Translation and Genetic Code PDF (2024)
Document Details
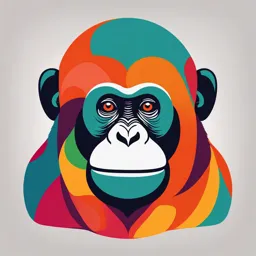
Uploaded by ToughestChlorine
2024
Dr. Madona Akhobadze
Tags
Related
- Lecture 3 Protein Structure, Function and Translation PDF
- Molecular Biology and Cytogenetics - Translation - from mRNA to Protein PDF
- Molecular Biology and Cytogenetics - Translation PDF
- Molecular Biology and Cytogenetics - Translation PDF
- Molecular Biology and Cytogenetics: Translation from mRNA to Protein PDF
- Protein Synthesis Process Guide PDF
Summary
This document presents an overview of translation and the genetic code. It discusses protein structure, polypeptides, and the process of converting genetic information into proteins. The author, Dr. Madona Akhobadze, presents slides on various aspects of protein synthesis and related concepts. The year is 2024.
Full Transcript
Translation and Genetic Code Dr. Madona Akhobadze 2024 Content: One Gene—One Colinear Polypeptide. Protein Synthesis: Translation. The Genetic Code. Codon-tRNA Interactions. Recognition of Codons By tRNAs Readin...
Translation and Genetic Code Dr. Madona Akhobadze 2024 Content: One Gene—One Colinear Polypeptide. Protein Synthesis: Translation. The Genetic Code. Codon-tRNA Interactions. Recognition of Codons By tRNAs Reading: Ch. 12 - Principles of Genetics, by Snustad & Simmons SICKLE-CELL ANEMIA: DEVASTATING EFFECTS OF A SINGLE BASE-PAIR CHANGE James Herrick was the first to publish a description of sickle-cell anemia, the first inherited human disease to be understood at the molecular level. Hemoglobin contains four polypeptides— two alpha-globin chains and two beta-globin chains—and an iron-containing heme group. SICKLE-CELL ANEMIA: DEVASTATING EFFECTS OF A SINGLE BASE-PAIR CHANGE sixth amino acid of the beta-chain of sickle-cell hemoglobin was valine, whereas glutamic acid was present at this position in normal adult human hemoglobin the process by which genetic TRANSLATION information stored in sequences of nucleotides in mRNAs is used to specify the sequences of amino acids in polypeptide gene products takes place in the cytoplasm on complex workbenches called ribosomes and requires the participation of many macromolecules PROTEIN STRUCTURE Proteins are complex macromolecules composed of 20 different amino acids Collectively, the proteins constitute about 15 percent of the wet weight of cells. Water molecules account for 70 percent of the total weight of living cells. With the exception of water, proteins are by far the most prevalent component of living organisms in terms of total mass. Not only are proteins major components in terms of cell mass, but they also play many roles vital to the lives of all cells. POLYPEPTIDES: TWENTY DIFFERENT AMINO ACID SUBUNITS Proteins are composed of polypeptides, and every polypeptide is encoded by a gene. Each polypeptide consists of a long sequence of amino acids linked together by covalent bonds. Twenty different amino acids are present in most proteins. Occasionally, one or more of the amino acids are chemically modified after a polypeptide is synthesized, yielding a novel amino acid in the mature protein POLYPEPTIDES The amino acids differ from each other by the side groups (designated R for Radical) that are present. The highly varied side groups provide the structural diversity of proteins. These side chains are of four types: (1) hydrophobic or nonpolar groups, (2) hydrophilic or polar groups, (3) acidic or negatively charged groups, and (4) basic or positively charged groups The chemical diversity of the side groups of the amino acids is responsible for the enormous structural and functional versatility of proteins. POLYPEPTIDES A peptide is a compound composed of two or more amino acids. Polypeptides are long sequences of amino acids, ranging in length from 51 amino acids in insulin to over 1000 amino acids in the silk protein fibroin The amino acids in polypeptides are covalently joined by linkages called peptide bonds PROTEINS: COMPLEX THREE-DIMENSIONAL STRUCTURES Four different levels of organization—primary, secondary, tertiary, and quaternary—are distinguished in the complex three-dimensional structures of proteins. The primary structure of a polypeptide is its amino acid sequence, which is specified by the nucleotide sequence of a gene. The secondary structure of a polypeptide refers to the spatial interrelationships of the amino acids in segments of the