ions travelling along the neurone, the higher the velocity (speed) of transmission the better the function, especially in neurones with long axons (such as motor neurones) The speed which the current travels along the axon is called the conduction velocity SOME SODIUM IONS WILL STICK TO THE MEMBRANE OR CAPACITANCE MOVEMENT WILL BE HINDERED BY POSITIVE IONS HELD THERE ------------ The axonal membrane is very thin. As a result of this, there is a capacitance across the membrane, where positively charged ions, including sodium are attracted to the charges outside the cell This example is of capacitance is occurring during an action potential. What effect could this have on conduction velocity? LEAKY CHANNELS AND CAPACITANCE ------------ There are channels in the membrane which are permeable to potassium ions, and to a lesser extent sodium (out) and chloride (in) ions These leaky channels, especially when there is a stronger attraction to the negative extracellular charge, will leak more K+ What will happen to the action potential if K+ leaves the neurone? THE ACTION POTENTIAL WILL BE DEGRADED AXON DIAMETER AND CONDUCTION VELOCITY 6 µM HERE ARE TWO AXONS WITH DIFFERENT DIAMETERS. WHICH OF THESE WILL CARRY THE ACTION POTENTIAL MOST EFFICIENTLY AND WHY? 13 15 µM AXON DIAMETER AND CONDUCTION VELOCITY 6 µM THE AXON WITH A LARGER DIAMETER WILL CARRY THE CURRENT MORE EFFICIENTLY BECAUSE THERE IS LESS RESISTANCE IN THIS AXON BASICALLY, THERE IS LESS IN THE WAY – ANALOGY: TRAFFIC FLOW CAN BE INCREASED BY WIDENING A ROAD 13 15 µM CONCEPT: OPENING CHANNELS TAKES TIME (ALTHOUGH NOT MUCH TIME) MYELINATION THE AXONS OF BOTH SENSORY AND MOTOR NEURONS ARE MYELINATED ALONG MOST OF THEIR LENGTH ACTING AS INSULATION, MYELIN SPEEDS TRANSMISSION ALONG AXONS AND THUS IS CRITICAL FOR QUICK REFLEX MOVEMENTS LIKE THE KNEE JERK. THE MYELIN SHEATH IS ARRANGED IN CONCENTRIC LAYERS OF LIPIDS INTERSPERSED BETWEEN PROTEIN LAYERS MYELIN HAS A COMPOSITION SIMILAR TO THAT OF PLASMA MEMBRANES, CONSISTING OF 70% LIPID AND 30% PROTEIN, WITH A HIGH CONCENTRATION OF CHOLESTEROL AND PHOSPHOLIPID. OLIGODENDROCYTES OLIGODENDROCYTES ENSHEATH SEVERAL AXON PROCESSES IN THE CENTRAL NERVOUS SYSTEM ARE FOUND ONLY IN THE CENTRAL NERVOUS SYSTEM, LAY DOWN A LAMINATED, LIPID-RICH WRAPPING CALLED MYELIN AROUND SOME, BUT NOT ALL, AXONS. MYELIN HAS IMPORTANT EFFECTS ON THE SPEED OF THE TRANSMISSION OF ELECTRICAL SIGNALS SCHWANN CELLS IN THE DEVELOPMENT OF THE PERIPHERAL NERVOUS SYSTEM, THE EXTERNAL CELL MEMBRANE OF SCHWANN CELLS SURROUNDS A SINGLE AXON ELONGATES AND SPIRALS AROUND THE AXON IN CONCENTRIC LAYERS THE CYTOPLASM OF THE SCHWANN CELL APPEARS TO BE SQUEEZED OUT DURING THE ENSHEATHING PROCESS WHEN THE SCHWANN CELL'S PROCESSES CONDENSE INTO THE COMPACT MATURE MYELIN SHEATH. MYELINATION INCREASES CONDUCTION VELOCITY MYELINATION IS THE ADDITION OF AN INSULATING LAYER WRAPPED AROUND SOME AXONS. THIS REDUCES BOTH THE CAPACITANCE AND THE LEAKINESS OF THE MEMBRANE. THEREFORE THE CURRENT IS CARRIED MORE EFFICIENTLY AND CONDUCTION VELOCITY IS HIGHER. THE NODE OF RANVIER THE NODE OF RANVIER IS A GAP BETWEEN MYELINATED REGIONS THIS AREA IS VERY RICH IN SODIUM AND BUT LESS SO IN POTASSIUM CHANNELS AND SODIUM/POTASSIUM PUMPS THIS AREA IS REQUIRED AS A BOOSTER FOR THE ACTION POTENTIAL WHICH ALTHOUGH FLOWING MORE EFFICIENTLY IN MYELINATED CELLS, WILL STILL DEGRADE IF THE AXON IS LONG (SIMPLY SODIUM IONS WILL BE GRADUALLY LOST TO THE AXOPLASM THE NODE OF RANVIER CAPACITANCE VERY LOW ACTION POTENTIAL TRAVELS WITH LESS RESISTANCE CAPACITANCE VERY LOW 1. ACTION POTENTIAL TRAVELS WITH LESS RESISTANCE WHEN FOLLOWING A PATH OF LESS RESISTANCE IT WILL HAVE A HIGHER CONDUCTION VELOCITY 2. AS THE ACTION