Optical Properties of Dental Ceramics PDF
Document Details
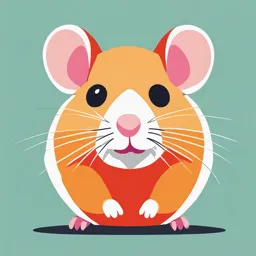
Uploaded by AstoundedAstrophysics
Reem A. Hany
Tags
Summary
This article reviews the optical properties of dental ceramics, focusing on factors such as composition, crystal structure, and porosity that affect the appearance and translucency of dental restorations. It also discusses various methods for shade matching and measuring colour. The research explores different techniques for increasing the translucency of zirconia, which is important for achieving improved aesthetics in dental procedures.
Full Transcript
Biomaterials Journal http://www.biomatj.com Online ISSN: 2812-5045 Type of the Paper (Review Article) Optical properties of dental ceramics Reem A. Hany 1,*...
Biomaterials Journal http://www.biomatj.com Online ISSN: 2812-5045 Type of the Paper (Review Article) Optical properties of dental ceramics Reem A. Hany 1,* 1 Dental Biomaterials Department, Faculty of Dentistry, Cairo University, Egypt * Corresponding author e-mail: [email protected] Abstract: Achieving successful aesthetics with a restoration is a difficult process due to the complex optical characteristics of tooth colour. The tooth reflects, absorbs, diffuses and transmits light reach- ing its surface. Thus, for acceptable aesthetic results, favourable shade matching of the all ceramic restorations should be achieved by controlling the reflection, transmission and light absorption of dental ceramic materials. Optical properties of ceramic materials are the main factors responsible for the natural appearance of the ceramic restorations. These properties are affected by several fac- tors such as: composition, crystalline content, porosity, additives, grain size and the angle on inci- dence of light. Keywords: Optical Properties; Dental Ceramics 1. Introduction Achieving successful aesthetics with a restoration is a difficult process due to the complex optical characteristics of tooth colour. The tooth reflects, absorbs, diffuses and transmits light reaching its surface. Thus, for acceptable aesthetic results, favourable shade matching of the all ceramic restorations should be achieved by controlling the re- flection, transmission and light absorption of dental ceramic materials. Optical properties of ceramic materials are the main factors responsible for the nat- ural appearance of the ceramic restorations. These properties are affected by several fac- tors such as: composition, crystalline content, porosity, additives, grain size and the angle Citation: Remm A. Hany. Opti- on incidence of light 1. cal properties of dental ceramics. Biomat. J., 1 (2),18 – 27 (2022) The optical properties of ceramic materials include 2,3: a. Wavelength dependant optical properties: https://doi.org/10.5281/znodo.582940 Colour (hue, chroma and value). 8 Translucency. Received: 10 January 2022 Opalescence. Accepted: 25 January 2022 Published: 10 February 2022 b. Bulk properties: Refractive index. Copyright: © 2022 by the authors. Colour in ceramics is one of the characteristic optical properties that occur when light Submitted for possible open access and matter interacts. When light beam strikes a polycrystalline material, this interaction publication under the terms and may result in scattering, absorption into its bulk, transmission through its mass, reflection conditions of the Creative Commons depending on the surface roughness or refraction, figure (1). All these behaviours of light Attribution (CC BY) license can be used to explain the optical properties of dental ceramics such as: transparency, (https://creativecommons.org/license refractive index and colour matching 4. s/by/4.0/). Biomat. J., 1 (2),18 – 27 (2022) Biomat. J., 1 (2),18 – 27 (2022) 19 of 10 Figure (1): Interactions between light and a surface Factors affecting the optical properties of dental ceramics: a.Translucency: - It is the ability of the material to permit the passage of light. As the amount of light passing through the material increases, the translucency increases. It is a very critical property of dental ceramics. - In all ceramic restorations, the translucency is dependent on the nature of the reinforcing crystalline phase within the core matrix, their chemical nature and size 5, 6. Alumina and conventional zirconia-based systems are opaque, while leucite reinforced are more trans- lucent. The translucency of spinel-based systems is comparable to that of lithium disilicate. Lithium disilicate ceramics are translucent even with the high crystalline content, owing to the low refractive index of lithium-disilicate crystals. Recently, translucent zirconia systems are available. The matching between the refractive index of the crystalline phase and the glassy matrix is a very important factor affecting the translucency of the porcelain. As the matching in the refractive index increases the translucency of the restoration increases. Regarding the