Lymphatic & Immune Systems
Document Details
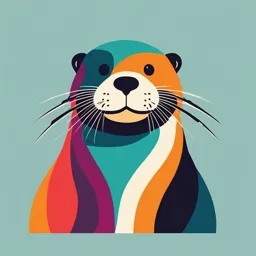
Uploaded by CrediblePiccoloTrumpet
Njala University
Tags
Summary
This document provides an explanation of the lymphatic and immune systems, including the structure of the lymphatic system, and the function of the immune system. It covers topics such as lymphatic vessels, lymph nodes, the thymus, and the spleen. The document details how the lymphatic and immune systems work to protect the body against disease.
Full Transcript
LYMPHATIC & IMMUNE SYSTEMS One hundred trillion microorganisms live on or inside the human body. Some of the millions of microorganisms living inside the body—such as those in the intestines—are necessary for health. Many others, however, can cause disease. If not for the immune system, the body wou...
LYMPHATIC & IMMUNE SYSTEMS One hundred trillion microorganisms live on or inside the human body. Some of the millions of microorganisms living inside the body—such as those in the intestines—are necessary for health. Many others, however, can cause disease. If not for the immune system, the body would be overrun by viruses and bacteria. To illustrate the importance of the immune system, consider that when someone dies, the immune system stops working completely. Within hours, bacteria and parasites invade; within a few weeks, microorganisms can completely consume the body. Although morbid to consider, this illustrates the incredible effectiveness of the immune system. The lymphatic and immune systems work hand-in-hand to protect the body. The immune system basically consists of a population of cells that defend the body against disease. Most of these cells exist within the lymphatic system—a network of organs and vessels that extend throughout the body. Lymphatic System The lymphatic system consists of lymphatic vessels, lymph (the fluid within the vessels), lymphatic tissue, and lymphatic organs. As shown here, the vessels of the lymphatic system cover the body in much the same way as blood vessels. The tissues and organs of the lymphatic system— the lymph nodes, thymus, tonsils, spleen, and red bone marrow—produce immune cells. FAST FACT Lymphatic vessels are found in almost every tissue, except for bone marrow, cartilage, and the central nervous system. Lymph Lymphatic vessels are filled with lymph: a clear, colorless fluid similar to plasma but with a lower protein content. Lymph originates in the tissues as the fluid left behind following capillary exchange. Depending upon its location in the body, lymph may contain lipids (after draining the small intestines), lymphocytes (after leaving the lymph nodes), hormones, bacteria, viruses, and cellular debris. Lymphatic Vessels Similar to veins, lymphatic vessels—also called lymphatic capillaries—have thin walls and valves to prevent backflow. Lymphatic vessel walls are formed by a thin layer of epithelial cells. However, unlike the cells in veins (which are tightly joined), the cells forming lymphatic vessel walls overlap loosely, allowing gaps to exist between the cells. Unlike blood vessels, lymphatic vessels carry fluid in one direction only: away from the tissues. The Body AT WORK The lymphatic system has three functions: the maintenance of fluid balance, the absorption of fat, and immunity. Maintenance of fluid balance: Fluid continually seeps out of capillaries into surrounding tissues. The capillaries reabsorb about 85% of the fluid, leaving about 15% behind. This amount may seem minimal, but, over the course of a day, the remaining fluid would total as much as 4 liters, enough to cause massive swelling and even death. One of the roles of the lymphatic system is to absorb this fluid and return it to the bloodstream. Absorption of fats: Specialized lymphatic vessels in the small intestines absorb fats and fat- soluble vitamins. Immunity: The lymphatic system is a key component of the immune system. Lymph nodes and other lymphatic organs filter lymph (the fluid inside the lymphatic vessels) to remove microorganisms and foreign particles. The Body AT WORK In the cardiovascular system, the heart acts as a pump to help propel blood through the blood vessels. The lymphatic system, however, has no pump. Instead, the fluid moves passively, aided primarily by the rhythmic contractions of the lymphatic vessels themselves. The valves within the vessels prevent backflow. Flow is aided further by the contraction of skeletal muscles, which squeeze the lymphatic vessels. Finally, respiration causes pressure changes that help propel lymph from the abdominal to the thoracic cavity. The Body AT WORK The lymphatic system has two collecting ducts: the right lymphatic duct and the thoracic duct. The right lymphatic duct drains lymph for the upper right quadrant of the body into the right subclavian vein. The thoracic duct (which originates at a dilated portion of a lymphatic vessel in the abdomen called the cisterna chyli) drains lymph from the rest of the body into the left subclavian vein Lymphatic Tissues and Organs Patches of specialized tissue containing lymphocytes exist throughout the body. Passages that open to the outside of the body (such as the respiratory, digestive, urinary, and reproductive tracts) contain a scattering of lymphocytes throughout their mucosa linings. In other parts of the body, lymphatic