POTENTIAL TRAVELS IT WILL LOSE SOME IONS – THUS THE SIGNAL WILL EVENTUALLY DEGRADE 3. AT THE NODE OF RANVIER, THE ACTION POTENTIAL TRIGGERS THE OPENING OF VOLTAGE GATED SODIUM CHANNELS 4. THIS BRINGS IN MORE SODIUM IONS AND HELPS TO RESTORE THE ACTION POTENTIAL COMPARISON OF CONDUCTION VELOCITY TYPE OF NEURONE FIBRE DIAMETER CONDUCTION (µm) VELOCITY (m/sec) MYELINATED LARGE 13-20 80-120 SMALL 6-12 35-75 VERY SMALL 1-5 5-30 UNMYELINATED TYPICAL RANGE 0.2-1.5 0.5-2 Presynaptic Terminal Synaptic vesicles filled with neurotransmitters Postsynaptic Membrane Synaptic cleft / synapse Gap between pre- and post- synaptic cells THIS PSEUDOCOLOURED IMAGE TAKEN WITH A SCANNING ELECTRON MICROSCOPE SHOWS AN AXON TERMINAL THAT WAS BROKEN OPEN TO THE CHEMICAL REVEAL SYNAPTIC VESICLES (BLUE AND ORANGE) INSIDE THE NEURON. (CREDIT: MODIFICATION OF WORK BY TINA CARVALHO, NIH-NIGMS) SYNAPSE VESICLES IN THE SYNAPTIC TERMINAL CONTAIN NEUROTRANSMITTER MOLECULES PRESYNAPTIC NEURON ME SICULAR MBRAN E E V SYNAPTIC VESICLES CONTAINING MOLECULES OF NEUROTRANSMITTER SYNAPTIC MEMBRANE SYNAPTIC CLEFT VESICLE-ASSOCIATED TRANSPORTER SMALL MOLECULE NEUROTRANSMITTERS ARE MOSTLY SYNTHESIZED IN THE CYTOPLASM OF THE SYNAPSE THEY ARE THEN TRANSPORTED INTO VESICLES THROUGH A VESICLE-ASSOCIATED TRANSPORTER (VAT) CYTOPLASM INTRAVESICULAR LUMEN Clear Core Vs Dense Core Vesicles Clear Vesicles: Typically Dense-core Vesicles: contain small-molecule Contain neuropeptides, neurotransmitters, such growth factors, and as glutamate, GABA, or some classical acetylcholine. neurotransmitters. Release of Neurotransmitters ACTION POTENTIAL PRESYNAPTIC NEURON (OR TERMINAL) Release of neurotransmitter molecules usually follows arrival of an action potential at the synapse, Release may also follow a graded electrical potential (Axoaxonic RELEASE Synapses) RELEASE RELEASE SYNAPTIC CLEFT POSTSYNAPTIC NEURON VOLTAGE GATED CALCIUM ION CHANNELS POSITIVELY CHARGED + + + + AMINO ACIDS RESTING POTENTIAL + + + + + + + + + + POSITIVELY CHARGED ION + CALCIUM ION + + + + + + + + + + + ACTION POTENTIAL + + + + VOLTAGE GATED CALCIUM ION CHANNELS + + + + + POSITIVELY CHARGED AMINO ACIDS + + + + + + + + + + + + + POSITIVELY CHARGED ION ▪ WHEN THE MEMBRANE DEPOLARISES THE ION CHANNEL OPENS VOLTAGE GATED CALCIUM ION CHANNELS + + + + + POSITIVELY CHARGED AMINO ACIDS + + + + + + + + ▪ WHEN THE MEMBRANE REPOLARISES THE ION CHANNEL CLOSES VESICULAR RELEASE ACTIVATED ACTION BY CALCIUM IONS POTENTIAL CALCIUM ION SODIUM ION CALCIUM IONS ENTER THE SYNAPSE THERE ARE NUMEROUS VESICLES LOCATED IN PRESYNAPTIC THE SYNAPTIC TERMINAL TERMINAL When an action potential THESE CONTAIN reaches the axon NEUROTRANSMITTERS terminal, voltage-gated THEY ALSO HAVE SEVERAL calcium (Ca²⁺) channels MOLECULES ON THEIR open, allowing Ca²⁺ influx. MEMBRANE POSTSYNAPTIC DENDRITE VESICULAR RELEASE ACTION ACTIVATED BY CALCIUM IONS POTENTIAL CALCIUM ION SODIUM ION CALCIUM IONS ENTER THE SYNAPSE CALCIUM IONS BIND TO ONE OF THE VESICLE PRESYNAPTIC SURFACE RECEPTORS, TERMINAL SYNAPTOTAGMIN POSTSYNAPTIC DENDRITE VESICULAR RELEASE ACTION ACTIVATED BY CALCIUM IONS POTENTIAL CALCIUM ION SODIUM ION CALCIUM IONS BIND TO ONE OF THE VESICLE SURFACE RECEPTORS, SYNAPTOTAGMIN THIS CAUSES THE VESICLES TO MOVE TOWARDS AND/OR FUSE WITH THE SYNAPSE MEMBRANE PRESYNAPTIC THERE ARE SEVERAL HYPOTHESES TERMINAL AS TO WHAT HAPPENS EXACTLY. POSTSYNAPTIC DENDRITE Vesicular Fusion Several vesicular membrane proteins, syntaxin, synaptobrevin and SNAP-25 form a complex Vesicular Fusion Several vesicular membrane proteins, syntaxin, synaptobrevin and SNAP-25 form a complex Vesicular Fusion Several vesicular membrane proteins, syntaxin, synaptobrevin and SNAP-25 form