effect of the crystalline phase, increasing the amount of crystalline phase in many high strength ceramics decrease the translucency and increase the opacity. So, these materials are used as a core in all ceramic restorations and have to be veneered with a more translucent ceramic layer 5, 6. Veneering ceramics (Layering technique): Veneering ceramics are used to veneer metals in ceramo-metallic restorations and relatively opaque core ceramics in all ceramic restorations. In ceramo-metallic restorations, the ceramic is built up in three main layers of different translucencies: core, dentin (body) and enamel (incisal) porcelains. The opaque core has very low translucency allowing it to mask the metal sub- strate, then a dentin shade and finally an enamel shade. The enamel porcelain has the highest translucency values giving the restoration a natural appearance 5. In all ceramic restorations, dental ceramics should display both translucency and opacity to mimic the dental structure. Since, there is no dental ceramic product that can offer both translucency and opacity in a single material. Therefore, manufacturers offered three basic types of porcelain powder. Opaque ceramics for coverage of dentin or discoloured abutment (they have high masking ability) and translucent glazes (dentin and enamel shades) to be used in layering technique to simulate the different natural tooth structure successfully 5, 7. b.Opalescence and counter opalescence: Opalescence is a form of scattering that happens when the crystal size is smaller than or equal to the wavelength of the light. In reflected light, the shorter blue-violet wave lengths are transmitted. While, in transmitted light, the longer red- orange wave lengths are transmitted. Opalescence is a desired property to optically mimic the natural appearance of human enamel. Human enamel has a filter that selectively passes the long waves and reflects the short waves at the same time. That’s why the incisal enamel has a bluish white colour. When the transmitted long waves hit the dentin, they are reflected giving the enamel an orange glaze. This effect is known as counter opalescence. In ceramics, the opalescence and counter opalescence effects Biomat. J., 1 (2),18 – 27 (2022) 20 of 10 are obtained through different opalescence glazes which are fine refraction oxides particles. Some have bluish colour and others have orange colour 5, 7. c.Fluorescence: Dental ceramics should exhibit fluorescent characteristics in order to create the effect of luminosity. Fluorescence is achieved in dental ceramics by adding rare earth oxides such as cerium, ytterbium, and europium oxides. When a dental ceramic restoration is not fluorescent, it tends to have a greyish appearance (reduced vitality look) especially in dark lights 5. d.Colour stability: Many factors can influence the colour stability of dental ceramics, such as; extrinsic dyes, type of ceramic material, thickness of ceramic material and the degree of porosity. The lower the degree of porosity, in the ceramic material after processing, the higher the colour stability 5. e.Shade matching: Shade matching is a very challenging step in replacing natural teeth. The shades of commercial dental porcelain pow- ders are in the yellow to yellow-red range. Since the shades of natural teeth are much greater than the yellow and yellow red range, modifiers were added to porcelain for adjustment. These modifiers are strongly pigmented porcelains sup- plied in blue, yellow, pink, orange, brown and grey.Another way of modifying or changing the appearance of a ceramic crown is the application of highly pigmented glazes. The main disadvantages of this extrinsic surface staining are; lower durability, increased solubility and the reduction in translucency 5. f.Fabrication method 5, 6, 8: The fabrication methods of dental ceramics include: 1. Sintering. 2. Heat pressing. 3. Slip casting. 4. Machining (CAD/CAM). The manufacturing technique of dental ceramics can affect the optical properties of the final restoration. In sintering, it is a process of firing the compacted ceramic powder at high temperature to ensure optimal densification, pore elimination and viscous flow when the firing temperature is reached. This densification and pore elimination in- crease the translucency of the final restoration. In heat pressed all ceramic materials, it relies on application of external pressure at high temperature. This technique promotes excellent crystal dispersion, higher crystallinity and smaller crystal size which increase the opacity of the final restoration. So, for aesthetics, two techniques are available for leucite based and lithium disilicate based heat pressed ceramics: a staining technique, or the layering technique (application of veneering ceramic). In slip-cast all ceramic materials, there are three types of ceramics available for this technique: alumina based, spinel based and zirconia toughened alumina. The