polypeptide. The tertiary structure of a polypeptide refers to its overall folding in three-dimensional space The quaternary structure refers to the association of two or more polypeptides in a multimeric protein protein folding involves interactions with proteins called chaperones that help nascent polypeptides form the proper three-dimensional structure. The two most common types of secondary structure in proteins are alpha-helices and beta-sheets The five types of molecular interactions that determine the tertiary structure, or three-dimensional conformation, of a polypeptide. The disulfide bridge is a covalent bond; all other interactions are noncovalent KEY POINTS Most genes exert their effect(s) on the phenotype of an organism through proteins, which are large macromolecules composed of polypeptides. Each polypeptide is a chainlike polymer assembled from different amino acids. The amino acid sequence of each polypeptide is specified by the nucleotide sequence of a gene. The vast functional diversity of proteins results in part from their complex three-dimensional structures. ONE GENE–ONE COLINEAR POLYPEPTIDE The sequence of nucleotide pairs in a gene specifies a colinear sequence of amino acids in its polypeptide product Most genes encode polypeptides. BEADLE AND TATUM: ONE GENE–ONE ENZYME mutations in genes whose products are involved in the biosynthesis of essential metabolites would be expected to produce mutant strains with additional growth factor requirements Beadle and Tatum tested this prediction by irradiating asexual spores (conidia) of wild-type Neurospora with X rays or ultraviolet light, and screening the clones produced by the mutagenized spores for new growth-factor requirements each mutation resulted in a requirement for one growth factor in several cases one mutation resulted in the loss of one enzyme activity COLINEARITY BETWEEN THE CODING SEQUENCE OF A GENE AND ITS POLYPEPTIDE PRODUCT one gene—one polypeptide relationship the nucleotide pair sequences in genes are colinear with the amino acid sequences of the polypeptides that they encode the presence of introns in genes does not invalidate the concept of colinearity there is no direct correlation in physical distances between the positions of base pairs in a gene and the positions of amino acids in the polypeptide specified by that gene PROTEIN SYNTHESIS: TRANSLATION The genetic information in mRNA molecules is translated into the amino acid sequences of polypeptides according to the specifications of the genetic code. requiring the functions of a large number of macromolecules, including (1) over 50 polypeptides and three to five rRNA molecules present in each ribosome (the exact composition varies from species to species), (2) at least 20 amino acid-activating enzymes, (3) 40 to 60 different tRNA molecules, and (4) numerous soluble proteins involved in polypeptide chain initiation, elongation, and termination OVERVIEW OF PROTEIN SYNTHESIS The first step in gene expression, transcription, involves the transfer of information stored in genes to messenger RNA (mRNA) intermediaries, which carry that information to the sites of polypeptide synthesis in the cytoplasm The second step, translation, involves the transfer of the information in mRNA molecules into the sequences of amino acids in polypeptide gene products Translation occurs on ribosomes, which are complex macromolecular structures located in the cytoplasm TRANSLATION involves three types of RNA, all of which are transcribed from DNA templates (chromosomal genes). In addition to mRNAs, three to five RNA molecules (rRNA molecules) are present as part of the structure of each ribosome, and 40 to 60 small RNA molecules (tRNA molecules) function as adaptors by mediating the incorporation of the proper amino acids into polypeptides in response to specific nucleotide sequences in mRNAs. The amino acids are attached to the correct tRNA molecules by a set of activating enzymes called aminoacyl-tRNA synthetases. COMPONENTS REQUIRED FOR PROTEIN SYNTHESIS: RIBOSOMES About one-third of the total dry mass of most cells consists of molecules that participate directly in the biosynthesis of proteins In prokaryotes, ribosomes are distributed throughout cells; in eukaryotes, they are located in the cytoplasm, frequently on the extensive intracellular membrane network of the endoplasmic reticulum. Ribosomes are approximately half protein and half