a complex Neurotransmitter molecules bind to receptors on the postsynaptic membrane And sometimes on the presynaptic membrane Ionotropic – Excitatory Vs Inhibitory Receptors Inhibitory channels cause an Excitatory channels cause an influx of negatively charged ions influx of positively charged ions Cl Na − + SYNAPTIC CLEFT POST-SYNAPTIC NEURONE AUTORECEPTORS Autoreceptors are located on presynatpic terminals They play a critical role in autoregulation of neurotransmission PRESYNAPTIC TERMINAL They may have varying functions To activate vesicular release through depolarising or hyperpolarising the synapse (prevent Ca2+ channels opening) To activate reuptake transporters And several others not covered here POSTSYNAPTIC DENDRITE PRESYNAPTIC ACTION CHLORIDE ION POTENTIAL CALCIUM ION RECEPTORS SODIUM ION In this example a presynaptic receptor linked to chloride channel is present PRESYNAPTIC TERMINAL When activated an influx of chloride ions, causing hyperpolarisation, prevent the opening of calcium channels Another mechanism is using K+ channels causing a potassium efflux POSTSYNAPTIC DENDRITE Autoreceptors – An Example GABAA RECEPTORS Found postsynaptically GABAB RECEPTORS GABAB PRESYNAPTIC TERMINAL Found presynaptically, and can GABAB activation inhibits inhibit synaptic release release of GABA This is an autoreceptor. Autoreceptors are sensitive to higher concentration of the same neurotransmitter GABAA POSTSYNAPTIC DENDRITE Neurotransmitter Deactivation Start here The action of neurotransmitters needs to be terminated after release Taken up by presynaptic neuron via transporter protein DIFFUSES Inactivated/degraded by enzymes in the synaptic cleft Taken up by glial cell Diffuses away into periphery NEUROTRANSMITTER PRESYNAPTIC TERMINAL REUPTAKE Specific transporter proteins remove most small-molecule neurotransmitters (or their metabolites) from the PRESYNAPTIC synaptic cleft. TERMINAL Thus, delivering them back to the presynaptic terminal for reuse These transporters are highly specific Reuptake also occurs at glial cells POSTSYNAPTIC PRESYNAPTIC CELL CYTOPLASM SYNAPTIC CLEFT Transporters usually utilise symport to transport neurotransmitters across the membrane of the presynaptic neuron. The neurotransmitter will bind to sodium ions, NOT ALL where the sodium ion will flow down its NEUROTRANSMITTERS concentration and electrical gradient. HAVE REUPTAKE TRANSPORTERS These forces will pull the neurotransmitter into the cell, against its own gradient NEUROTRANSMITTER PRESYNAPTIC TERMINAL DEGRADATION After a neurotransmitter has been secreted into the synaptic cleft, it must be removed This enables the postsynaptic cell to produce another synaptic event. PRESYNAPTIC TERMINAL Neurotransmitters can undergo degradation by specific enzymes. The metabolites of these reactions often undertake a similar reuptake mechanism They are subsequently recycled in the synapse thus making more of the required neurotransmitter POSTSYNAPTIC Enzymes clear neurotransmitter molecules from the synaptic cleft Not all neurotransmitters have enzymes in the synaptic cleft SYNAPTIC CLEFT POSTSYNAPTIC CELL CYTOPLASM NEUROTRANSMITTER PRESYNAPTIC TERMINAL DEGRADATION Often neurotransmitters after reuptake back into the synaptic terminal They are subsequently recycled in the synapse PRESYNAPTIC thus making more of the TERMINAL required neurotransmitter POSTSYNAPTIC Neurotransmitter Deactivation Just some examples There are differences Start here between life-cycles of neurotransmitters All of these enzymes, receptors, and transporters are pharmacological (drug) targets Drug Targets – What PRESYNAPTIC TERMINAL would happen? A What would happen if you What is