Intensive Care Monitoring PDF
Document Details
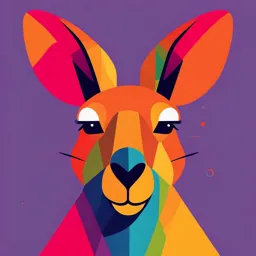
Uploaded by AltruisticInspiration260
Kenneth Waxman, MD, Frederic S. Bongard, MD, Darryl Y. Sue, MD
Tags
Summary
This document discusses physiologic monitoring in intensive care units (ICUs). It details electrocardiography, including lead placement, arrhythmia detection, and myocardial ischemia. The document also covers blood pressure monitoring, including the relationship between blood pressure and cardiac function, peripheral circulation and calculations for systemic vascular resistance.
Full Transcript
187 00 8 Intensive Care Monitoring Kenneth Waxman,...
187 00 8 Intensive Care Monitoring Kenneth Waxman, MD Frederic S. Bongard, MD Darryl Y. Sue, MD Physiologic monitoring is available for appropriate indica- of extraneous noise. A “modified lead II” configuration is tions in the ICU. Monitoring should be selected and applied appropriate for routine monitoring, with limb leads to detect pathophysiologic abnormalities in patients at high extended proximally to lie over the shoulders. Placing them risk of developing them and to aid in the titration of therapy over bony prominences reduces electrical noise from muscle to appropriate physiologic end points. contractions. Most electrocardiographic amplifiers and display modules can be used for both diagnostic and monitoring applications. Electrocardiography The diagnostic setting permits greater amplifier bandwidth Continuous electrocardiography permits monitoring of heart (0.05–100 Hz) when compared with the monitor setting rate, detection of arrhythmias, and evaluation of pacemaker (0.5–50 Hz). For routine rate and arrhythmia detection, the function. It also may help detect myocardial ischemia or elec- monitor setting is preferred because it decreases baseline trolyte abnormalities. Continuous electrocardiographic mon- wander, reduces unwanted interference, and improves overall itoring is indicated for patients with potential for developing trace quality. However, because it may falsely elevate or arrhythmias—particularly those with acute myocardial depress ST segments, the diagnostic mode should be selected infarction, traumatic cardiac contusion, following cardiac when myocardial ischemia is the primary concern. surgical procedures, and those with a prior history of arrhyth- mia. It is also useful for those in whom heart rate monitoring Clinical Applications is indicated, such as patients at risk of hemorrhage or those undergoing fluid resuscitation. Monitoring of the ST seg- A. Electrocardiographic Monitoring—Lead placement at ments is indicated for patients at risk of myocardial ischemia, the shoulders and in the lead II position parallels the atria such as those with coronary artery disease who have an injury, and results in the greatest P-wave voltage of any surface lead illness, or operation. Monitoring of the ECG also may be use- configuration. This facilitates recognition of arrhythmias ful to detect certain electrolyte abnormalities such as and inferior wall ischemia. When placed in the V5 position hypokalemia during treatment of diabetic ketoacidosis. along the anterior axillary line, both anterior and lateral wall The cardiac electrical potential available for skin surface ischemia can be detected. Because patient positioning may monitoring is between 0.5 and 2.0 mV. Because of this low make a true lead V5 position difficult, a modified arrange- signal level, electrocardiographic systems must have good ment (CS5), in which the left arm lead is placed just lateral to sensing, amplifying, and display capabilities. The electrodes the left nipple and the lower limb lead is placed over the iliac used for electrocardiographic monitoring are usually com- crest, is a good alternative. When possible, leads II and V5 posed of silver–silver chloride gel (Ag/AgCl) inside an adhe- should be monitored simultaneously. Esophageal leads are sive pad. Prior to placement, the skin should be clean and better than lead II for the detection of arrhythmias, but their dry. The stratum granulosum has an electrical resistance of use is difficult in patients who are not paralyzed and sedated, 50,000 Ω/cm3, which can be reduced to 10,000 Ω/cm3 simply and they are rarely used in the ICU setting. by cleansing, which removes oils and dead cells. Difficulties with a low signal are often remedied by reapplying the elec- B. Complications—Difficulties associated with electrocardio- trode after cleaning the skin. graphy are usually due to technical error or equipment mal- Optimal electrode placement (Figure 8–1) allows proper function. Electrodes may not function properly when they are detection of the electrocardiographic signals with a minimum old and dry or if they are not attached securely. Electrical noise Copyright © 2008 by The McGraw-Hill Companies, Inc. Click here for terms of use. 188 CHAPTER 8 Midclavicular line Anterior axillary line Midaxillary line Horizontal plane of V4-6 V1 V2 V3V4 V5 V6 V7 V8 V9 V9R V8R V7R (V2R)(V1R) V6R V5R V4R V3R 1 2 3 6 4 5 Figure 8–1. Locations of the unipolar precordial leads on the body surface. (Reproduced, with permission, from Goldschlager N, Goldman MJ: Principles of Clinical Electrocardiography, 13th ed. Originally published by Appleton & Lange. Copyright © 1989 by The McGraw-Hill Companies, Inc.) accompanying the displayed electrocardiogram is usually due Goodman S, Shirov T, Weissman C: Supraventricular arrhythmias to loose electrodes, broken wires, or poorly fitting contacts or in intensive care unit patients: Short and long-term conse- problems with associated electrical equipment. Proximity of quences. Anesth Analg 2007;104:880–6. [PMID: 17377100] the patient to electrical lines (ie, power cords, etc.) produces a Reinelt P et al: Incidence and type of cardiac arrhythmias in criti- cally ill patients: A single-center experience in a medical- potential difference through capacitive coupling known as cardiological ICU. Intensive Care Med 2001;27:1466–73. common-mode voltage. Typically as low as a few millivolts, cou- [PMID: 11685339] pling can cause voltages as high as 20 V. Common-mode volt- age interference usually presents as 60-Hz interference and often can be reduced by using properly placed shielded wires, Blood Pressure Monitoring good skin preparation, and an electrocardiographic amplifier that offers common-mode rejection. Because systemic blood pressure is related to both cardiac Proper sensitivity setting of the amplifier and recorder function and the peripheral circulation, blood pressure mon- is essential to make certain that large T waves are not itoring provides information related to the overall circula- “double-counted” in the rate determination. Additional fil- tory condition. While blood pressure monitoring is standard tering is occasionally required for patients who have and universal for critically ill patients, the type of blood pres- pacemakers—in whom the pacer spike is interpreted as a sure monitoring and its frequency should be chosen based QRS complex. on the individual patient’s diagnosis and condition. INTENSIVE CARE MONITORING 189 Blood pressure represents lateral force exerted on the vas- The velocity of blood flow is slowest in the largest arter- culature by flowing blood. Pressure is maximal shortly after ies because they are distensible and absorb energy from the ventricular systole (SBP). The diastolic pressure (DBP) fol- pressure wave front. The pulse wave travels at a rate of 7–10 m/s lows cardiac diastole and is the lowest pressure in the cycle. in large arteries such as the subclavian artery and increases to The mean arterial pressure (MAP) represents the standing 15–30 m/s in smaller distal arteries. pressure in the arterial circuit and is calculated as follows: When a pressure wave front enters a small, nondistensible artery, part of the wave may be reflected back proximally. If a (SBP + 2 × DBP) reflected wave strikes an oncoming wave, the two summate, MAP = causing a higher pressure than would occur otherwise. This 3 phenomenon produces pressures in the distal peripheral arteries that paradoxically may be more than 20–30 mm Hg Pulse pressure is the arithmetic difference between the sys- above those recorded in the aorta. tolic and diastolic pressures. Pulse pressures vary with stroke Arterial pressure depends on cardiac output (CO) and volume or vascular compliance. Pulse pressures less than systemic vascular resistance (SVR). The latter is calculated as 30 mm Hg are common with hypovolemia, tachycardia, aor- follows: tic stenosis, constrictive pericarditis, pleural effusions, and ascites. Widened pulse pressures may be due to aortic regur- (MAP − CVP) gitation, thyrotoxicosis, patent ductus arteriosus, arteriove- SVR = × 80 nous fistula, and coarctation of the aorta. Variability of pulse CO pressure and systolic pressure during the respiratory cycle has been correlated with response to intravascular fluid repletion. When MAP and CVP (central venous pressure) are in The initial upstroke and peak of the arterial waveform are millimeters of mercury and CO is in liters per minute, SVR produced by left ventricular ejection. The end of systole is is expressed in dynes × seconds × cm–5. Evaluation of the marked by a brief decline in pressure until the aortic valve equation indicates that an increase in either SVR or CO will closes and redirects backflowing blood into the aorta. The increase mean arterial pressure. “dicrotic notch” so created may be detected on recordings obtained from aortic or proximal arterial sites. The waveform Clinical Applications becomes more peaked and of higher amplitude as it progresses distally. The initial upstroke is prolonged, producing a higher Arterial blood pressure can be assessed either by direct systolic and a lower diastolic pressure (Figure 8–2). instrumentation of the vascular tree or by indirect means. The