RNA RIBOSOMES composed of two subunits, one large and one small, which dissociate when the translation of an mRNA molecule is completed and re-associate during the initiation of translation. Each subunit contains a large, folded RNA molecule on which the ribosomal proteins assemble RIBOSOMES Although the size (in Svedberg (S) units) and macromolecular composition of ribosomes vary, the overall three-dimensional structure of the ribosome is basically the same in all organisms. RIBOSOMES E. coli, the small (30S) ribosomal subunit contains a 16S (molecular weight about 6 3 105) RNA molecule plus 21 different polypeptides, and the large (50S) subunit contains two RNA molecules (5S, molecular weight about 4 3 104, and 23S, molecular weight about 1.2 3 106) plus 31 polypeptides. In mammalian ribosomes, the small subunit contains an 18S RNA molecule plus 33 polypeptides, and the large subunit contains three RNA molecules of sizes 5S, 5.8S, and 28S plus 49 polypeptides. The ribosomal RNA molecules, like mRNA molecules, are transcribed from a DNA template. COMPONENTS REQUIRED FOR PROTEIN SYNTHESIS: TRANSFER RNAS direct interactions between the amino acids and the nucleotide triplets or codons in mRNA are unlikely tRNA molecules contain a triplet nucleotide sequence, the anticodon, which is complementary to and base-pairs with the codon sequence in mRNA during translation. There are one to four tRNAs for each of the 20 amino acids. There are three tRNA binding sites on each ribosome The A or aminoacyl site binds the incoming aminoacyl-tRNA, the tRNA carrying the next amino acid to be added to the growing polypeptide chain. The P or peptidyl site binds the tRNA to which the growing polypeptide is attached. The E or exit site binds the departing uncharged tRNA. TRANSLATION: THE SYNTHESIS OF POLYPEPTIDES USING MRNA TEMPLATES The translation of the sequence of nucleotides in an mRNA molecule into the sequence of amino acids in its polypeptide product can be divided into three stages: (1) polypeptide chain initiation, (2) chain elongation, and (3) chain termination. TRANSLATION: POLYPEPTIDE CHAIN INITIATION The initiation of translation includes all events that precede the formation of a peptide bond between the first two amino acids of the new polypeptide chain The synthesis of polypeptides is initiated by a special tRNA, designated tRNAf Met, in response to a translation initiation codon (usually AUG, sometimes GUG). Polypeptide chain initiation begins with the formation of two complexes: (1) one contains initiation factor IF-2 and methionyl - tRNAf Met , and (2) the other contains an mRNA molecule, a 30S ribosomal subunit and initiation factor IF-3 TRANSLATION: POLYPEPTIDE CHAIN INITIATION Prokaryotic mRNAs contain a conserved polypurine tract, consensus AGGAGG, located about seven nucleotides upstream from the AUG initiation codon. This conserved hexamer, called the Shine-Dalgarno sequence, is complementary to a sequence near the 3 terminus of the 16S ribosomal RNA. TRANSLATION: POLYPEPTIDE CHAIN INITIATION The initiation of translation is more complex in eukaryotes, involving several soluble initiation factors (1) The amino group of the methionine on the initiator tRNA is not formylated as in prokaryotes. (2) The initiation complex forms at the 5 terminus of the mRNA, not at the Shine-Dalgarno/AUG translation start site TRANSLATION: POLYPEPTIDE CHAIN INITIATION In eukaryotes, the initiation complex scans the mRNA, starting at the 5’ end, searching for an AUG translation-initiation codon. translation frequently begins at the AUG closest to the 5’ terminus of the mRNA molecule Changes of other bases in the sequence cause smaller decreases in initiation efficiency. These sequence requirements for optimal translation initiation in eukaryotes are called Kozak’s rules, after Marilyn Kozak, who first proposed them. TRANSLATION: POLYPEPTIDE CHAIN ELONGATION The process of polypeptide chain elongation is basically the same in both prokaryotes and eukaryotes. The addition of each amino acid to the growing polypeptide occurs in three steps: (1) binding of an aminoacyl-tRNA to the A site of the ribosome, (2) transfer of the growing polypeptide chain from the tRNA in the P site to the tRNA in the A site by the formation of a new peptide bond, and (3) translocation of the ribosome along the mRNA to position the next codon in the A site In the first step, an aminoacyl-tRNA enters and