4600 Midterm Review (PDF)
Document Details
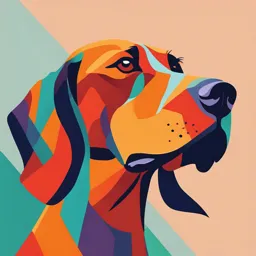
Uploaded by StrongerRainbow
Tags
Summary
This document reviews ion channels, specifically focusing on store-operated calcium channels (SOCCs). It details the roles of these channels in maintaining calcium homeostasis and their involvement in various cellular processes like signaling and differentiation.
Full Transcript
L1: Intro to Ion Channels What are Ion Channels? - Protein subunits combine to form a channel - Can be leaky (opens spontaneously) or gated (ligand gated, voltage gated) - Ion transporters play an important role in maintaining the uneven distribution of ions in subcellular compartme...
L1: Intro to Ion Channels What are Ion Channels? - Protein subunits combine to form a channel - Can be leaky (opens spontaneously) or gated (ligand gated, voltage gated) - Ion transporters play an important role in maintaining the uneven distribution of ions in subcellular compartments - Located on plasma membrane or endocytic membranes of intracellular organelles - Driving forces include concentration gradients, electrical gradients - Voltage across the membrane determines direction of ion flow; the inside of the cells negative and thus attracts positive ions such as sodium or calcium - Chemical synapses have ligand gated ion channels; activation of LGCs causes a voltage change that usually opens voltage gated ion channels (INTERcellular signaling) - Electrical synapses have connexin channels that allow passive flow of ions - Ion channels at the EM of organelles mediate ionic movement between cytosol and organelle lumen spaces (INTRAcellular signaling) Store Operated Ca2+ Channels - Changes in cytosolic calcium is a key signal in cell physiology, causing both rapid and slow responses - Rapid responses usually occurs in excitable cells - Ex. AP arriving at axonal terminal causes VG calcium channels to open, causing Ca2+ influx, Ca2+ binds synaptotagmin to cause NT release from vesicles - Ex. calcium ions in muscle cells bind troponin-c to expose myosin binding sites to trigger muscle contraction - Slow responses due to long lasting calcium influx can occur in excitable and non excitable cells - Long Lasting calcium entry through SOCCs can increase basal cytosolic Ca2+ concentration, resulting in gene transcriptions, cell differentiations, plasticity, and migration - Rapid vs slow responses depends on the location of calcium channels and calcium binding proteins - Most calcium channels / binding proteins have specific EF- hand domains to bind calcium Ex. Calmodulin - Will bind 4 calcium molecules to cause conformational change, and thus activation - CaM can bind calcium and activate: - 1) calcineurin: activation causes it to dephosphorylate NFAT to activate it, stimulating it to migrate to the nucleus and effect gene transcription (T cell differentiation) - 2) Cam dependent kinases: such as myosin light chain kinase during muscle contraction - 3) Ca2+ pumps and cation/Ca2+ exchangers: remove calcium from cytosol, either to the ER or extracellular space, to maintain low cytosolic Ca2+ Calcium is NOT the signal, change in Vm is - Cytosolic calcium is 10000x lower than that of extracellular fluid, and lower than ER concentrations (stores) - Calcium will travel down its concentration gradient - The speed of calcium movement between intracellular compartments depends on the channel permeability to Ca2+, and the extent of concentration/electrical gradients Regulating Calcium In/Out of the ER - Ligand gated calcium channels on the ER membrane can mediate Ca2+ efflux - Activated GPCR → G alpha subunit leads to activation of PLC, which hydrolyzes PIP2 into DAG and IP3 - IP3 binds IP3R receptor on ER membrane to cause Ca2+ efflux - Calcium itself can bind RyR-2 on ER membrane to cause Ca2+ efflux - L-type calcium voltage gated channels can cause a physical conformational change that opens RyR-1 on SR of smooth muscle cells, to cause Ca2+ efflux (physical coupling) Mitochondria Associated Membrane: - Zones of close contact between ER and mitochondria - VDACs on outer mitochondrial membrane allow Ca2+ influx to the mitochondria - MCU on inner membrane allow Ca2+ influx to the mitochondria - VDACs/MCU is held in close proximity to ER channels like IP3R by the grp75 protein - Intra- mitochondrial Ca2 can be transported out into the cytosol by H+/Ca2+ exchanger LETM1, and Na+/Ca2+ exchanger NCLX How to return to low cytosolic Ca2+ - When cytosolic calcium increases, it binds calmodulin to cause activation of SERCA - SERCA refills ER calcium stores, requires ATP - When cytosolic calcium increases, it binds calmodulin to cause activation of PMCA (plasma membrane calcium atpase) and (NCLX) Na+/Ca2+ - PMCA/NCLX transport calcium into