indirect technique usually involves inflating a cuff to occlude an artery. As the cuff is deflated and inflow resumes, arterial pressure can be determined. A. Noninvasive Arterial Pressure Monitoring— Central 1. Palpation—A blood pressure cuff is placed above an eas- Aortic root ily palpated artery and inflated until pulsation ceases. On Subclavian artery cuff deflation, systolic pressure is estimated as that pressure at which pulsation resumes. This method is limited because Axillary artery it underestimates true arterial pressure and does not provide a diastolic pressure. Brachial artery 2. Auscultation (Riva-Rocci method)—When an occlud- Radial artery ing proximal cuff is deflated below systolic pressure, flow begins through the compressed artery. The turbulent flow thus Femoral artery created strikes the walls of the vessel, causing them to reverber- Dorsalis pedis artery ate (Korotkoff sounds). As long as the cuff pressure is higher than diastolic pressure, no flow will occur during diastole. The Peripheral sound thus produced is rhythmic in nature. Once the pressure Figure 8–2. The shape of the arterial pressure wave in the cuff is below diastolic pressure, flow occurs throughout front changes as it progresses distally. The systolic peak the cardiac cycle, and the sounds disappear. A cuff 20% wider becomes more pronounced, as does the dicrotic notch. than the diameter of the limb must be used to obtain correct (Reproduced, with permission, from Morgan GE, Mikhail sphygmomanometric pressures. If the cuff is too narrow, the MS: Clinical Anesthesiology. Originally published by systolic and diastolic pressures will be artificially increased, Appleton & Lange. Copyright © 1992 by The McGraw-Hill and vice versa. Other sources of error include too-tight or too- Companies, Inc.) loose cuff application and too-rapid or too-slow cuff deflation. 190 CHAPTER 8 Unduly slow deflation produces venous congestion, which where Δf is the frequency shift, fe is the frequency of the decreases the amplitude of the Korotkoff sounds as the cuff insonating beam, V is blood velocity, θ is the incident angle pressure nears the diastolic pressure. of insonation, and C is the velocity of sound in tissue. When compared with intraarterial pressure measure- The depth of tissue penetration by the sound beam is ments, those obtained by auscultation differ by 1–8 mm Hg inversely proportional to the frequency of insonation. systolic and 8–10 mm Hg diastolic. At intraarterial pressures Because arteries of interest are typically superficial, a 10- below 120 mm Hg systolic, auscultation tends to overesti- MHz probe can be used. As can be seen from the equation, mate pressure, whereas above 120 mm Hg, auscultation the largest frequency shift is obtained when the probe is held underestimates arterial pressure. parallel to the artery. Perpendicular positioning decreases the frequency shift (cos θ → 0). Doppler blood pressure meas- 3. Oscillometry—The oscillometer uses two cuffs in series; urements are obtained by placing an ultrasonic probe on an one occludes the artery proximally, whereas the other detects artery distal to a compressing cuff. the onset of pulsations. Slow deflation of the proximal cuff Doppler sounds become apparent when cuff pressure falls produces aneroid needle oscillation or mercury column vari- below arterial pressure. Arterial pressures obtained using a ation at systolic pressure. Oscillometry is the only noninva- Doppler probe usually are higher than those obtained by pal- sive technique capable of indicating mean arterial pressure, pation and lower than those obtained by direct measurement, which coincides with maximum deflection of the manome- although the overall correlation is excellent. An automated ter. Although diastolic pressure is defined as that point at device (Arteriosonde) is available for Doppler measurements. which oscillation ceases, measurement of diastolic pressure is It uses a 2-MHz insonation frequency directed at the brachial in fact inaccurate. Oscillometry requires several cardiac artery. Overall accuracy is very good—especially at low pres- cycles to measure blood pressure accurately. sures, when ultrasonic and palpatory techniques are more Automated oscillometry devices generally use single- accurate than auscultation. Disadvantages include motion bladder cuffs that are alternately inflated and deflated. On sensitivity, requirement for accurate placement, and the need deflation, alterations in cuff pressure are sensed by a trans- to use a sonic transmission gel. ducer inside the instrument. Pairs of oscillations and corre- sponding cuff pressures are stored electronically to permit B. Invasive Pressure Monitoring—Insertion of a catheter measurement of the systolic and diastolic pressures. Use of into an artery is the most accurate technique for pressure these automated devices is limited in those with irregular monitoring. Such catheters are connected by tubing to pres- rhythms or when motion cannot be minimized. In addition, sure transducers that convert pressure into electrical signals. measurements tend to be unreliable in low-flow states. Because arterial pressure waves are themselves too weak to generate electrical impulses, most transducers actually meas- 4. Plethysmography—Arterial pulsations produce minute ure the displacement of an internal diaphragm. This changes in the volume of an extremity. Such alterations in diaphragm is connected to a resistance bridge such that finger volume can be detected photometrically with a motion of the diaphragm modulates an applied current. The plethysmograph. These devices tend to be less accurate than transducer’s sensitivity is the change in applied current for a alternative pressure monitoring techniques, particularly dur- given pressure change. ing low-flow and stress conditions. Because transducers are ultimately mechanical, they 5. Doppler—The Doppler principle states that any moving absorb energy from the systems they monitor. If absorbed object in the path of a sound beam will alter the frequency of the energy in the transducer’s diaphragm is suddenly released, it transmitted signal. The sound beam used to “insonate” tissue is will begin to vibrate at its natural (resonant) frequency. The created by applying an electrical potential to a crystal that causes tendency for this oscillation to stop depends on the damping it to oscillate in the radiofrequency spectrum. This sound is cou- of the system. Oscillating frequency increases as damping pled to the tissue of interest through an acoustic gel. decreases. The resonant frequency is a function of the natu- When the beam strikes moving blood cells, the frequency ral frequency and the damping coefficient. Classically, a sys- of the reflected beam is altered in a manner proportionate to tem’s damping coefficient is determined by applying and the velocity of the reflecting surface. Continuous- and pulsed- releasing a square pressure wave (Figure 8–3). wave Doppler equipment is currently available. Continuous- Damping increases when compliance increases. Soft wave transducers have two crystals mounted together in a (compliant) connecting tubing absorbs transmitted pressure single probe. One is continuously transmitting, and the other waves and damps the system. Other factors that increase is continuously receiving. Only the velocity of flow and its damping include air in the transducer dome or tubing, exces- direction can be determined by a continuous-wave device. sively long or coiled tubing, connectors containing Because a Doppler shift occurs only when blood moves rela- diaphragms, and the use of stopcocks. Because air is more tive to the transducer, an angle correction must be applied: compressible than water, even small bubbles increase the sys- tem damping. Excessive damping results in underestimation of systolic pressure and overestimation of diastolic pressure. 2feV(cos θ ) There is little effect on mean pressure. Underdamped systems Δf = C produce the opposite effects. Additionally, systems with INTENSIVE CARE MONITORING 191 Figure 8–3. The amplitude ratio obtained by measuring the amplitude of oscillations after pressure release. Either the listed formula or the tables then can be used to calculate the damping coefficient. (Reproduced, with permission, from Morgan GE, Mikhail MS: Clinical Anesthesiology. Originally published by Appleton & Lange. Copyright © 1992 by The McGraw-Hill Companies, Inc.) 192 CHAPTER 8 insufficient compliance tend to “ring” when rapid pressure insufficiency may result from occlusion of the dominant changes cause oscillations within the system. Conversely, artery, all patients should undergo an Allen test prior to overdamping decreases the frequency response to the point catheter insertion, and the results should be entered into the that rapid changes in pressure may not occur. The effect of medical record. However, one prospective study has demon- damping on the natural frequency of the system is illustrated strated that vascular complications were not reliably related in Figure 8–4. The optimal damping coefficient is near 0.7 to results of the Allen test. Overall, there is a 10% incidence because there is essentially no effect on amplitude until the of arterial occlusion in adults cannulated with 20-gauge measured frequency approaches the natural frequency of the Teflon catheters for a period of 1–3 days. The use of 22-gauge measuring system. catheters seems to reduce this incidence. For unknown reasons, women have a lower incidence of arterial thrombosis than men. When thromboses do occur in Clinical Applications women, occlusions are usually temporary. Distal occlusion of The arteries commonly used for invasive blood pressure the radial artery may cause overestimation of systolic pres- monitoring, in order of usual preference, are the radial, sure because of increased wave reflection, whereas