tissue exists in masses called lymphatic nodules. An example of this is Peyer’s patches, lymphatic nodules residing in the small intestines. Lymphatic organs, on the other hand, are well defined. These organs include red bone marrow, the thymus, lymph nodes, the tonsils, and the spleen. Red bone marrow and the thymus—called primary lymphatic organs—provide a location for B and T lymphocytes to mature. The lymph nodes, tonsils, and spleen—called secondary lymphatic organs—contain lymphocytes that have matured in either the red bone marrow or the thymus. Thymus Located in the mediastinum, the size of the thymus varies with age. Quite large in children, it begins to shrink about age 14. By adulthood, it is a fraction of its former size. The thymus also produces a hormone called thymosin that promote the development of lymphocytes. The thymus is divided into lobules that extend inward from a fibrous outer capsule. Each lobule consists of a dense outer cortex and a less dense medulla filled with T lymphocytes. Lymph Nodes As lymph flows along its course, it passes through multiple lymph nodes. When it reaches a node, the fluid slows to a trickle as the lymph node removes pathogens and other foreign material. Besides cleansing lymph, lymph nodes also serve as sites for final maturation of some types of lymphocytes and monocytes. The body contains hundreds of lymph nodes. Shaped like a bean, some lymph nodes are tiny: only 1/25_ (1 mm) long; others are over an inch (25 mm). FAST FACT Lymph nodes remove 99% of the impurities in lymph before it returns the fluid to the bloodstream. The Body AT WORK Lymph nodes tend to occur in clusters in certain areas. The major lymph node groups are listed below. Cervical lymph nodes, found in the neck, monitor lymph coming from the head and neck. Axillary lymph nodes, clustered in the armpit, receive lymph from the arm and breast. Inguinal lymph nodes occur in the groin; they receive lymph from the legs. Tonsils Masses of lymphoid tissue, the tonsils form a protective circle at the back of the throat. They guard against pathogens entering the body through the nose or throat. There are three sets of tonsils: The Body AT WORK The ring of tonsils at the back of the throat filters air flowing in through the nose and mouth. The white blood cells within the lymphoid tissue of the tonsils can then destroy any viruses or bacteria before they enter the body. Occasionally, the tonsils themselves—particularly the palatine tonsils—become infected by a virus or bacteria. When this happens, the tonsils swell and become inflamed, a condition known as tonsillitis. Symptoms of tonsillitis include a sore throat, painful swallowing, and fever. When caused by a virus, the condition usually resolves on its own after a few days. When it’s caused by a bacteria (usually the streptococcal bacteria that causes strep throat), antibiotics are required. Surgical removal of the tonsils is recommended only when tonsillitis resists treatment or repeatedly recurs. Spleen About the size of a fist, the spleen is the body’s largest lymphatic organ. It resides in the upper left quadrant of the abdomen, just inferior to the diaphragm, where it’s protected by the lower ribs. Just like lymph nodes, the spleen is surrounded by a fibrous capsule; inward extensions of the capsule divide the spleen into compartments. The spleen contains two types of tissue: red pulp and white pulp. The Body AT WORK The spleen fulfills many functions in the body, including those described below. Immunity: Lymphocytes and macrophages in the white pulp screen passing blood for foreign antigens while phagocytic cells in the sinuses ingest and destroy any microorganisms. Destruction of old red blood cells: Macrophages in the sinuses digest worn-out RBCs and imperfect platelets. Macrophages also recycle haemoglobin from the destroyed RBCs, salvaging the iron and globin and returning it to the bone marrow and liver for later use. Blood storage: The spleen stores 20% to 30% of the body’s platelets. Consequently, it can help stabilize blood volume by rapidly adding blood back into general circulation. Haematopoiesis: The spleen produces red blood cells in the foetus. After birth, it does so only in cases of severe anaemia. Throughout life, the spleen provides a location for monocytes and lymphocytes to mature. Overview of the Immune System A person’s survival depends on the body’s ability to protect itself against the hordes of microorganisms—including viruses, bacteria, fungi, and protozoa—that constantly surround us. Other threats from which the body needs protection include toxic chemicals, radiation, and even allergens, like pollen. All the threats aren’t external, however. Some arise from inside the body. For example, abnormal cells often develop as a part of daily life. Left unchecked, these cells will continue to grow and divide, resulting in a tumor or even cancer. The body has three lines of defense for taking care of the threats it encounters on a daily basis. First line of defense External barriers, such as the skin and mucous membranes, keep most of the pathogens we encounter at bay. Second line of defense If a pathogen penetrates the first line of defense, the body launches several mechanisms geared at repelling a wide variety of threats, including the production of phagocytic white blood