A? blocked it? E What would happen if you What is B? blocked it? PRESYNAPTIC TERMINAL What would happen if you What is C? blocked it? REUPTAKE B ALSO What would happen if you OCCURS AT What is D? blocked it? GLIAL CELLS What would happen if you What is E? blocked it? C D POSTSYNAPTIC ACTION Co-neurotransmission POTENTIAL CALCIUM ION SODIUM ION Packaging Different neurotransmitters can be PRESYNAPTIC packaged in the same or different TERMINAL ACh vesicles VIP Release: Depending on the frequency or strength of action potentials, one or both neurotransmitters might be released. This is an example of acetylcholine (ACh) which is sometime co- released with vasoactive-intestinal peptide (VIP) POSTSYNAPTIC DENDRITE ACTION Co-neurotransmission POTENTIAL CALCIUM ION SODIUM ION Usually, fast response is mediated via classical NTs (neurotransmission) and slow PRESYNAPTIC TERMINAL response via neuropeptides ACh (neuromodulation) VIP Allows for more complex, flexible effects Classical neurotransmitters are usually packaged in clear core vesicles Neuropeptides are stored in large dense-core vesicles POSTSYNAPTIC DENDRITE EPSPs, IPSPs and Summation SUMMATION AND THE INITIATION OF THE ACTION POTENTIAL WHEN LIGAND GATED SODIUM ION CHANNELS ARE OPEN BY A CHEMICAL NEUROTRANSMITTER, Na+ Ca2+ Na+ ENTERS THE NEURONE AND DIFFUSES THROUGH THE LIGAND GATED VOLTAGE GATED VOLTAGE GATED VOLTAGE GATED CYTOPLASM Na+ CHANNEL Na+ CHANNEL K+ CHANNEL Ca2+ CHANNEL SYNAPSE AXON HILLOCK AXON Graded CELL BODY potentials ACTIVATION OF VOLTAGE GATED Na+ CHANNELS, CAUSES AN INFLUX OF Na+ WHICH CAN IN TURN ACTIVATE ADJACENT VOLTAGE GATED Na+ DENDRITES CHANNELS ALTHOUGH Na+ ENTERING THE AXON, WILL DIFFUSE IN ALL IF ENOUGH Na+ DIFFUSE TO THE AXON HILLOCK, DIRECTIONS. THE ION CHANNELS TO THE LEFT (CELL BODY END) ARE DEACTIVATED AND ONLY CHANNELS LEADING TO THE SYNAPTIC WHERE THERE IS A HIGH CONCENTRATION OF VOLTAGE GATED Na+ CHANNELS, THESE TERMINAL CAN BE ACTIVATED THIS ENSURES THAT THE ACTION POTENTIAL TRAVELS IN ONE CHANNELS CAN THEN BE ACTIVATED DIRECTION ONLY Changes in membrane potentials: GRADED potentials Local changes in membrane potential that do not reach the action potential threshold Magnitude varies directly with the magnitude of the triggering event Graded potentials diminish away from the site of initiation Serve as short-distance signals Sherwood Intro to Human Physiology Chapter 4 GENERATION OF A Most synapses terminate on dendrites POSTSYNAPTIC ACTION DENDRITES POTENTIAL ePSPs/iPSPs decline with distance Loss of current due to membrane leakage In this example there is a synaptic connection between cells. POSTSYNAPTIC CELL Some synapses terminate 1. The presynaptic cell when activated on soma + + releases a neurotransmitter which opens ligand gated sodium channels Soma has large diameter, low + 2. The influx of positively charged sodium resistance ions then diffuses through the Local ePSPs/iPSPs can spread through soma postsynaptic cell: this is called an + Synapses on soma have larger effect excitatory postsynaptic potential + + than those on dendrites 3. These ions open voltage gated sodium PR + + ion channels ES Y NA + + 4. This causes an additional influx of PT sodium which initiates an action IC + + CE + potential in the post-synaptic cell LL + + + + FOR ANOTHER ACTION POTENTIAL TO BE ACTION GENERATED WE NEED AN INFLUX OF POTENTIAL POSITIVELY CHARGED IONS TO ACTIVATE VOLTAGE GATED SODIUM ION CHANNELS SUMMATION DENDRITES – In this example there are two synaptic – connections to the postsynaptic cell. S E – N AP 1. The new presynaptic cell when activated SY POSTSYNAPTIC CELL releases a neurotransmitter which opens – ligand gated chloride channels + + – 