main disadvantage of this technique is the highly opaque restorations (ex- cept for spinel-based restorations). The alumina crystals have high refractive index which accounts for some degree of opacity, while the spinel phase has better sintering, less porosity and thus better translucency. Factors affecting light scattering and translucency of monolithic zirconia: In order to make a dental ceramic restoration more aesthetic and translucent, the light scattering from the bulk of the material must be reduced. Internal light scattering may result from several sources such as; pores, different crystal- line phases, incomplete sintering, impurities and grain boundaries. Here is a presentation of factors affecting the light scattering and translucency in dental ceramic materials. a.Intrinsic factors: b.Extrinsic factor: 1. Composition. 1. Thickness. 2. Grain size. 2. Cement layer. 3. Sintering. 3. Light source. 4. Porosity. 4. Colour matching. Biomat. J., 1 (2),18 – 27 (2022) 21 of 10 Methods to increase the translucency of zirconia: - The conventional Yttria-stabilized tetragonal zirconia (Y-TZP) is a widely used ceramic biomaterial owing to its excel- lent mechanical properties, however, its high opacity lead to the development of a more translucent zirconia for use in fixed dental prosthesis. The translucence of zirconia is influenced by grain size, crystal isotropy, thickness, porosity, alumina content and many other factors 9. Composition 9, 10: Y-TZP is partially stabilized in the tetragonal phase at ambient temperature by metal oxides (yttria). Tetragonal crystals have high mechanical properties because of the phase transformation toughening from tetragonal to monoclinic, but they have reduced translucency. Tetragonal crystals are optically anisotropic because of the differences in the refractive index in different crystallo- graphic directions known as (birefringence). This can create discontinuity of the refractive index at the grain boundaries if the crystal orientations of the adjacent grains are not the same inducing reflection, scattering and refraction at the grain boundaries and reducing light transmittance. Therefore, a new approach to increase the translucency of zirconia is to produce fully stabilized cubic zirconia material (FSZ). This was achieved by increasing the percentage of the yttria to stabilize zirconia. Cubic grains have an isotropic orientation, which reduce light scattering and less interference with light transmission among the grains. Also, cubic grains are larger than tetragonal grains, so, they have reduced number of grain boundaries which are sources of light scattering. The content and distribution of alumina is an important factor that affect zirconia’s translucency. Alumina in incorpo- rated in zirconia-based ceramics to increase their aging stability. Unfortunately, alumina has a different refractive index than the zirconia which results in light scattering and decreased translucency 11. Another approach in increasing the translucency of zirconia was increasing the lanthanum oxide content to 0.2% mol. Grain size: Concerning the effect of grain size on the optical properties of monolithic zirconia, two concepts have been introduced. The first suggests that large grains are associated to fewer grain boundaries and increased light transmission 12. Large grains usually produced through higher temperature sintering, lead to better elimination of porosity and in- creased density, which makes the material structure more compact, thus increasing translucency 13. The second concept according to the Rayleigh scattering model, when light rays are incident on a birefringent material, the greatest scattering is recorded when grains have similar size with the visible light wavelength (400–700 nm). Con- ventional tetragonal zirconia grain size lies between 0.2 and 0.8 µm; which is greater than the wavelength range of visible light 14. Therefore, the use of nanometric tetragonal crystals should minimize the birefringence effect and improve light trans- mission, decrease scattering and increase translucency 15. Consequently, the grain size alone cannot define translucency and other parameters such as presence of cubic phase, porosity, and final density are important. Sintering: Sintering parameters such as temperature and holding time have been shown to affect the optical properties of mono- lithic zirconia. All of the studies investigating higher sintering temperatures reported an increase in translucency, cor- related to the associated increase in grain size, pore elimination and consequently increase in density. This decrease scattering and increase translucency. Most monolithic zirconia ceramics should be sintered in a sintering temperature between 1400–1550 ◦C and no higher than that, as at temperatures of 1600 or 1700 ◦C grain boundary cracks can be generated, increasing light scattering and decreasing translucency 15, 16. Porosity: Pores are the main cause of light scattering, especially when they are of a size similar to that of the wavelength of visible light (400 to 700 nm). They play a significant role in the optical properties and in particular in the translucency of zirconia ceramics. That happens because there is a difference between the refractive indexes of air (n = 1) and zirconia (n = 2.1– 2.2) 17. Biomat. J., 1 (2),18 – 27 (2022) 22 of 10 Pores can be either intra-granular or inter-granular. Inter-granular are the pores among grains with a different orienta- tion, while intra-granular pores are located inside a grain. For transparent polycrystalline materials, an extremely low porosity (˂0.01 vol.