becomes bound to the A site of the ribosome, with the specificity provided by the mRNA codon in register with the A site The three nucleotides in the anticodon of the incoming aminoacyl-tRNA must pair with the nucleotides of the mRNA codon present at the A site. This step requires elongation factor Tu carrying a molecule of GTP (EF-Tu.GTP) EF-Tu.GDP is inactive and will not bind to aminoacyl-tRNAs. EF-Tu.GDP is converted to the active EF-Tu.GTP form by elongation factor Ts (EF-Ts), which hydrolyzes one molecule of GTP in the process The second step in chain elongation is the formation of a peptide bond between the amino group of the aminoacyl-tRNA in the A site and the carboxyl terminus of the growing polypeptide chain attached to the tRNA in the P site. This key reaction is catalyzed by peptidyl transferase, an enzymatic activity built into the 50S subunit of the ribosome TRANSLATION: POLYPEPTIDE CHAIN ELONGATION During the third step in chain elongation, the peptidyl-tRNA present in the A site of the ribosome is translocated to the P site, and the uncharged tRNA in the P site is translocated to the E site, as the ribosome moves three nucleotides toward the 3 end of the mRNA molecule. The translocation step requires GTP and elongation factor G (EF-G) TRANSLATION: POLYPEPTIDE CHAIN TERMINATION Polypeptide chain elongation undergoes termination when any of three chain - termination codons (UAA, UAG, or UGA) enters the A site on the ribosome These three stop codons are recognized by soluble proteins called release factors (RFs). In E. coli, there are two release factors, RF-1 and RF-2. RF-1 recognizes termination codons UAA and UAG; RF-2 recognizes UAA and UGA. In eukaryotes, a single release factor (eRF) recognizes all three termination codons. TRANSLATION: POLYPEPTIDE CHAIN TERMINATION The presence of a release factor in the A site alters the activity of peptidyl transferase such that it adds a water molecule to the carboxyl terminus of the nascent polypeptide. This reaction releases the polypeptide from the tRNA molecule in the P site and triggers the translocation of the free tRNA to the E site. Termination is completed by the release of the mRNA molecule from the ribosome and the dissociation of the ribosome into its subunits. The ribosomal subunits are then ready to initiate another round of protein synthesis KEY POINTS Genetic information carried in the sequences of nucleotides in mRNA molecules is translated into sequences of amino acids in polypeptide gene products by intricate macromolecular machines called ribosomes. The translation process is complex, requiring the participation of many different RNA and protein molecules. Transfer RNA molecules serve as adaptors, mediating the interaction between amino acids and codons in mRNA. The process of translation involves the initiation, elongation, and termination of polypeptide chains and is governed by the specifications of the genetic code. DECIPHERING THE CODE The cracking of the genetic code in the 1960s took several years and involved intense competition between many different research laboratories (1) Which codons specify each of the 20 amino acids? (2) How many of the 64 possible triplet codons are utilized? (3) How is the code punctuated? (4) Do the codons have the same meaning in viruses, bacteria, plants, and animals? TRANSFER OF GENETIC INFORMATION: THE CENTRAL DOGMA During the replication of RNA viruses, information is also transmitted from RNA to RNA. The transfer of genetic information from DNA to protein involves two steps: (1) transcription, the transfer of the genetic information from DNA to RNA, and (2) translation, the transfer of information from RNA to protein. TRANSFER OF GENETIC INFORMATION: THE CENTRAL DOGMA In addition, genetic information flows from RNA to DNA during the conversion of the genomes of RNA tumor viruses to their DNA proviral forms Thus, the transfer of genetic information from DNA to RNA is sometimes reversible, whereas the transfer of information from RNA to protein is always irreversible. During translation, the sequence of nucleotides in the RNA transcript is converted into the sequence of amino acids in the polypeptide gene product. This conversion is governed by the genetic code, the specification of amino acids by nucleotide triplets called codons in the gene transcript THE GENETIC CODE nonoverlapping code, with each amino acid plus polypeptide initiation and