the extracellular space - Therefore, cells use a lot of energy (ATP) to maintain subcellular [Ca2+] L2: Store Operated Calcium Channels - An increase in cytosolic calcium will cause activation of SERCA to refill ER stores (mediated by Ca2+/CaM) - But HOW do we get the calcium to refill the ER? - SOCCs are triggered to open after stimulus of ER calcium depletion STIM1 - A transmembrane protein in the ER membrane that senses Ca2+ release, and undergoes oligomerization - EF-hand domains on the luminal side of the ER binds calcium to sample ER [Ca2+] - Unbinding of calcium due to depletion of ER stores causes the SAM domain to initiate structural change - The CC1 domain will unfold/stretch such that the SOAR+ domain is released from the Ca3 motif - Lysine rich domains (positive charge) interact with orai proteins on the plasma membrane (via their CT-CCD-) - Lysine domains also interact with negatively charged phospholipids on the PM to stabilize this interaction - Two STIM1 molecules (the protein complex is a dimer) form a lose dimer where the CC1 domain is folded and the Ca3+ motif holds the SOAR+ inactive ORAI1 - A transmembrane protein with 4 transmembrane domains and intracellular C and N terminals - The negatively charged coiled domain at the C terminus (CT-CCD) interacts with the positive SOAR domain of STIM1 proteins, causing the formation of a CRAC channel - There is a 1:1 molecule ratio, meaning that 6 ORAI proteins hold 6 STIM proteins to form a hexameric CRAC channel - The proline rich region in the NT of ORAI is critical for channel kinetics STIM1/ORAI1 - Mechanism of Action - In resting cells with a Ca2+ filled ER, the two STIM1 molecules are only PARTIALLY dimerized through soar domains, to form a lose homodimer - Upon ER depletion, STIM1 molecules unfold and connect to ORAI (SOAR - CT-CCD) to form CRAC channels CRAC Channel Electrophysiology: - CRAC channels have inward rectification, meaning that the current amplitude becomes larger (more ions flowing through the channel) when the membrane voltage becomes more negative, but current flow approaches zero (no ions flowing through) when membrane voltage becomes more positive - Therefore, the amount of Ca2+ rectification entry is enlarged by even a small change in membrane voltage - CRAC channels have low unitary conductance, with ion flow being ~9fs at Ca2+ concentration of 2mM - CRAC channels have high selectivity and permeation to Ca2+, and is 1000x greater than that of other cations (like sodium) ‘Atypical’ SOC Entry - Mediated by transient receptor potential channels (TRPCs) - Their structure is 6 transmembrane domains, with an extracellular loop between TM5 and TM6, which bends partially to form the channel pore - Both N and C terminals are located intracellularly and have a coiled coil domain - NT-CCD = positive - CT -CCD = negative - A TRPC channel can be a homomeric tetramer (TRPC1/TRPC1) or a heteromeric tetramer (TRPC1/TRPC3) - The current-voltage relationship is almost linear due to the non-selectivity for cations - TRPC SOCCS are often activated by stimulation of Gq/11 PCRs Gating of TRPC channels - STIM1 may gate TRPC1/3 channels via electrostatic interaction (ie. they do not have physical contact) - STIM1’s positively charged lysine residues (684-KK-685) interact with TRPC1’s negatively charged aspartates (639-DD-640) - STIM1’s psotively charged lysine residues (684-KK-685) interact with TRPC3’s negatively charged aspartates (697-DD-698) - STIM1 may gate TRPC1/3 channels via protein-protein interaction - STIM1 can gate homomeric TRPC1 channels using its SOAR+ domain to interact with negative CT-CCD of TRPC1 - STIM1 CANNOT gate homomeric TRPC3 channels because their CT-CCD and NT-CCD interact, shielding STIM1’s SOAR+ domain from interacting with the CT-CCD - STIM1 can gate heteromeric TRPC1/TRPC3 because TRPC1’s CT-CCD will dissociate the TRPC3 NT-CCD to allow SOAR+ access to the CT-CCD Pharmacology of SOCC’s - SOCC inhibitors: - Lanthanides block ORAI channels - 2-APB: will transiently activate ORAI mediated Ca2+ entry, but will completely block entry at high concentrations - BTP2: widely used in experiments - SOCC activators: - GalphaPCR agonists that increase PLC activity and IP3 production - Compounds that deplete intracellular Ca2+ stores such as ionomycin or thapsigargin (a SERCA inhibitor) L3: Dysregulation of SOCCs and Diseases Antigen Presentation to TCells - APCs, such as dendritic cells or macrophages, can engulf and process foreign peptides from bacteria to be specific antigens - They expose the antigen on the plasma membrane surface via MHC2 - APCs release chemokines, and T helper cells have chemokine receptors - This stimulates chemotaxis of the T cell to the APC such that it can be exposed to the antigen - CD4+ (T helper cells) use the TCR protein to selectively interact with MHC2, using CD4 protein to help with this interaction - The conjugation structure between APC and T cell is termed an immunological synapse - Binding of antigen to the TCR stimulates T cells by activating STIM/ORAI mediated CRACs, causing T cell proliferation and differentiation - Antigens are protein, lipid, or nucleic acids - They can either trigger production of a specific antibody (B lymphocytes) or bind a TCR of a T lymphocyte - APCs are phagocytic cells that process foreign materials to become an antigen and present it via MHC2 molecules MHC2/Antigen Processing in APCs A. Endocytosis of foreign protein B. Endosome is formed C. Foreign protein is digested in endolysosome D. Late endolysosome is formed E. MHC2, CLIP, and HLA-DM are synthesized in the ER F. MHC, CLIP, and HLA-DM are modified in the golgi G. HLA-DM catalyzes the exchange of CLIP2 for the antigen to MHC2 in the vesicle H. Antigen presentation to the PM via vesicular exocytosis MHC2/TCR Protein-Protein Interaction 1. CD4 in T cells interacts specifically with MHC2 in APCs to help determine the specificity of antigen presentation a. CD28 in T cells is a costimulatory receptor for CD80 (helps stabilize the interaction) 2. Antigen interaction with the TCR activates tyrosine kinase complexes such as ZAP70 and LAT, causing recruitment of PLCy1 3. In the meantime, released chemokines from APCs bind their GPCRs on T cells to activate PLCb 4. PLC will hydrolyze PIP2 into IP3 and DAG a. IP3 binds IP3R and causes calcium efflux b. Decrease of ER calcium stimulates STIM1 oligomerization and formation of CRAC channels, mediating Ca2+ influx 5. Increased cytosolic calcium causes translocation of K+ channels to the PM membrane to cause hyperpolarization 6. Elevated Ca2+ ions due to the SOC entry also activates Ca2+/CaM dependent calcineurin, which dephosphorylates NFAT so it can translocate to the nucleus 7. NFAT translocates to nucleus to initiate gene expressions that induce cell proliferation and phenotypic differentiation of T cells T cell Phenotypic Differentiation - After encountering Ag/TCR, naive T cells are activated to differentiate into distinct T helper subsets, and express unique transcription factors and produce hallmark cytokines - Both the TYPE of antigen interaction, and the cytokine environment is critical for the phenotype development of each Th subset - There are at least 4 distinct sunsets: TH1, TH2, TH17, and iTreg - All have specialized immune functions Severe Combined Immunodeficiency (SCID) - SCID is an autosomal recessive disease caused by mutations in the ORAI or STIM gene, impairing the functions of various cell types - Impaired immune cells: SCID due to CRAC channel dysfunction, characterized by repeated infections from birth, including chronic diarrhea, pneumonia, gastrointestinal infection, sepsis, and inflammation of middle ear - Impaired muscle cells: because SOC entry is critical for muscle cells, SCID patients often show congenital myopathy - muscular dysplasia and hypotonia - Impaired connective tissue cells: SCID patients may show inability to sweat and thus experience recurrent fevers L4: SOCE Dysregulation in Neurological Diseases Forms of SOCCs in Neurons - SOCE facilitates neurogenesis - SOCE through ORAI/TRPC1 channels modulates synaptic formation and plasticity (learning and memory) - SOCE through TRPC1/3 channels underlies slow EPSPs in cerebellar purkinje neurons (motor learning) Cerebellum - The cerebellum is critical for motor learning - During cerebellar development and motor learning, the input signals from mossy fibers drives granule neurons, generating high frequency APs - The high frequency APs induce the purkinje cell and granule neuron growth and plasticity of PN-GN synapses, hence motor learning Ca2+ entry during Slow EPSC - PF-PN synapse: NTs from purkinje fibers bind receptors on purkinje neurons to cause calcium release from the ER, and subsequence calcium influx - STIM1 mediates mGluR-1 dependent synaptic transmission and Ca2+ signaling by forming CRAC channels with TRPC3 Diseases - A point mutation in TRPC3 causes cerebellar ataxia - TRPC3 is highly expressed in purkinje neurons (PNs) during the phase of dendritogenesis - Genetic engineered Mwk Mice got a GOF mutation (Thr635Ala) in the TRPC3 gene, resulting in enhanced TRPC3 channel current, reduced dendritic branches of PNs, and PN death and gait - Increased SOCE to PNS dysregulates gene expression to cause abnormal morphology and function of PNS - Impaired SOCE may be associated with aging related neurodegenerative diseases such as Alzhiemers - Reduced synaptic STIM2 expression, impaired store operated calcium entry, and reduced mature spines in AD model mice