%) is required. If the pores are incorporated by growing grains during densification, they become intra-granular and impossible to remove. The inter-granular pores are more favourable to be eliminated during sintering. In the last stage of sintering, the pores are closed, and spherical and grain growth is evident. The pores are gradually decreased by diffusion to the grain boundary region 18. However, this low porosity can be achieved under sintering conditions involving high temperatures and long holding times. Pores larger than 50 nm can cause significant scattering negatively affecting light transmittance. In order to minimize pore size, the starting zirconia powder needs to be in nanometric scale. Using 40-nm instead of 90- nm powder reduces pores, improves the sintered density and reduces scattering 19. However, porosity alone cannot be considered as the main scattering generator, as other defects such as impurities or oxygen vacancies may be present in polycrystalline zirconia materials, acting as light-absorbing or scattering centres 20. Thickness: Zirconia translucency values vary inversely with its thickness. As the thickness of the restoration decreases, the trans- lucency increases. It was shown that the thickness of the restoration affects the light transmission through ceramics 21. Cement layer: The cement shade and translucency have an important effect on the final colour of the ceramic restoration. The thickness of ceramic should be at least 2 mm in order to mask the effect of the underlying discoloured tooth on the final colour of the restoration. In many clinical cases, achieving a 2-mm reduction is not possible without affecting the pulp and com- promising the strength of the remaining tooth structure. In such cases, when achieving an optimal ceramic thickness is not feasible, using cement with an appropriate thickness and colour might be the only available solution to mask the colour of the substructure and its effect on the final colour of the restoration 22. The white and yellow cements can change the final colour of ceramic so that it would be clinically acceptable. The white cement not only have a greater effect on the final colour of ceramic but also decrease the effect of the discoloured sub- structure on the final colour of the restoration. In contrary, the translucent cement will cause no clinical change in the final restoration colour compared to the colour before cementation, that’s why it is preferred in cases of non-discoloured substructure that don’t need masking 22. Light source and standard illumination 23, 24: The appearance of an object is dependent on the nature of the light under which the object is viewed because the light reflected from an object is dependent on the spectral power distribution (SPD) of the incident light. Light source is the emitter of visible electromagnetic radiation. An illuminant is a specification of a particular light source. The most commonly used CIE standard illuminant is natural daylight (D65), which has a correlated colour temperature of 6504 K. Also, incandescent lamp (A) and fluorescent lamp (F2) are used. Since each of these illuminants has a specific SPD, the colour parameters of ceramic materials under different lights may change by the illuminant. Therefore, specifying the colour of an object requires defined light source. Moreover, normal illumination geometry is shown as the beam impinging the surface at any angle between 0º and 10º. Measurement of optical properties of dental ceramics a.Measurement of color: The two primary systems used in measuring colour are the Munsell system and the CIE system (Commission Interna- tionale de l’Eclairage). The Munsell System 25, 26: It describes colour in terms of (Hue, Chroma and Value), figure (2). Hue of an object in the Munsell colour system is the distinctive colour of the object (basic colour). Chroma is the intensity of the hue. As the Chroma of a colour increases, the hue in the object increases. Value identifies the lightness or darkness of the colour. The lower values indicate dark objects. Biomat. J., 1 (2),18 – 27 (2022) 23 of 10 Figure (2): The Munsell system. The Munsell system divides Hue into 100 equal divisions around a colour horizontal circle. Chroma measured radially