termination specified by RNA codons composed of three nucleotides how the sequence of the four different nucleotides in DNA could control the sequence of the 20 amino acids present in proteins? By the mid-1960s, the genetic code was largely solved PROPERTIES OF THE GENETIC CODE 1. The genetic code is composed of nucleotide triplets. Three nucleotides in mRNA specify one amino acid in the polypeptide product; each codon contains three nucleotides. THREE NUCLEOTIDES PER CODON Twenty different amino acids are incorporated into polypeptides during translation at least 20 different codons must be formed with the four bases available in mRNA THREE NUCLEOTIDES PER CODON In 1961, Francis Crick and colleagues published the first strong evidence in support of a triplet code genetic analysis of mutations induced at the rII locus of bacteriophage T4 by the chemical proflavin Proflavin is a mutagenic agent that causes single base-pair additions and deletions Phage T4 rII mutants are unable to grow in cells of E. coli strain K12, but grow like wild-type phage in cells of E. coli strain B isolated proflavin-induced revertants of a proflavin-induced rII mutation These revertants were shown to result from the occurrence of additional mutations at nearby sites rather than reversion of the original mutation. Second-site mutations that restore the wild-type phenotype in a mutant organism are called suppressor mutations because they cancel, or suppress, the effects of the original mutation. if the original mutation was a single base-pair addition or deletion, then the suppressor mutations must be single base-pair deletions or additions, occurring at a site or sites near the original mutation. AAAGGGCCCTTT can be read (1) AAA, GGG, CCC, TTT, (2) A, AAG, GGC, CCT, TT, or (3) AA, AGG, GCC, CTT, T A single base-pair addition or deletion will alter the reading frame of the gene and mRNA for that portion of the gene distal to the mutation all the isolated mutations grouped into two groups, plus (+) and minus (-) (for additions and deletions), based on the reasoning that a (+) mutation would suppress a (-) mutation, but not another (+) mutation, and vice versa The critical result was that recombinants with three (+) mutations or three (-) mutations often exhibited the wild-type phenotype. This indicated that the addition of three base pairs or the deletion of three base pairs left the distal portion of the gene with the wild-type reading frame. This result would be expected only if each codon contained three nucleotides. (1) Trinucleotides were sufficient to stimulate specific binding of aminoacyl-tRNAs to ribosomes. (2) Chemically synthesized mRNA molecules that contained repeating dinucleotide sequences directed the synthesis of copolymers (large chainlike molecules composed of two different subunits) with alternating amino acid sequences. (3) In contrast, mRNAs with repeating trinucleotide sequences directed the synthesis of a mixture of three homopolymers PROPERTIES OF THE GENETIC CODE 2. The genetic code is nonoverlapping. Each nucleotide in mRNA belongs to just one codon except in rare cases where genes overlap and a nucleotide sequence is read in two different reading frames. PROPERTIES OF THE GENETIC CODE 3. The genetic code is comma-free There are no commas or other forms of punctuation within the coding regions of mRNA molecules. During translation, the codons are read consecutively. PROPERTIES OF THE GENETIC CODE 4. The genetic code is degenerate All but two of the amino acids are specified by more than one codon. A DEGENERATE CODE The occurrence of more than one codon per amino acid is called degeneracy All the amino acids except methionine and tryptophan are specified by more than one. Three amino acids—leucine, serine, and arginine—are each specified by six different codons. Isoleucine has three codons. The other amino acids each have either two or four codons The degeneracy in the genetic code is not at random; instead, it is highly ordered. In most cases, the multiple codons specifying a given amino acid differ by only one base, the third or 3’ base of the codon. A DEGENERATE CODE The degeneracy is primarily of two types (1) Partial degeneracy occurs when the third base may be either of the two pyrimidines (U or C) or, either of the two purines (A or G). With partial degeneracy, changing the third base from a purine to a pyrimidine, or vice versa, will change the amino