proximal ulnar, dorsalis pedis, posterior tibial, femoral, and axillary occlusion usually causes reduction in pressure owing to arteries. The radial artery is preferred because of its ease of overdamping. Another complication of arterial catheters is cannulation and relatively low incidence of serious compli- infection, most commonly limited to the skin but some- cations. The ulnar artery is the dominant artery to the hand times involving the artery as well; distal septic emboli rarely in 90% of patients. It connects with the radial artery through occur. The incidence and severity of such infections can be the palmar arches in 95% of patients. Because vascular minimized by strict adherence to policies of daily catheter Figure 8–4. The amplitude ratio depends on the natural frequency of the system and the damping ratio (h). A sys- tem that operates below its natural frequency and with a damping ratio near 0.7 is desirable. (Reproduced, with permission, from Fry DL: Physiologic recording by modern instruments with particular reference to pressure recording. Physiol Rev 1960;40.) INTENSIVE CARE MONITORING 193 Figure 8–5. The height of the transducer must be adjusted to the phlebostatic axis to ensure accuracy of the pressure measurements. inspection, sterile dressing changes, and limiting catheteriza- by 1.36. Therefore, if the system is calibrated accurately, the tion to 5 days or less at any single site. Pseudoaneurysm for- pressure reading should be about 14.8 mm Hg when the mation may be a late complication of arterial catheters. The stopcock is raised 20 cm above the transducer. incidence of pseudoaneurysms may be minimized by using An additional use of arterial catheterization is to provide smaller catheters, minimizing the duration of catheteriza- access for arterial blood sampling. This is often indicated in tion, and preventing catheter infections. patients who require frequent sampling of blood for arterial Physiologic pressures are measured with reference to the blood gases or other blood tests. tricuspid valve, where intravascular pressure is defined as zero. This phlebostatic axis is independent of changes in Araghi A, Bander JJ, Guzman JA: Arterial blood pressure monitor- body habitus. Postural changes affect the reference pressure ing in overweight critically ill patients: Invasive or noninvasive? by less than 1 mm Hg. The phlebostatic point is identified as Crit Care 2006;10:R64. [PMID: 16630359] (1) 61% of the way from the back to the front, (2) exactly in Bur A et al: Factors influencing the accuracy of oscillometric blood pressure measurement in critically ill patients. Crit Care Med the midline, and (3) one-quarter of the distance above the 2003;31:793–9. [PMID: 12626986] inferior tip of the xiphoid process. A convenient method of Gibbs NC, Gardner RM: Dynamics of invasive pressure monitor- system calibration is to move an open stopcock attached by ing systems: Clinical and laboratory evaluation. Heart Lung fluid-filled connecting tubing to the transducer up against 1988;17:43–51. [PMID: 3338943] the patient’s midaxillary line. The digital display on the mon- Gunn SR, Pinsky MR: Implications of arterial pressure variation in itor indicates whether the midaxillary line is above (positive patients in the intensive care unit. Curr Opin Crit Care pressure) or below (negative pressure) the transducer. The 2001;7:212–7. [PMID: 11436530] bed is then moved up or down until the pressure reads zero Mignini MA, Piacentini E, Dubin A: Peripheral arterial blood pres- (Figure 8–5). sure monitoring adequately tracks central arterial blood pres- Calibration of the monitor for nonzero pressure can be sure in critically ill patients: An observational study. Crit Care 2006;10:R43. [PMID: 16542489] done internally or externally. External calibration can be done with a mercury manometer for systemic arterial pres- Pittman JA, Ping JS, Mark JB: Arterial and central venous pressure monitoring. Int Anesthesiol Clin 2004;42:13–30. [PMID: sures. A convenient method adequate for the lower pressure 14716195] range required for a pulmonary artery catheter (up to 60 cm H2O) takes advantage of the fluid-filled connecting tubing. Central Venous Catheters After establishing the zero reference, move an open stopcock connected to the transducer above the transducer a meas- Central venous (CV) catheters are inserted via the subcla- ured amount. The height above the transducer in centimeters vian, internal jugular, or a peripheral vein in the arm. (cm H2O pressure) should be read by the monitor system in Femoral venous catheters are not long enough to reach millimeters of mercury as height in centimeters divided “central” veins but provide similar access for intravenous 194 CHAPTER 8 infusions. For monitoring purposes, CV catheters provide contraction. The x descent corresponds to the period of ven- estimates of central venous pressure (CVP) and measure- tricular ejection, when blood empties from the heart; it is ment of central venous oxygen saturation (ScvO2). Central inscribed when the ventricle draws down on the floor of the venous pressure (CVP) reflects the balance between systemic atrium and decreases the CVP. The v wave is produced by the venous return and cardiac output. In the normal heart, the increase in atrial pressure that takes place as venous return right ventricle is more compliant than the left. This differ- continues while the tricuspid valve is closed. The y descent ence in compliance accounts for the slope of their correspon- occurs when the tricuspid valve opens at the conclusion of ding Frank-Starling curves. The use of CVP to assess ventricular contraction, and blood enters the right ventricle. left-sided preload causes difficulty because CVP primarily The a wave is absent during atrial fibrillation and is magni- reflects changes in right ventricular end-diastolic pressure fied by tricuspid stenosis (cannon wave). The x descent also and only secondarily reflects changes in pulmonary venous may be absent with atrial fibrillation. The x and y descents and left-sided pressures. The relationship between CVP and are both exaggerated by constrictive pericarditis. Cardiac venous return is shown in Figure 8–6A. Decreasing right tamponade magnifies the x descent while abolishing the y atrial pressure below zero does not significantly increase descent. When tricuspid regurgitation occurs, the c wave and CVP because of collapse of the vasculature leading to the the x descent are replaced by a large single regurgitant wave. chest. The figure also demonstrates that changes in mean sys- Pulmonary hypertension decreases right ventricular compli- temic pressure cause a parallel change in venous return. ance and accentuates the v wave. Alterations in vascular resistance (decreased by anemia, arte- riovenous fistulas, pregnancy, or thyrotoxicosis) change the slope of the respective curves (Figure 8–6B). Clinical Applications A water manometer may be used to measure CVP. The A. CVP Monitoring—CVP monitoring is best used for normal range of CVP is between –4 and +10 mm Hg (–5.4 patients without preexisting cardiac disease as one indicator and +13.6 cm H2O). of the adequacy of venous return and cardiac filling. An An electronic transducer also displays the pressure wave- intravenous fluid challenge is employed to aid in determin- form. The bandwidth of a catheter-transducer system used to ing whether decreased blood pressure is due to hypovolemia monitor CVP can be significantly narrower than that used or to cardiogenic failure. Measurements of CVP are affected for arterial pressure. by ventilation because transthoracic pressure is transmitted A typical CVP waveform has three positive deflections through the pericardium and the thin-walled venae cavae. (a, c, and v) and two descents (x and y) (Figure 8–7). The During spontaneous ventilation, inspiration lowers CVP, and increase in venous pressure caused by atrial contraction pro- exhalation increases it. The situation is reversed in patients duces the a wave. The c wave is created when the tricuspid valve being mechanically ventilated, in whom inspiration increases is displaced into the right atrium during initial ventricular intrathoracic pressure and elevates CVP. Venous return (L/min) Venous return (L/min) Figure 8–6. A. Effect of mean systemic pressure on venous return. B. Effect of systemic vascular resistance on venous return. (Reproduced, with permission, from Otto CW: Central venous pressure monitoring. In: Blitt CD (ed), Monitoring in Anesthesia and Critical Care. New York: Churchill Livingstone, 1985. Copyright 1985 Elsevier.) INTENSIVE CARE MONITORING 195 c pressure (mm Hg) Water Inspiration Inspiration 20 a v manometer Right atrial pressure 10 0 Mean end expiratory pressure x y Exhalation Exhalation Figure 8–7. Effect of mechanical ventilation on central venous pressure. The a, c, and v waves along with the x and y descents are shown. (Reproduced, with permission, from Otto CW: Central venous pressure monitoring. In: Blitt CD (ed), Monitoring in Anesthesia and Critical Care. New York: Churchill Livingstone, 1985. Copyright 1985 Elsevier.) The degree of this elevation depends on the compliance ventricle approaches 100%. Such perforations may occur of the lungs and intravascular volume and will vary among either from guidewires or from catheter erosion—again, par- patients. For this reason, CVP measurements are best made ticularly with introducer catheters. Other structures that may and compared at end expiration. When positive end- be injured on insertion include the brachial plexus, the stel- expiratory pressure (PEEP) is applied, the positive pressure is late ganglion, and the phrenic nerve. Air emboli are uncom- transmitted through to the right atrium, causing a decrease mon at insertion but more often during use or at the time of in venous return and a rise in CVP. Again, the magnitude of removal when the patient is not positioned properly. Late this effect of PEEP on CVP varies with pulmonary compli- complications are due to catheter migration, embolization, ance and blood volume. Some argue that the patient should and infection. The incidence of cannula-related thrombosis be temporarily removed from PEEP while the measurement of the axillary and subclavian veins varies between 16.5% and is taken. This is both impractical and potentially dangerous. 