outward from the grey vertical axis, starting at 0 on a centre line and increasing to 10 or 18 depending on the Hue. Perpendicular to the plane formed by hue and chroma is the Value from 0 (black) to 10 (white) vertically. Neutral greys lie along the vertical axis between black and white. A point in the colour space is specified by hue, value and chroma in the form H V/C, figure (3). Figure (3): Description of the colour of a point as H V/C. The CIE System (Commission Internationale de l’Eclairage) 25, 26: The CIE system offers a specifying colour stimulus under a set of viewing conditions and evaluation of perceived colour difference. CIELAB Colour Space: The 3-dimensional colour space is defined from three axes, which are perpendicular to each another. Each colour is represented by a colour point L*, a*, b* in the colour space, figure (4). The L* (vertical axis) represents a measure of lightness, from 0 (black) to 100 (white). The a* measures redness (+a*) or greenness (-a*). The b* measures yellowness (+b*) or blueness (-b*). Figure (4): CIE L*a*b* Colour System. colour Difference: The colour difference (ΔE) between two colours is the distance between the colour points of the two colours. For exam- ple, the ΔE* ab is the colour difference of two colours that have colour coordinates L * 1, a* 1, b* 1 and L * 2, a* 2, b* 2, respectively. If two points in the L*a*b* colour space are coincident, the colour difference between them will be zero. A small ΔE* ab value indicates that the colours are close to one another. As the distance in colour space between the two points in- creases, the colour difference between them increases. The colour difference can be calculated by the following equation: Biomat. J., 1 (2),18 – 27 (2022) 24 of 10 Colour perceptibility and acceptability thresholds 25, 26: The difference in colour which is visually detectable by human observers is known as (perceptibility threshold) and the difference in colour that constitutes an unacceptable limit to dental aesthetics is known as (acceptability threshold). The perceptibility threshold ΔEab=1.2 and the acceptability threshold ΔEab=3.7 for dental ceramic restorations. Since there is difficulty in controlling light conditions in the oral cavity, and an average difference of up to (ΔE=3.7) was considered acceptable. An extended visual rating scale (EVRSAM) is used to understand the clinical significance of the numerical results of instrumental analysis evaluation of colour for dental materials, table (1) 27. ΔE Clinical significance 0 Excellent aesthetics with accurate colour choice, not being clinically perceived, or only with great difficulty. 2 Very slight difference in colour, with very good aesthetics. 4 Obvious difference, but with an average acceptable to most patients. 6 Poor aesthetics, but within the limits of acceptability. 8 Aesthetics are very poor and unacceptable to most patients. 10 Aesthetics are totally unacceptable. Table (1): extended visual rating scale b.Measurement of the translucency: Translucency is the ability of the material to permit the passage of the light. The greater the quantity of light that passes through the object, the higher the translucency of the material. Translucency can be determined from the transmitted light through ceramic material, where the light source and de- tector are on opposite sides of the sample 28. Measurement of transmitted light is done by spectrophotometer that records (L*source) with no sample in place. The sample is placed and (L*sample) is recorded. The percentage of total transmission is calculated using the following equation: T% = (L*sample/L*source) x 100 In addition, the two important measurements of translucency of ceramic materials are Contrast Ratio and Translucency Parameter. Although, there is strong correlation between them, their definitions are different with an important distinc- tion 29. Contrast ratio (CR) 25: CR is a measure of translucency that is defined as the ratio of reflectance (Y) of a given material measured with a black backing (Yb) to the measure of reflectance of the same material with a white backing (Yw), using any instrument capable of quantitatively measuring visible light intensity. CR values range from 0.0 (transparent material to 1.0 (opaque material). Luminous reflectance (Y) of the specimens recorded on black (Yb) and white (Yw) backgrounds are used to calculate the contrast ratio (CR) as follows: Translucency parameter (TP): TP is defined as the colour difference (ΔE) between a sample of uniform thickness measured with a white and black backing 25. A dental spectrophotometer is used to record the CIE coordinates (L*, a* and b*) of the same ceramic sample placed on a black (B) and a white (W) background to determine the translucency parameter (TP) by calculating the colour differ- ence according to the following equation 26: The major factors that affect TP are specimen thickness and the reflectance parameters