cells and triggering inflammation and fever. Because these responses are aimed at a broad range of attackers, rather than one specific pathogen, the response is called nonspecific immunity. Another term is innate immunity because the mechanisms are present from birth, allowing the body to repel pathogens to which it has never been exposed. Third line of defense The last line of defense is specific immunity. This occurs when the body retains a memory of a pathogen after defeating it. If exposed to the same pathogen in the future, the body can quickly recognize it, targeting a response at this one specific invader. Nonspecific Immunity Nonspecific immunity protects against a broad range of pathogens, using a variety of mechanisms, such as external barriers, phagocytosis, antimicrobial proteins, natural killer cells, inflammation, and fever. External Barriers The skin and mucous membranes provide the first line of defense against microorganisms. The skin, composed of tough protein, repels most pathogens, while its surface, which is dry and lacking in nutrients, makes a hostile environment for bacteria. Further inhibiting bacterial growth is the acid mantle, a thin layer of acid produced by sweat. The mucous membranes lining the digestive, respiratory, urinary, and reproductive tracts (which are open to the exterior) produce mucus that physically traps pathogens. In the respiratory tract, the mucus is then swallowed, and the pathogens are destroyed by stomach acid. Mucus, tears, and saliva also contain an enzyme called lysozyme, which destroys bacteria. That Makes Sense Remember that specific immunity is aimed at a specific pathogen. For the immune system to recognize that pathogen, it must have been previously “introduced” (or exposed) to that pathogen. (The same thing goes for us: to recognize someone, you need to have been previously introduced.)! Phagocytosis If a pathogen makes its way past the skin or mucous membranes and enters the body, it will immediately confront a key player in the second line of defense: phagocytes. Types of Phagocytes The most important phagocytes are neutrophils and macrophages. While neutrophils roam the body, seeking out bacteria, most macrophages remain fixed within strategic areas. Macrophages evolve from monocytes (another type of phagocytic WBC). Monocytes migrate into connective tissues, where they grow several times larger than their original size and transform into macrophages. (The name macrophage actually means “large eater.”) Macrophages congregate in areas where microbial invasion is likely to occur: the alveolus of the lungs, the liver, nerve tissue, bone, and the spleen. Antimicrobial Proteins Two types of proteins help provide nonspecific resistance against bacterial and viral invasion: interferons and the complement system. Interferons Some cells respond to viral invasion by producing a protein called interferon. When a virus infects a cell, the cell produces interferon, which it releases to nearby cells. The interferon binds to surface receptors on neighbouring cells. This triggers the production of enzymes within the cells that would prevent the virus from replicating if it managed to invade. Complement System Over 20 different proteins (called complement) circulate in the bloodstream in an inactive form, waiting to assist in the immune response. A bacteria, or antibodies against the bacteria, activate the complement. Once a complement reaction begins, it continues as a cascade of chemical reactions, with one complement protein activating the next (similar to what occurs in blood clotting). Complement also aids the immune system by coating pathogens, making them attractive to phagocytes, and stimulating inflammation (which summons neutrophils through chemotaxis). Natural Killer Cells A unique group of lymphocytes called natural killer (NK) cells continually roam the body, seeking out pathogens or diseased cells. They recognize and destroy any foreign cells, including cancer cells, virus-infected cells, and bacteria—as well as the cells of transplanted organs and tissues. The NK cells use several methods to destroy the cells. Most of them involve the secretion of chemicals that causes the cell to die and break apart (lysis). Inflammation Tissue injury, whether from trauma, ischemia, or infection, produces inflammation. Inflammation stimulates the body’s defense system to begin fighting the infection while instigating measures to contain the pathogen. Furthermore, the inflammatory response includes processes that clean up and repair the damaged tissue. Most of the phagocytes (especially the neutrophils) die during the process of fighting the infection. The dead cells pile up, along with tissue debris and fluid, to create a thick yellowish fluid called pus. When pus accumulates in a tissue cavity, it’s called an abscess. The Body AT WORK Redness, swelling, heat, and pain are the four classic signs of inflammation. Swelling results from fluid leaking out of the capillaries. Redness results from hyperemia. Heat also results from hyperemia. Pain may result from injured nerves, pressure on the nerves from swelling, or stimulation of nerves by bacterial toxins. The signs of inflammation also facilitate healing. Swelling compresses veins—reducing venous drainage—while forcing the capillary valves open to promote capillary