46%. Central venous catheter infections occur in approxi- In critical situations, an esophageal probe can be inserted to mately 5% of insertions. The organisms most commonly estimate transthoracic pressure. Subtracting the transtho- involved are Staphylococcus epidermidis, 30%; Staphylococcus racic pressure from the CVP provides transmural pressure, aureus, 8%; streptococci, 3%; gram-negative rods, 18%; diph- which is a better estimate of right atrial pressure in the pres- theroids, 2%; Candida species, 24%; and other pathogens, 15%. ence of elevated transthoracic pressure. Both colonization of central venous catheters and systemic sepsis are reduced by routine catheter care and periodic B. Central Venous O2 Saturation—Mixed venous oxygen removal and reinsertion. saturation (S–vO2) reflects the relative delivery of O2 to the tis- sues compared with consumption. If lower than normal, concern should be raised about tissue hypoxia. True S–vO2 Kalfon P et al: Comparison of silver-impregnated with standard must be measured in the pulmonary artery. Central venous multi-lumen central venous catheters in critically ill patients. Crit Care Med 2007;35:1032–9. [PMID: 17334256] O2 saturation (ScvO2) does not require a pulmonary artery catheter, but theoretically, the value will differ from S–vO2 Kusminsky RE: Complications of central venous catheterization. J Am Coll Surg 2007;204:681–96. [PMID: 17382229] because ScvO2 obtained from a subclavian or internal jugular Marx G, Reinhart K: Venous oximetry. Curr Opin Crit Care vein does not reflect venous blood returning via the inferior 2006;12:263–8. [PMID: 16672787] vena cava or coronary sinus. Generally, ScvO2 is about 5% Michard F, Teboul JL: Predicting fluid responsiveness in ICU patients: higher than S–vO2. A critical analysis of the evidence. Chest 2002;121:2000–8. [PMID: In practice, however, ScvO2 appears to have similar predic- 12065368] tive value for end-organ hypoxia as S–vO2. Recent studies Onders RP, Shenk RR, Stellato TA: Long-term central venous emphasizing early goal-directed therapy in sepsis have catheters: Size and location do matter. Am J Surg 2006; emphasized a target ScvO2 of greater than 70% by giving 191:396–9. [PMID: 16490554] blood transfusion and cardiac inotropic drugs. ScvO2 can be Pinsky MR, Teboul JL: Assessment of indices of preload and vol- obtained from a small sample of blood drawn back through ume responsiveness. Curr Opin Crit Care 2005;11:235–9. the catheter or by using an oximeter-tipped CVP catheter. [PMID: 15928472] Rivers E, et al. Early goal-directed therapy in the treatment of C. Complications—Inadvertent arterial insertion occurs severe sepsis and septic shock. N Engl J Med. 2001;345:1368–77. about 2% of the time; such insertion is particularly dangerous [PMID: 11794169] if large, rigid “introducer” catheters are inserted. Perforation Rivers E: Mixed vs central venous oxygen saturation may be not of the superior vena cava is associated with a 67% mortality numerically equal, but both are still clinically useful. Chest rate, whereas the rate associated with laceration of the right 2006;129:507–8. [PMID: 16537845] 196 CHAPTER 8 passes through the right ventricle, a wedgelike pressure trac- Rivers EP, Ander DS, Powell D: Central venous oxygen saturation monitoring in the critically ill patient. Curr Opin Crit Care ing may be obtained. This “pseudowedge” is due to engage- 2001;7:204–211. [PMID: 11436529] ment of the catheter tip beneath the pulmonary valve or Taylor RW, Palagiri AV: Central venous catheterization. Crit Care within trabeculations. Withdrawal of 10 cm of the catheter Med 2007;35:1390–6. [PMID: 17414086] will solve the problem. Overinflation of the balloon, causing it to herniate over the tip of the catheter, results in a pressure Pulmonary Artery Catheterization tracing that continues to rise to high levels. The balloon should be deflated and a short length of catheter withdrawn Catheterization of the pulmonary artery is a useful addition before further advancement is attempted. to CVP monitoring. It provides information related to left The final position of the catheter tip within the pul- heart filling pressures and allows sampling of pulmonary monary artery is critical. This may be described with refer- artery blood for determination of mixed venous oxygen sat- ence to three lung zones that depend on the relationship of uration. Thermodilution cardiac output measurements are airway and vascular pressures (Figure 8–9). In zones I and II, made using a thermistor-tipped catheter. mean airway pressure is intermittently greater than pul- As the balloon flotation catheter is advanced through the monary venous pressure, which results in collapse of the vas- heart, characteristic pressure waveforms are obtained that culature between the catheter tip and the left atrium. In this indicate the position of the catheter’s distal port (Figure 8–8). position, observed pressures will be more indicative of air- Simultaneous electrocardiographic monitoring ensures that way pressure than of left atrial pressure. Only in zone III is ventricular tachyarrhythmias will be detected as the there an uninterrupted column of blood between the catheter traverses the right ventricle. After a pulmonary catheter and the left atrium. In the supine position, zone III capillary wedge tracing is obtained, the catheter should be assumes a more dependent position, caudal to the atrium. deflated and withdrawn until only 1 mL of inflation is Decreased airway pressures change the ventilation-perfusion required to advance from a pulmonary artery to a capillary relationship, producing a relative increase in zone III. wedge tracing. Insertion of excessive catheter length con- Hypovolemia decreases vascular pressures and decreases tributes to intracardiac knotting. If subsequent pressure zone III. tracings are not obtained within 15 cm of additional inser- Correct catheter position should be ensured by chest x- tion, looping should be suspected. When the catheter is ray. Although most catheters migrate caudally and to the placed through either the subclavian or the jugular vein, the right, an occasional catheter will become wedged anterior to typical distances required are as follows: right atrium, 10–15 the vena cava. In this position, true pulmonary capillary cm; right ventricle, 20–30 cm; pulmonary artery, 45–50 cm; pressures may be less than alveolar pressures, resulting in and pulmonary capillary wedge, 50–55 cm. As the catheter spuriously elevated measurements. A lateral chest x-ray will mm Hg Figure 8–8. Normal pressures and waveforms obtained as a pulmonary artery flotation catheter is advanced from the right atrium to a pulmonary artery wedge position. (Reproduced, with permission, from Morgan GE, Mikhail MS: Clinical Anesthesiology. Originally published by Appleton & Lange. Copyright © 1992 by The McGraw-Hill Companies, Inc.) INTENSIVE CARE MONITORING 197 Figure 8–9. The effect of airway pressure on the pulmonary vasculature is divided into three zones. A pulmonary artery flotation catheter should wedge in zone III, where there is a continuous column of blood between the pul- monary capillary and the left atrium. (Reproduced, with permission, from West JB, Dollery CT, Naimark A: Distribution of blood flow in isolated lung: Relation to vascular and alveolar pressures. J Appl Physiol 1964;19:713.) demonstrate when the catheter has assumed this position. the mean pulmonary capillary wedge pressure and has been Indicators of proper tip placement include (1) a decline in used to estimate left ventricular pressure when the pul- pressure as the catheter moves from the pulmonary artery monary capillary wedge pressure is not available—but this is into the “wedged” position, (2) ability to aspirate blood from unreliable and inconsistent in the case of lung disease, pul- the distal port (eliminating the possibility of overwedging), monary hypertension, or tachycardia. Normal values for pul- and (3) a decline in end-tidal CO2 concentration with infla- monary artery catheter pressures are shown in Figure 8–8. tion of the balloon (produced by a rise in alveolar dead Pulmonary capillary filtration pressure (Pcap) is a measure space). In a patient receiving positive end-expiratory pres- of the potential difference that drives fluid from the pulmonary sure (PEEP), another indicator of correct positioning is an vasculature into the perivascular interstitial and alveolar spaces. increase pulmonary capillary wedge pressure less than 50% The contribution of hydrostatic and osmotic pressure differ- of any increase in PEEP. This is so because normal lung and ences to fluid filtration is described by Starling’s law. The equa- chest wall compliances are approximately equal at end expi- tion relating mean PA pressure, PCWP, and Pcap is ration. Therefore, intrathoracic pressure will increase by 50% of PEEP, and pulmonary capillary wedge pressure also will Pcap = PCWP + 0.4 × (PA – PCWP) increase by at most 50%. Lung disease (decreased lung com- pliance generally) will distort this relationship, but almost Adult respiratory distress syndrome (ARDS) widens the always to less than 50%. If the pulmonary capillary wedge PA-to-PCWP gradient and increases Pcap, contributing to pressure rises more than 50% of the PEEP, repositioning pulmonary edema. must be considered. The pulmonary capillary wedge (pulmonary capillary Clinical Applications