of the black and white back grounds Biomat. J., 1 (2),18 – 27 (2022) 25 of 10 If the TP value is zero, the material is completely opaque. The greater the TP value, the higher the translucency of the ceramic. TP values of human enamel and dentin at 1mm are (18.1) and (16.4). TP values of glass ceramics at 1mm thickness range from (14.9-19.6). TP values of zirconia at 1mm thickness range from (5.5- 13.5) 25. c.Measurement of opalescence 26: Opalescence improves ceramic restoration appearance to look more natural. The values CIE coordinates (a* and b*) of the ceramic samples placed on a black (B) and a white (W) background were used to calculate the opalescence parameter (OP) according to the following equation: OP values of dental structures are between (19.8 and 22.6). OP values of monolithic ceramic restorations are between (2.5–13.3). d.Measurement of Refractive Index 30: Refractive Index Measurement or Refractometry is the method of measuring substances refractive index and assess their composition or purity. Refractometry is a technique that measures how light is refracted when it passes through a given substance and the amount of the refracted light. Snell’s Law which is also known as The Law of Refraction describes the formula behind the refraction: Refractive index should be measured at a specific wavelength. There are two methods for determining refractive index (n). Goniometric method: A goniometer-spectrometer is used to measure transmission at angle of minimum deviation of light. It has a continu- ously rotating object table with a prism. This method demands a transparent sample in form of a large triangular prism. Then, the (n) is calculated from Snell's law. Spectrophotometric methods: A spectrophotometer, with a sampling accessory, can be used to rotate the sample and the detector to angles that allow absolute specular reflectance data to be collected. e.Shade matching and measuring tools of colour: Manual (visual) shade matching 31: Most commonly, the colour matching of teeth to ceramic materials is done manually and visually with dental shade guides as it is much cheaper to obtain. However, the colour selection done in this method is subjective and influenced by the ability of the human observer to choose the best matching shade. Many factors affect the accuracy of shade matching such as; observer colour perception, ambient lighting, eye fatigue, age, stress, prior exposure of eyes to light, Biomat. J., 1 (2),18 – 27 (2022) 26 of 10 the effect of the surrounding environment, metamerism and the acceptance threshold of mismatch. To avoid these fac- tors, colorimetric instrumentation can be used to choose standardizing close colour match. Automatic “Instrumental” shade matching: These instruments were introduced to overcome the limitations of the visual tools. These instruments can be classified as spectrophotometers, colorimeters and digital imaging devices. 1.Spectrophotometer 31, 32: Spectrophotometers measure and record the amount of light energy reflected or transmitted from an object’s surface along the visual light spectrum (380-780 nm). This device has high precision, sensitivity to measure absolute colours and is equipped with spectral distributions of various illuminates. Spectrophotometer can be used to: 1. Measure the colour of ceramic materials: - Measure the spectral reflectance of a colour and converts it into a tri-stimulus value (CIE L*a*b*). 2. Measure the translucency of ceramic materials: - Measure percentage of transmittance (T%). - Measure the illuminance (Y) over standard white and black background. 3. Measure the absolute transmittance and absolute reflectance. 4. Convert readings taken into other calculations of optical properties, such as refractive index. 2.Colorimeters 31: Measure quantitatively visible light intensity and difference in colour intensity, which is related to colour absorption. Analyse the values for red, green and blue reflected through filters that simulate sensing photoreceptor cells of the human eye. The tristimulus X, Y and Z coordinates are automatically converted to L*, a*, b* values of the CIE L*a*b* system. However, they are less accurate than spectrophotometers as colorimeters do not register total visual light spec- tral reflectance. 3.Digital cameras 31: Are based on the RGB colour model in which the camera obtains red, green and blue data that is used to produce the colour image and generate a broad arrangement of colours. Edge loss effects 31: One issue associated with spectrophotometers and colorimeters is phenomena of edge loss effects. These instruments measure colour with a small window (measuring area) for illumination and measurement, resulting in loss of light at the edges of the illuminated area during reflectance measurements of translucent materials. The edge loss effect occurs in translucent object when light is transmitted and scattered through the edge of an object and not reflected back to the instrument to be measured creating a blurring effect at the edges. This can affect the accu- racy of measurements by these instruments. References 1. Porojan L, Vasiliu RD, Bîrdeanu MI, Porojan SD. Surface characterization and optical properties of reinforced dental glass- ceramics related to artificial aging. Molecules. 