acid specified by the codon. (2) In the case of complete degeneracy any of the four bases may be present at the third position in the codon, and the codon will still specify the same amino acid. For example, valine is encoded by GUU, GUC, GUA, and GUG PROPERTIES OF THE GENETIC CODE 5. The genetic code is ordered. Multiple codons for a given amino acid and codons for amino acids with similar chemical properties are closely related, usually differing by a single nucleotide. AN ORDERED CODE Scientists have speculated that the order in the genetic code has evolved as a way of minimizing mutational lethality Many base substitutions at the third position of codons do not change the amino acid specified by the codon. amino acids with similar chemical properties (such as leucine, isoleucine, and valine) have codons that differ from each other by only one base. many single base-pair substitutions will result in the substitution of one amino acid for another amino acid with very similar chemical properties (for example, valine for isoleucine). Conservative substitutions of this type will yield active gene products, which minimizes the effects of mutations. PROPERTIES OF THE GENETIC CODE 6. The genetic code contains start and stop codons. Specific codons are used to initiate and to terminate polypeptide chains. INITIATION AND TERMINATION CODONS In both prokaryotes and eukaryotes, the codon AUG is used to initiate polypeptide chains In rare instances, GUG is used as an initiation codon. the initiation codon is recognized by an initiator tRNA, tRNAfMet in prokaryotes and tRNAiMet in eukaryotes INITIATION AND TERMINATION CODONS In prokaryotes, an AUG codon must follow an appropriate nucleotide sequence, the Shine-Delgarno sequence, in the 5’ nontranslated segment of the mRNA molecule in order to serve as translation initiation codon. In eukaryotes, the codon must be the first AUG encountered by the ribosome as it scans from the 5’ end of the mRNA molecule. At internal positions, AUG is recognized by tRNAMet, and GUG is recognized by a valine tRNA. INITIATION AND TERMINATION CODONS Three codons—UAG, UAA, and UGA—specify polypeptide chain termination These codons are recognized by protein release factors, rather than by tRNAs. Prokaryotes contain two release factors, RF-1 and RF-2. RF-1 terminates polypeptides in response to codons UAA and UAG RF-2 causes termination at UAA and UGA codons. Eukaryotes contain a single release factor that recognizes all three termination codons PROPERTIES OF THE GENETIC CODE 7. The genetic code is nearly universal. With minor exceptions, the codons have the same meaning in all living organisms, from viruses to humans. A NEARLY UNIVERSAL CODE Vast quantities of information are now available from in vitro studies, from amino acid replacements due to mutations, and from correlated nucleic acid and polypeptide sequencing, which allow a comparison of the meaning of the 64 codons in different species. These data all indicate that the genetic code is nearly universal; the codons have the same meaning, with minor exceptions, in all species. The most important exceptions to the universality of the code occur in mitochondria of mammals, yeast, and several other species. Mitochondria have their own chromosomes and protein-synthesizing machinery In the mitochondria of humans and other mammals, (1) UGA specifies tryptophan rather than chain termination, (2) AUA is a methionine codon, not an isoleucine codon, and (3) AGA and AGG are chain-termination codons rather than arginine codons. The other 60 codons have the same meaning in mammalian mitochondria as in nuclear mRNAs There are also rare differences in codon meaning in the mitochondria of other species and in nuclear transcripts of some protozoa. However, since these exceptions are rare, the genetic code should be considered nearly universal. KEY POINTS Each of the 20 amino acids in proteins is specified by one or more nucleotide triplets in mRNA. Of the 64 possible triplets, given the four bases in mRNA, 61 specify amino acids and 3 signal chain termination. The code is nonoverlapping, with each nucleotide part of a single codon, degenerate, with most amino acids specified by two or four codons, and ordered, with similar amino acids specified by related codons. The genetic code is nearly universal; with minor exceptions, the 64 triplets have the same meaning in all organisms. გმადლობთ , ყურადღებისთვის !!!