occlusion) pressure (PCWP) estimates left ventricular end- diastolic pressure and thus serves as an estimate of left ven- A. Pressure Measurements—In most instances, PCWP is tricular preload. Because the pulmonary vasculature is a an accurate indicator of left ventricular end-diastolic pres- low-resistance circuit, the pulmonary artery end-diastolic sure. Correlation between CVP and PCWP may be poor in pressure in normals is usually only 1–3 mm Hg higher than critically ill patients with cardiopulmonary disease because 198 CHAPTER 8 of differences between right and left ventricular function. In Pulmonary artery blood should be withdrawn slowly to this group, both absolute values and relative changes in CVP avoid inadvertently pulling “pulmonary capillarized” blood are unreliable because alterations in the pulmonary vascular with a misleadingly higher O2 saturation. bed affecting the right side of the heart do not equally affect Mixed venous oxygen saturation is an indicator of sys- the left ventricle. This is of particular importance following temic oxygen. utilization. Normally, peripheral oxygen con- pulmonary embolization, which increases right ventricular sumption (VO2) is independent of oxygen delivery (DO2). afterload without affecting left ventricular end-diastolic Therefore, as cardiac output and oxygen delivery decline, pressure. CVP and pulmonary systolic and diastolic pres- peripheral oxygen extraction increases to keep consumption sures are all elevated, whereas PCWP is decreased because of constant. This results in decreased mixed venous oxygen sat- the decline in forward flow. uration. Conversely, sepsis may cause a reduction in periph- PCWP correlates best with left atrial pressure (LAP) when eral oxygen consumption, thereby increasing mixed venous the latter is less than 25 mm Hg. However, PCWP will be oxygen saturation. lower than LAP in hypovolemic patients, whose pulmonary The partial pressure of oxygen in mixed venous blood is vasculature collapses during peak inspiration. When LAP normally about 40 mm Hg, resulting in a hemoglobin satu- increases to more than 25 mm Hg—which may occur after ration of 75%. Oxygen content can be calculated for both acute myocardial infarction with decreased left ventricular arterial and venous hemoglobin saturations (%Sat Hb) using compliance—PCWP tends to underestimate left-ventricular the following formula: end-diastolic pressure (LVEDP). As left ventricular function deteriorates, the contribution that atrial contraction makes to CXO2 = 1.34 × Hb × %Sat + (0.0031 × PXO2) left ventricular filling is increased, and LVEDP can be signifi- cantly higher than PCWP. Several conditions affect the accu- If hemoglobin concentration is in grams per deciliter, racy of PCWP as an indicator of LVEDP. In mitral stenosis, oxygen content is expressed in milliliters per deciliter. left atrial pressure at end diastole may be significantly higher Dissolved oxygen (0.0031 × PO2) contributes minimally to than left ventricular pressure. This is diagnosed by the pres- oxygen content but may become significant in patients who ence of large v waves on a PCWP tracing. Large left atrial are profoundly anemic. The normal arteriovenous oxygen myxomas also elevate PCWP. Aortic regurgitation produces content difference—C(a–v)O2—is 5 mL/dL. Hypovolemia and an underestimation of LVEDP by PCWP because the mitral cardiogenic shock both increase the difference (>7 mL/dL), valve closes early despite increasing left ventricular pressure. whereas sepsis decreases it (15 mm Hg) can result in pulmonary tinuous estimates of oxygen extraction and intrapulmonary vascular collapse, causing PCWP to reflect airway pressure shunting. From continuous.. oximetry data, the ventilation- instead of left atrial pressure (conversion from zone III to perfusion index (V/QI) can be calculated by the following zone I). As with CVP, an esophageal pressure transducer per- equation: mits calculation of transmural rather than transthoracic pres- V 1.32 + Hb × (1 − SpaO2 ) + (0.0031 × PAO2 ) sures. However, because pulmonary compliance is not disturbed uniformly, the pressure obtained through the = esophageal probe may not correctly reflect the pressure that QI 1.32 × Hb × (1 − S vO2 ) + (0.0031 × PAO2 ) surrounds the pericardium. While PCWP often closely estimates LVEDP, these values where PAO2 is alveolar. oxygen tension, calculated from may not accurately reflect true LV preload, which is a func-...the alveolar gas equation.V/QI correlates well with shunt (Qs/Qt) tion of LV end-diastolic volume and stretch of myocardium. over a wide range of parameters and clinical conditions. Patients with LV hypertrophy, diastolic heart failure, and LV ischemia have distorted relationships between LVEDP and C. Complications—Complications of pulmonary arterial ventricular preload. Consequently, a single PCWP measure- catheterization may occur both on insertion and subse- ment may be less helpful than therapeutic trials of volume quently. The incidence of a pneumothorax with either the loading or diuretics with serial measurements. subclavian or internal jugular approach is 2–3%. Catheter knotting is related to the size of the catheter and the insertion B. Mixed Venous Oxygen Saturation—Mixed venous oxy- length. Smaller catheters knot more frequently, as do those gen saturation (S–vO2) is obtained from blood from the pul- with excessive redundancy in the ventricle. The incidence of monary artery drawn out of the distal port of the catheter. catheter-induced transient right bundle branch block is INTENSIVE CARE MONITORING 199 between 0.1% and 0.6% and is thought to be caused by direct National Heart, Lung, and Blood Institute Acute Respiratory trauma to the bundle of His. The incidence increases to as Distress Syndrome (ARDS) Clinical Trials Network: high as 23% in patients with preexisting left bundle branch Pulmonary-artery versus central venous catheter to guide treat- block. Ventricular arrhythmias also may occur, although they ment of acute lung injury. N Engl J Med 2006;354:2213–24. are usually transient and do not require treatment. Other [PMID: 16714768] complications that may occur during insertion include tra- Pinsky MR, Vincent JL: Let us use the pulmonary artery catheter cheal laceration, innominate artery injury, and bleeding. correctly and only when we need it. Crit Care Med Pulmonary artery rupture may occur at the time of place- 2005;33:1119–22. [PMID: 15891346] ment, as a result of laceration by the catheter tip, or subse- Sakr Y et al: Use of the pulmonary artery catheter is not associated with worse outcome in the ICU. Chest 2005;128:2722–31. quently, from overinflation of the balloon in the distal [PMID: 16236948] pulmonary artery. The overall incidence of rupture is sub- Shah MR et al: Impact of the pulmonary artery catheter in criti- stantially less than 1%. Contributory factors include distal cally ill patients: Meta-analysis of randomized clinical trials. position of the catheter, decreased vessel diameter (primary JAMA 2005;294:1664–70. [PMID: 16204666] pulmonary hypertension), systemic anticoagulation, and Summerhill EM, Baram M: Principles of pulmonary artery prolonged balloon inflation. Hemoptysis is usually the pre- catheterization in the critically ill. Lung 2005;183:209–19. senting sign. The need for complete removal of the catheter [PMID: 16078042] is debatable because the requirements for monitoring are compounded by the complication. The catheter should be withdrawn to a more proximal site and the patient posi- Cardiac Output tioned with the affected side down to optimize ventilation- The bedside technique of measuring cardiac output by ther- perfusion relationships. Emergency thoracotomy is required modilution added a new dimension to ICU monitoring. in rare cases when uncontrolled bleeding occurs. When a known quantity of hot (or cold) solution (indicator) Air embolism occurs most commonly with tubing is injected into the circulation, a time-temperature curve changes and transducer calibrations. Approximately 20 mL/s may be produced that allows calculation of flow. The area of air is required in adults before symptoms appear, with under the time-temperature curve is inversely proportional 75 mL/s required to produce hemodynamic collapse and to cardiac output. This is calculated using the Stewart- death. The precipitating cause is mechanical obstruction of Hamilton indicator dilution equation: right ventricular outflow by the air embolus. Patients should be placed in the left decubitus and steep Trendelenburg posi- VI × (TB − TI ) × SI × CI × 60 tion. This puts the outflow tract in a dependent location and Cardiac output = allows the air to rise in the ventricle. Aspiration of air through SB × CB the pulmonary artery catheter has been reported with varying results. Obstruction of the pulmonary vasculature by the where VI is the volume of injectate (mL) and TB, TI, SI, SB, CB, embolus results in hypoxemia, increased pulmonary artery and CI are temperature, specific gravity, and specific heat of pressure, and right ventricular dysfunction. Passage of air blood (B) and indicator (I), respectively. through a patent foramen ovale may cause cerebral emboliza- tion and stroke. Thromboemboli may originate from the tip or body of SI × CI = 1.08 for D5W the catheter and can result in pulmonary emboli. Catheters SB × CB left in place for long periods may cause subclavian or jugular venous thrombosis. Other complications include infective endocarditis, sepsis, aseptic thrombotic endocarditis, and Either iced or room-temperature thermal boluses can be rupture of the chordae tendineae. used, although the use of iced fluid slightly improves the The avoidance of sepsis from pulmonary arterial signal-to-noise ratio. Room-temperature boluses generally catheters is identical to the protocols for central venous are adequate except when cardiac output is very low. For best catheters. Daily sterile catheter care, dressing changes, and results, the difference between the blood’s temperature and regular rotation of insertion sites are critical to minimize that of the injectate should be at least 12°C, which is easily catheter-related infections. achieved with room-temperature injectate. Bolus injection speed and warming of the indicator as it passes through the Binanay C et al: Evaluation study of congestive heart failure and catheter have only minimal effects (±3%). When technique is pulmonary artery catheterization effectiveness: The ESCAPE optimal, measurement repeatability is within 10%. Severe trial. JAMA 2005;294:1625–33. [PMID: 16204666] cardiac arrhythmias may reduce repeatability and yield Harvey S et al: Assessment of the clinical effectiveness of pul- results that may not accurately reflect average cardiac output. monary artery catheters in management of patients in intensive Timing of injection with a particular phase of respiration care (PAC-Man): A randomised, controlled trial. Lancet (end expiration) improves consistency of measurements. 