2020;25(15). 2. Manziuc M-M, Gasparik C, Negucioiu M, et al. Optical properties of translucent zirconia: A review of the literature. Euro- Biotech J. 2019;3(1):45-51. 3. Brow. R.K. Chapter 10 : Optical Properties. Cer103 Notes. Published online 1800:10-19. 4. Hojo J. Materials Chemistry of Ceramics. Springer Nature Singapore Pte Ltd; 2019. 5. Sakaguchi RL and Powers JM: Craig’s Restorative Dental Materials, Thirteen edition, Mosby, 2012; 254-264. 6. Callister WD:Materials Science and Engineering An Introduction, Sixth edition, John Wiley and Sons, 2003; 384. 7. Ilie N and Hickel R. Correlation between ceramics translucency and polymerization efficiency through ceramics. Dent Ma- ter 2008;24:908-14. 8. Denry I and Holloway JA. Ceramic For Dental Applications: A Review. Materials 2010, 3, 351-368. Biomat. J., 1 (2),18 – 27 (2022) 27 of 10 9. Harada K, Raigrodski AJ, Chung KH, et al. A comparative evaluation of the translucency of zirconias and lithium disilicate for monolithic restorations. J Prosthet Dent 2016; 116(2):257-63. 10. Zhang Y. Making yttria-stabilized tetragonal zirconia translucent. Dent Maker 2014; 30(10):195-203. 11. Ritter RG. Use of high translucency zirconia in the aesthetic zone. Dent Today. 2013;32. doi:10.1155/2014/432714. 12. Stawarczyk, B.; Özcan, M.; Hallmann, L.; Ender, A.; Mehl, A.; Hämmerlet, C.H.F. The effect of zirconia sintering tempera- ture on flexural strength, grain size, and contrast ratio. Clin. Oral Investig. 2013, 17, 269–274. 13. Tuncel I.; Turp, I.; ܸsümez, A. Evaluation of translucency of monolithic zirconia and framework zirconia materials. J. Adv. Prosthodont. 2016, 8, 181–186. 14. Ban, S. Reliability and properties of core materials for all-ceramic dental restorations. Jpn. Dent. Sci. Rev. 2008, 44, 3–21. 15. Carrabba, M.; Keeling, A.J.; Aziz, A.; Vichi, A.; Fonzar, F.R.; Wood, D.; Ferrari, M. Translucent zirconia in the ceramic scenario for monolithic restorations: A flexural strength and translucency comparison test. J. Dent. 2017, 60, 70–76. 16. Gómez, S.; Suárez, G.; Rendtorff, N.M.; Aglietti, E.F. Relation between mechanical and textural properties of dense materi- als of tetragonal and cubic zirconia. Sci. Sinter. 2016, 48, 119–130. 17. Sabet, H.; Wahsh, M.; Sherif, A.; Salah, T. Effect of different immersion times and sintering temperatures on translucency of monolithic nanocrystalline zirconia. Futur. Dent. J. 2018, 4, 84–89. 18. Vagkopoulou, T.; Koutayas, S.O.; Koidis, P.; Strub, J.R. Zirconia in dentistry: Part 1. Discovering the nature of an upcoming bioceramic. Eur. J. Esthet. Dent. 2009, 4, 130–151. 19. Anselmi-Tamburini, U.; Woolman, J.N.; Munir, Z.A. Transparent nanometric cubic and tetragonal zirconia obtained by high-pressure pulsed electric current sintering. Adv. Funct. Mater. 2007, 17, 3267–3273. 20. Kontonasaki E, Rigos AE, Ilia C, Istantsos T. Monolithic zirconia: an update to current knowledge. optical properties, wear, and clinical performance. Dentistry journal. 2019 Sep;7(3):90. 21. Yu B, Ahn JS, Lee YK, Measurement of translucency of tooth enamel and dentin. Acta Odontal Scand 2009;67:57-64. 22. Cekic-Nagas I, Canay S, Sahin E. Bonding of resin core materials to lithium disilicate ceramics: the effect of resin cement film thickness. Int J Prosthodont. 2010. Sep-Oct;23(5):469–71. 23. Lee B uy M& Y. Color difference of all-ceramic materials by the change of illuminants. Am J Dent. 2018;22(2). 24. Clarke PJ. Surface Colour Measurement. Nigel Fox, Quality of Life Division; 2006. 25. Hill C. Optical Properties Of A Novel Glass Ceramic Restorative Material. J Chem Inf Model. 2020;53(9). 26. Della Bona A, Nogueira AD, Pecho OE. Optical properties of CAD-CAM ceramic systems. J Dent. 2014;42(9):1202-1209. 27. Cláudia Ângela Maziero Volpato MCF, Petter, Analúcia Gebler Philippi CO. Ceramic Materials and Color in Dentistry.; 2004 28. Bergmann CP. Dental Ceramics; Microstructure, Properties and Degradation. Springer Heidelberg New York Dordrecht London; 2013. 29. Barizon KTL, Bergeron C, Vargas MA, et al. Ceramic materials for porcelain veneers. Part I: Correlation between translu- cency parameters and contrast ratio. J Prosthet Dent. 2013;110(5):397-401. 30. Kozlova NS, Kozlova AP, Zabelina A V. Spectrophotometric Methods of Refractive Indices Measurement Measuring the refractive index of single crystal. Agil Technol Aust. 2019:1-5. 31. Ragain JC. A Review of Color Science in Dentistry: Shade Matching in the Contemporary Dental Practice. J Dent Oral Disord Ther. 2016;4(2):01-05. 32. Shono NN, Al HNA. Contrast Ratio and Masking Ability of Three Ceramic Veneering Materials. 2012:406-416.