2. The influx of negatively charged chloride – ions then diffuses through the + postsynaptic cell: this is called an – inhibitory postsynaptic potential – + PR + ES – + YNA – + PT + + + IC – CE + + + LL – + + These ions cause a more negative membrane – potential and prevent voltage gated sodium + ion channels from opening – + No action potential will be initiated in the post-synaptic cell NO ACTION This phenomenon is also summation POTENTIAL IC SUMMATION PT DENDRITES Y NA ES A single ePSP is unable to PR bring postsynaptic neuron – – to action potential S E threshold – AP N Postsynaptic response is a – POSTSYNAPTIC CELL SY result of possibly + + thousands of synaptic events from many incoming neurons PR + ES YNA PT + IC CE + LL IF ALL THE PRESYNAPTIC NEURONES IN THIS ARRAY ARE FIRING ACTION + POTENTIALS + IS THE PROBABILITY OF THE INITIATION + OF A POSTSYNAPTIC ACTION POTENTIAL OCCURRING, HIGHER OR LOWER THAN + THE PREVIOUS EXAMPLE? NO ACTION ? POTENTIAL C IC DENDRITES PT TEMPORAL SUMMATION YNA ES PR Here two excitatory inputs are allowing sodium ions to enter the neurone S E AP However, if they are not active at the same POSTSYNAPTIC CELL SY N time they may not provide an influx of + enough positive ions to initiate the + + postsynaptic action potential in the trigger + zone + + + The process by which two temporally + PR separated (separated by time) inputs effect + ES the initiation of a postsynaptic action + YNA + potential is called temporal summation PT IC + CE LL + + If for example these inputs are activated at slightly different times they may not elicit an + action potential + ACTION TRIGGER ZONE: POTENTIAL USUALLY THE AXON HILLOCK TEMPORAL SUMMATION In A there is a greater time lag between sodium influx (or current) cannot deliver enough EPSPs from a single presynaptic terminal positively charged ions to the trigger zone to produce an action potential PRESYNAPTIC PRESYNAPTIC CURRENT CURRENT In B there is less of a time lag TIME TIME and enough positively charged ions are delivered to the trigger zone to produce an action EPSP EPSP potential ACTION POTENTIAL EPSPs last about 15 ms A B An AP can be triggered by a single presynaptic terminal if the interval between impulses is less than 15 ms (= high frequency activation) SPATIAL SUMMATION DENDRITES Here two excitatory inputs are allowing sodium ions to enter the neurone AP S E N This [sodium ion] provides enough positive POSTSYNAPTIC CELL SY + charge to open voltage gated sodium ion + + channels in a specific area called the trigger + zone + If only one of these excitatory inputs is + activated it may not provide an influx of enough + PR + positive ions to initiate the postsynaptic action ES + YNA potential + PT + IC The process by which two spatially separated CE + LL (separated by space) inputs effect the initiation + of a postsynaptic action potential is called + spatial summation + + If for example these input a very far apart they may not elicit an action potential ACTION POTENTIAL SPATIAL SUMMATION InA there is a greater time lag between sodium influx (or current) because the presynaptic terminals are far apart and cannot deliver EPSPs from two presynaptic terminals enough positively charged ions to the trigger zone, at the same time to PRESYNAPTIC PRESYNAPTIC produce an action potential CURRENT CURRENT InB there is less of a time lag EQUIDISTANCE FROM TRIGGER ZONE EQUIDISTANCE FROM TRIGGER ZONE because the terminals are close together and enough positively EPSP charged ions are delivered to the EPSP trigger zone to produce an action potential ACTION POTENTIAL A B In a neuron, there's often a mix of EPSPs and IPSPs occurring Summation: simultaneously. For the posts