2005;366:472–7. [PMID: 16084255] Excessive patient movement also may produce erratic results. 200 CHAPTER 8 Continuous thermodilution cardiac output measure- yields results as good as those obtained with the suprasternal ments can be obtained using special pulmonary artery technique and offers the added advantage of providing data catheters. In one type, the right ventricular segment of the continuously. A recently developed transtracheal probe uses catheter warms the blood by a small amount above body a pulsed Doppler probe attached to the distal end of an endo- temperature. A sensitive downstream thermistor records the tracheal tube. Controlled studies have shown good accuracy, change in temperature. In another type, blood flow is esti- but extensive clinical experience is wanting. mated by how much electric current is needed to maintain a segment of the catheter at a temperature slightly above body C. Thoracic Bioimpedance—This noninvasive technique temperature. Blood flow is directly related, but in a complex measures stroke volume by passing a small alternating cur- manner, to the amount of energy needed for a constant tem- rent (2.5–4.0 mA) through the chest at radiofrequency perature. These methods correlate well with conventional 70–100 kHz. Four pairs of electrodes (one transmitter and bolus thermodilution but may differ in systematic or nonsys- one sensor) are required. Two pairs are placed at the base of tematic fashion. the neck and two at the level of the xiphoid process in the midcoronal plane. The change in thoracic impedance is due to blood flow, ventilation, and body movement. Respiratory Other Methods to Measure Cardiac Output variations occur much more slowly than those associated A. Indicator Dilution Cardiac Output—This technique with blood flow and can be eliminated by the computer algo- relies on dilution of a colored dye. A bolus of dye is injected rithm. Similarly, motion artifacts can be rejected by special intravenously through a central venous catheter while circuitry. The majority of systolic blood flow is due to pul- peripheral arterial blood is withdrawn. The arterial sample is satile blood flow in the descending thoracic aorta. Stroke vol- continuously passed through a densitometer. The area under ume is obtained by analyzing the impedance change over a the dye curve is calculated, and a modification of the cardiac cycle. Heart rate is determined at the same time and Stewart-Hamilton equation is applied. This techniques is multiplied by stroke volume to yield cardiac output. Other rarely used in the ICU. parameters obtained include ejection velocity index, thoracic fluid index, and ventricular ejection time. The thoracic fluid index is thought to correlate with extravascular lung water, 60 × indicator dose (mg) whereas ejection time has been used as a parameter of car- Cardiac output = average concentration × time diac function. Because bioimpedance cardiac output measurements are noninvasive, they can be repeated frequently. The volume of B. Doppler Ultrasound—Doppler devices measure ascend- electrically participating tissue (VEPT) is critical in determi- ing aortic flow and calculate cardiac output. A continuous- nation of the stroke volume. Although changes in body habi- wave Doppler probe is placed in the sternal notch to measure tus are included in the nomogram used to calculate VEPT, the velocity of aortic blood flow. A separate A-mode pulsed small changes can produce significant error. Similarly, elec- Doppler probe is centered in the third or fourth anterior trode placement is important. A 2-cm change in the distance intercostal space to measure the cross-sectional diameter of between the sensing electrodes will produce a 20% variation the aortic root. The stroke volume is the product of the in recorded cardiac output. Overall correlation with ther- cross-sectional area and the average blood velocity. Cardiac modilution in adults has been only fair. The method is fur- output is calculated by multiplying the heart rate and the ther limited by inaccuracies caused by dysrhythmias. stroke volume. Potential sources of error include (1) mis- Furthermore, readings are difficult during patient move- alignment of the Doppler beam, which produces errors in ment, including shivering. Cardiac output is overestimated measurement of blood velocity, (2) the assumption that the when preload is reduced; in low-flow states, when inotropes aorta is circular, and (3) the assumption that aortic blood are required; and with aortic insufficiency. Underestimation flow is laminar. Each of these factors accounts for a cardiac is produced by hyperdynamic sepsis, hypertension, and output error that approaches 15% when compared with intracardiac shunts. determinations obtained by other means. The difference between suprasternal Doppler ultrasound cardiac output D. Fick Method—Cardiac output may be calculated by relat- and standard thermodilution has been reported to range ing oxygen consumption to arterial and mixed venous oxy- from –4.9 to +5.8 L/min. An esophageal probe is now avail- gen saturation using Fick’s equation: able that measures descending aortic flow. An insertion depth of about 30 cm is required to reach the esophageal V O2 “window.” The aortic root is sized using an A-mode pulsed Cardiac output = C(a − v)O2 × 10 Doppler, and a single measurement of ascending aortic flow is performed with a continuous-mode suprasternal probe. The esophageal probe is then calibrated against the cardiac Calculation of cardiac output using Fick’s equation is the output obtained by the suprasternal technique. This method reference with which all other techniques are compared. The INTENSIVE CARE MONITORING 201 arteriovenous oxygen content difference requires that a pul- the introduction of new cardiac output computers that monary artery catheter be placed to obtain mixed venous measure the injectate’s temperature and automatically enter blood. Oxygen consumption is calculated by measuring the the value into the calculation. oxygen content difference between inspired and exhaled gas. Right-to-left intracardiac shunt will result in loss of the A modification of this technique substitutes mixed venous indicator, causing a falsely elevated cardiac output. Left-to- CO2 (rebreathing), arterial PCO2, and expired volume of CO2 right shunts permit recirculation of indicator that has for the corresponding oxygen values in the Fick equation. already passed through the lungs. This produces multiple peaks in the time-temperature curve that cannot be inter- E. Pulse Waveform Analysis—A noninvasive method that preted by the cardiac output computer, resulting in a bad shows some promise despite varying reported accuracy uses curve alert. When tricuspid regurgitation occurs, blood and algorithmic analysis of the arterial pulse waveform. The indicator mix, resulting in prolongation of transit time. The waveform bears a certain relationship with stroke volume curve produced has a slow upstroke and decay, thereby but is modified a great deal by the capacitance, impedance, increasing the area underneath it. This causes sporadic read- and other characteristics of upstream and downstream arte- ings and underestimation of the true cardiac output. rial beds. The aortic impedance plays a major role in this relationship and is likely to differ between patients. Thus Derived Parameters “calibration” of a noninvasive system (or one using an arte- rial catheter) using the arterial waveform with thermodilu- Cardiac output measurements may be combined with sys- tion cardiac output has been necessary. Recently, several temic arterial, venous, and pulmonary artery pressure deter- commercially available systems have been reported to have minations to calculate a number of hemodynamic variables adequate agreement with conventional measurements. useful in assessing the overall hemodynamic status of the patient (Table 8–1). Oxygen transport parameters also may Sources of Error be calculated (see below). Use of correct temperatures and volumes is the most impor- Cholley BP, Payen D: Noninvasive techniques for measurements of tant factor contributing to accurate thermodilution cardiac cardiac output. Curr Opin Crit Care 2005;11:424–9. [PMID: output results. If the amount of indicator injected is less than 16175028] the amount used in calculation, the fall in indicator temper- ature will be less than anticipated, and the cardiac output Pulse Oximetry will be falsely elevated. Cardiac output also will be falsely elevated if the injectate is warmer than that used in the cal- Pulse oximetry affords a noninvasive estimate of arterial oxy- culation. The latter problem has been largely overcome with gen saturation using the change in light absorption across a Table 8–1. Hemodynamic calculations and normal ranges. Formula Normal Range Stroke volume, mL 1000 × CO (L/min) 60–90 ml HR (beats/min) Stroke volume index, mL/m2 Stroke volume (mL/beat) 30–65 ml BSA (m2) Cardiac index, L/min/m2 CO (L/min) 2.8–4.2 L/min/m2 2 BSA (m ) 5 Systemic vascular resistance, dyne-s/cm [Mean BP (mm Hg) – CVP (mm Hg)] 1200–1500 dyn-s/cm5 × 80 CO (L/min) Pulmonary vascular resistance, dyne-s/cm5 [Mean PAP (mm Hg) – PAWP (mm Hg)] 100–300 dyn-s/cm5 × 80 CO (L/min) CO = cardiac output; BSA = body surface area; BP = systemic blood pressure; CVP = central venous pressure; PAP = pul- monary artery pressure; PAWP = pulmonary artery wedge pressure. 202 CHAPTER 8 vascular bed during the arterial pulse. In the ICU, pulse devices are programmed to avoid reporting O2 saturation oximetry has important uses and has become a standard of when low perfusion or a poor pulse signal is being measured. care in many institutions. There are, however, a number of In some of the few studies considering these issues in the ICU, issues that should be understood and considered with this failure of the pulse oximeter to measure O2 saturation was not monitoring technique. In particular, the reliability of this infrequent in patients with hemodynamic instability method may be limited in patients with severe hypoxemia, (12–15%). However, other studies have demonstrated that abnormal arterial pulsations, and hypoperfusion of the site some pulse oximeters continue to measure and report O2 sat- of measurement. uration despite very poor blood flow and severe hypotension. When light of a particular wavelength is transmitted These results may not be reliable, and there is concern that through a clear solvent containing solute that absorbs light at pulse oximeter O2 saturation under these conditions may be that wavelength, the amount of light absorbed is the product misleadingly high. Pulse oximeter technology continues to of solute concentration, path length, and the extinction coef- evolve. The latest-generation devices have improved resist- ficient (determined by the solute and the wavelength). For a ance to motion artifact and low perfusion. These are expected hemoglobin solution, the relative concentrations of oxy- and to be more reliable and accurate in the ICU setting. deoxyhemoglobin can be determined in a spectrophotome- B. Abnormal Hemoglobins—The pulse oximeter cannot ter because the extinction coefficients are different for these measure carboxyhemoglobin nor accurately measure oxyhe- two hemoglobin species at certain wavelengths. moglobin in the presence of carboxyhemoglobin. The oxy- Pulse oximetry uses the beat-to-beat changes in light gen saturation displayed is essentially equal to the difference absorption through a vascular bed to estimate arterial O2 sat- between total hemoglobin and deoxygenated hemoglobin uration, discarding any nonvariable light absorption by con- (100% – the percentage of deoxyhemoglobin), but the relative sidering only the difference between peak and nadir light concentrations of oxy- and carboxyhemoglobin are intensities. The method determines O2 saturation by a com- unknown. Other substances in the blood may or may not plex calculation that includes several important assump- affect pulse oximetry. Bilirubin has little effect on pulse tions. With the use of two light-emitting diodes (LEDs) oximetry; methemoglobin, generated in the presence of oxi- producing light in the red and infrared ranges, pulse oxime- dizing agents such as nitrites and sulfonamides, usually try is able to estimate oxyhemoglobin as a proportion of the increases the difference between functional O2 saturation and sum of oxyhemoglobin plus deoxyhemoglobin—the so- oxyhemoglobin, but a sufficiently high methemoglobin con- called functional oxyhemoglobin saturation. Pulse oximeters centration also may have the peculiar effect of causing the are “calibrated” by comparison of arterial blood oxygen sat- pulse oximeter to read 85% regardless of other conditions. A uration in volunteers to calculated values; the devices use a number of dyes such as indocyanine green and methylene “look-up table” to translate the measured proportion to the blue also have effects on the accuracy of measurement. displayed saturation. Pulse oximetry is subject to artifact- caused errors. Movement of the oximeter probe, extraneous Clinical Applications incident light (especially if pulsatile), variations in arterial pulsation, dependent position, venous pulsations, and other Pulse oximetry has widespread usefulness in the ICU, espe- factors may result in incorrect O2 saturation readings. Pulse cially in adjusting inspired oxygen, during weaning from oximeters are most commonly transmission pulse oximeters, mechanical ventilation, and in testing different levels of in which light is passed through tissue (ear or fingertip) to a PEEP, inverse I:E ratio, or other mechanical ventilator adjust- sensor on the opposite side, but they may be reflectance pulse ments. Other uses include monitoring during procedures oximeters, in which light passes through tissue but is such as bronchoscopy, gastrointestinal endoscopy, cardiover- reflected back to a sensor on the same side as the light source. sion, hemodialysis, and radiography. Pulse oximetry is par- ticularly accurate in following O2 saturation in patients who Validity have mild to moderate hypoxemia (O2 saturation >75%) but without severe hypoperfusion or hypotension. It cannot be The accuracy of pulse oximetry is generally considered good regarded as a complete substitute for arterial blood gas deter- in the range of normoxia to mild hypoxemia. However, accu- minations partly because of the lack of PO2 and pH determi- racy may be suspect during more severe hypoxemia, such as nations but also because of the relationship between PO2 and when arterial O2 saturation is below 75%. In this range, dif- O2 saturation when the latter is above 90–95%. Results of ferences between measured O2 saturation and pulse oximetry pulse oximetry should be interpreted cautiously in patients saturation range from 5–12%. with carboxyhemoglobinemia or methemoglobinemia. A. Patient Factors—Patients in the ICU frequently have hypotension, poor distal extremity perfusion, and impaired Keogh BF, Kopotic RJ: Recent findings in the use of reflectance oxygen delivery—or are being given pharmacologic vasopres- oximetry: A critical review. Curr Opin Anaesthesiol sors or vasodilators. These factors affect blood flow to the site 2005;18:649–54. [PMID: 16534307] of pulse oximetry and vary the contour and intensity of the McMorrow RC, Mythen MG: Pulse oximetry. Curr Opin Crit Care 2006;12:269–71. [PMID: 16672788] beat-to-beat pulse used to calculate O2 saturation. Most INTENSIVE CARE MONITORING 203 Airway CO2 Monitoring In addition, if minute ventilation is measured, A disposable colorimetric device that detects CO2 can con- P ECO2 firm endotracheal tube placement and position. When the V CO2 (L/min, STPD) = 0.826 × VE (L/min, BTPS) × device tests positively for CO2, this confirms that the endo- PB tracheal tube is in the trachea. However, a negative result is not as reliable, and alternative means for checking tube where PB is barometric pressure. placement must be used. Continuous airway CO2 monitoring uses a rapidly Volumetric Capnography responding infrared CO2 analyzer. Capnography is a continu- ous display or recording of CO2 concentration during each Capnography records CO2 concentration against time dur- breath. Other devices may display the end-tidal CO2 fraction ing expiration. If CO2 is plotted against expired volume, then or partial pressure (PETCO2). a semiquantative estimate of dead space:tidal volume ratio is The infrared analyzer uses an appropriate wavelength of obtained. Equipment for doing volumetric capnography can infrared light for which the CO2 concentration is propor- be built into mechanical ventilators. Volumetric capnogra- tionate to the absorption of the light. It has the advantage of phy has been used to help diagnose pulmonary embolism relatively low cost, real-time sampling, reliability, ease of cal- and theoretically should be applicable to other situations ibration, and acceptable response time. where dead space:tidal volume ratio is useful (eg, weaning, Capnography shows a continuous display of expired and, if ARDS, and asthma). desired, inspired CO2 concentration or partial pressure. The expired CO2 waveform can give a qualitative assessment of the Validity degree of ventilation-perfusion mismatching. For example, the steepness. of As described earlier, end-tidal PCO2 should not be used as an. the slope of the “alveolar plateau” indicates more severe V/Q mismatching because it demonstrates empty- accurate estimate of PaCO2. Patients with either worsening of ing of progressively less well ventilated lung units compared gas exchange function (increased PaCO2) or improvement in with a waveform.showing a flatter alveolar plateau in a patient function (decreased PaCO2) can have a fall in PETCO2. The. with less severe V/Q mismatching. The inspiratory segment former occurs because of an increase in P(a–ET)CO2; the lat- also should be inspected to confirm that the inspired gas is free ter represents a parallel fall in both PETCO2 and PaCO2. of CO2 as a result of malfunction of the ventilator’s expiratory However, among the relatively few reports involving ICU valve or some other component. patients—most of whom were receiving mechanical ventila- tion—some indicate that PaCO2 and PETCO2 track together rel- atively well, with mean differences less than 5 mm Hg and no End-Tidal and Mixed Expired PCO2 change in difference during weaning or extubation. In COPD patients, the difference was considerably higher (as much as 9 In normal subjects at rest and breathing at a normal tidal vol- mm Hg) but, again, relatively constant. In contrast, other stud- ume and respiratory rate, PETCO2 is close numerically to arte- ies have found that P(a–ET)CO2 varies considerably, and while rial PCO2, with the usual difference between PaCO2 and there was correlation with VD/VT, there was a lack of a con- PETCO2 0–4 mm Hg (P[a–ET]CO2). In patients with respira- stant value for P(a–ET)CO2 that would allow “tracking” of tory failure,.. contribution to expired gas from dead space and PaCO2 from PETCO2 alone. In particular, one study demon- high V/Q lung units decreases CO2 concentration during strated that both increases and decreases in P(a–ET)CO2 may expiration and at end expiration. The P(a–ET)CO2 becomes result from mechanical ventilator adjustments. increasingly large, with a strong correlation between P(a–ET)CO2 and the dead space–tidal volume ratio. The Clinical Applications PETCO2 should not be used as a substitute for PaCO2 in patients with lung disease. Furthermore, P(a–ET)CO2 cannot Airway CO2 monitoring has the advantage of being nonin- be assumed to remain constant in the face of lung disease vasive, and studies are available that indicate a decrease in and mechanical ventilation. the number of arterial blood gases obtained when this – ) The mixed expired CO2 fraction or partial pressure (PECO modality is used. However, it is clear that the critically ill 2 is usually determined from collection of expired gas for several patient with respiratory failure will have the largest and minutes. This should be distinguished from PETCO2 sampled at most unpredictable difference between PaCO2 and PETCO2; the end of a single breath. The mixed expired CO2 fraction can in these patients, PETCO2 is an unreliable estimate of PaCO2. be used with PaCO2 to calculate the dead space–tidal volume On the other hand, the difference between PaCO2 and ratio (VD/VT) using the modified Bohr equation: PETCO2 can be used as a measure of dead space:tidal volume ratio and therefore as a measure of the severity of gas exchange derangement. VD PaCO2 − P ECO2 = Although studies are lacking on the benefit of routine mon- VT PaCO2 itoring of airway CO2, capnography and PETCO2 monitoring 204 CHAPTER 8 have been used in several clinical situations. First, airway CO2 whereas in children it tends to be higher. (Values in neonates monitoring can provide rapid noninvasive assurance of correct may be very close to equal.) However, when either cardiac endotracheal tube placement. The capnogram should show output or regional perfusion is decreased, the ratio of increasing CO2 concentration during expiration, and PETCO2 transcutaneous-to-arterial PO2 is decreased in proportion to should be a plausible value. Second, PETCO2 has been used dur- the level of decreased perfusion. Hence transcutaneous oxy- ing cardiopulmonary resuscitation as a measure of the effec- gen monitoring may be used as a monitor of both oxygena- tiveness of artificial circulatory assistance; a very low PETCO2 tion and perfusion. A low PtcO2 value is an indicator that the suggests that venous blood is not adequately returning to the patient is either hypoxemic or in a low-flow state (or has central circulation. Third, the combination of arterial and end- reduced regional perfusion). tidal PCO2 provides an estimate of the inefficiency of ventila- Transcutaneous PCO2 (PtcCO2) has been measured using tion (VD/VT). Some researchers have suggested that a modified PCO2 electrode attached to the skin surface. In P(a–ET)CO2 can be used to titrate the optimal amount of PEEP. contrast to PtcO2, however, CO2 is more soluble than O2, so While the smallest difference in P(a–ET)CO2 has correlated with tissue stores of CO2 act as a buffer, reducing the dependence the highest degree of tissue oxygen delivery, this measurement of PtcCO2 on blood flow and metabolism. In theory, PtcCO2 has not proved ideal in all studies. Finally, it has been suggested should mirror PaCO2 more closely than PtcO2 reflects PaO2, that capnography can help in weaning patients from mechani- and there is no need to heat the skin at the monitoring site. cal ventilation, but the predictive value of airway CO2 monitor- Newly designed sensors with heaters have been studied ing in this clinical situation is unclear. recently. Results have been variable. Both PtcO2 and PtcCO2 devices should be calibrated Belpomme V et al: Correlation of arterial PCO2 and PETCO2 in pre- against known PO2 and PCO2. Because of the heating of the hospital controlled ventilation. Am J Emerg Med 2005; PtcO2 electrode site, the location must be changed every 4–6 23:852–9. [PMID: 16291440] hours to minimize the risk of thermal injury. Kallet RH et al: Accuracy of physiologic dead space measure- ments in patients with acute respiratory distress syndrome using volumetric capnography: Comparison with the meta- Clinical Applications bolic monitor method. Respir Care 2005;50:462–7. [PMID: Many neonatal ICUs routinely employ PtcO2 monitoring and 15807908] have found good correlation with arterial blood gases except in Moon SW et al: Arterial minus end-tidal CO2 as a prognostic fac- patients with severe cardiac compromise. In adults, PtcO2 tor of hospital survival in patients resuscitated from cardiac arrest. Resuscitation 2007;72:219–25. [PMID: 17101205] measurement is best used as a measure of tissue hypoperfusion. Verschuren F et al: Volumetric capnography as a screening test for A reduction of PtcO2 may be an early indicator of low flow, par- pulmonary embolism in the emergency department. Chest ticularly if pulse oximetry does not indicate severe hypoxemia. 2004;125:841–50. [PMID: 16117730] Kagawa S, Severinghaus JW: Errors in monitoring transcutaneous PCO2 on the ear. Crit Care Med 2005;33:2414–5. [PMID: Transcutaneous Blood Gases 16215403] Kagawa S et al: Initial transcutaneous PCO2 overshoot with ear Using transcutaneous blood gas monitors, partial pressures probe at 42ºC. J Clin Monit Comput 2004;18:343–5. [PMID: of oxygen and carbon dioxide may be measured in the tissue 15957625] beneath heated skin electrodes. This monitoring technique has value because it reflects tissue levels, but it cannot yet be employed as a substitute for blood gas monitoring. Respiratory Mechanics Measured parameters are tidal volume, vital capacity, airway pressure, and intrathoracic pressure. From these, respiratory Principle system and lung compliance, airway resistance, and work of The Clark electrode, similar to that used in blood gas analyz- breathing can be estimated. Modern mechanical ventilators ers, has been modified to be used on the skin surface. The often are equipped to measure airway pressure, tidal volume, skin in the area of the electrode is heated to 43–45°C. This inspiratory flow, and other derived values. They may be able heating is necessary to make the skin permeable to oxygen, to display in real time flow-volume or pressure-volume but it has the additional effect of increasing perfusion in the loops. A discussion of respiratory compliance and resistance tissues beneath the probe. Since transcutaneous PO2 (PtcO2) is found in Chapter 12. reflects the oxygen tension level of the tissue beneath the probe, values may be affected either by arterial oxygenation Tidal Volume or by systemic and regional perfusion. At relatively normal cardiac output and with normal regional blood flow, PtcO2 In the ICU, tidal volume is measured most commonly in values reflect arterial PO2 values. In adults, the ratio of patients who have endotracheal tubes and require mechanical transcutaneous-to-arterial PO2 is normally about 0.8, ventilation. Respiratory inductive plethysmography provides INTENSIVE CARE MONITORING 205 a noninvasive estimate of tidal volume. Tidal volume should Lung and Chest Wall Compliance be monitored frequently in patients receiving mechanical ventilation. When volume-preset modes are used, a difference Calculation of compliance of the respiratory system (chest in expired volume compared with preset volume indicates wall and lungs together) is reviewed in Chapter 12. The com- that there is a leak in the ventilator circuit, that inspiratory ponents of respiratory system compliance can be subdivided flow demand is extremely high, or that inspiratory peak pres- into chest wall and lung compliances. Lung compliance (CL) sure exceeds the preset limit. In pressure-controlled ventila- is calculated as the ratio of change in volume (ΔV) to change tion, tidal volume is used to adjust the level of set airway in pressure (ΔP), where ΔV is usually the tidal volume and pressure, and any change in expired tidal volume indicates a ΔP is the difference between end-inspiratory and end- change in lung or chest wall compliance or airway resistance. expiratory transpulmonary pressure. Transpulmonary pres- During spontaneous respiration, tidal volume monitoring sure is the pressure difference between the pressure in the using noninvasive measurement can be employed to help airway and the esophageal pressure. Normal lung compliance identify patients with obstructive sleep apnea or abnormal is about 200 mL/cm H2O at end-expiratory volume. If chest breathing patterns (Cheyne-Stokes respiration). wall compliance (CCW) is desired, the formula 1/CRS = 1/CL + 1/CCW can be used, where RS is the respiratory system). Decreased lung compliance has been used as a criterion of Maximum Inspiratory and Expiratory Airway ARDS in some clinical studies but is not usually required for Pressure its clinical diagnosis. Low lung or chest wall compliance sug- gests increased work of breathing and could suggest that Inspiratory and expiratory maximum pressures are deter- weaning would be difficult or inappropriate. On occasion, mined by a manometer connected either to a mouthpiece or abnormal chest wall compliance as a cause of respiratory fail- to tubing adapted to fit onto the endotracheal tube. These ure is not identified unless measured. If low chest wall com- pressures are measured correctly starting at functiona