drainage. This helps healing because lymphatic capillaries are more adept at removing bacteria, dead cells, and tissue debris than are blood capillaries. Hyperemia brings materials necessary for healing, including oxygen and amino acids. Heat in the area increases the metabolic rate, and thus, the rate of tissue repair. Pain signals that an injury has occurred and serves as a reminder to rest the area to allow healing. FAST FACT Words ending in the suffix -itis denote inflammation. For example, dermatitis is an inflammation of the skin, tonsillitis is inflammation of the tonsils, and arthritis is inflammation of the joints. Fever Also known as pyrexia, fever is an abnormal elevation of body temperature. (A person with a fever is said to be febrile.) Experts now believe that fever is beneficial during an illness. Besides promoting the activity of interferon, an elevated body temperature inhibits the reproduction of bacteria and viruses. Following is the normal sequence of events during a fever: 1. As neutrophils and macrophages phagocytize bacteria, they secrete a fever-producing substance called a pyrogen. The pyrogen stimulates the anterior hypothalamus to secrete prostaglandin E (PGE). 2. PGE resets the body’s set point for temperature. For example, it may raise it from a normal of 98.6° F (37° C) to 102° F (39° C). 3. When the set point rises, the body needs to generate heat, which it does through shivering and constricting blood vessels in the skin. The result: chills and cold, clammy skin. 4. The temperature rises until it reaches its new set point, where it remains as long as the pathogen is present. 5. When the pathogen is no longer a threat, the phagocytes stop producing the pyrogen and the body’s set point for temperature returns to normal. When this happens, the body needs to lose the excess heat, which it does through sweating and dilating blood vessels in the skin. The result: warm and flushed skin. Even though most fevers are beneficial, excessively high temperatures are dangerous. Temperatures above 105° F (40.5° C) can cause convulsions; those above 111° F to 115° F (44° C to 46° C) typically result in irreversible brain damage or death. 6. FAST FACT FAST FACT Drugs like aspirin and ibuprofen, often given to reduce fever, act by inhibiting the synthesis of PGE. The Body AT WORK A body temperature of 98.6° F (37° C) is commonly considered to be normal. However, body temperature varies during the course of a day, with different activity levels, and even from person to person. A temperature deemed normal in one person may represent a fever in another. Temperature also varies according to the part of the body being tested: measurements taken inside a body cavity, such as orally or rectally, are higher than those measured on the skin. Specifically, a temperature taken rectally is usually 1° F (0.6° C) higher than an oral reading, whereas a reading taken under the arm (axillary) is 1° F (0.6°C) lower than an oral reading. Specific Immunity In contrast to nonspecific immunity, specific immunity is directed against a specific pathogen. After being exposed to a pathogen, the immune system retains a memory of the encounter. If that particular pathogen enters the body in the future, the immune system will recognize it immediately, allowing it to destroy the pathogen before symptoms even develop. For this third line of defense, the body employs two mechanisms. One aims to destroy foreign cells or host cells that have become infected with a pathogen. This is called cellular (cell mediated) immunity. The other mechanism, which focuses on pathogens outside the host cells, sends out antibodies to “mark” a pathogen for later destruction. This is called humoral (antibody-mediated) immunity. Understanding these two forms of immunity requires an understanding of their weapons: lymphocytes and antibodies. FAST FACT Humoral immunity is so named because antibodies exist in body fluids, and body fluids were once called humors The Body AT WORK The body routinely makes its own antibodies or T cells against a pathogen; this is called active immunity. It’s also possible to achieve immunity after receiving an injection of antibodies from another person or an animal; this is called passive immunity. Active immunity is permanent, or at least long lasting. In passive immunity, the body doesn’t develop a memory for the pathogen, so the immunity lasts only a few months. Following are the four classes of immunity: Natural active immunity: This type of immunity occurs when the body produces antibodies or T cells after being exposed to a particular antigen. (For example, if you become ill with the measles, your body will produce antibodies to this particular virus, making you immune to infection in the future.) Artificial active immunity: This results when the body makes T cells and antibodies against a disease as a result of a vaccination (such as for tetanus or influenza). By injecting a vaccine containing dead or weakened (attenuated) pathogens, the recipient’s body produces an immune response without actually developing the illness. Natural passive immunity: This form of immunity results when a fetus acquires antibodies from the mother through the placenta, or when a baby acquires them through breastfeeding. Artificial passive immunity: This form of immunity involves obtaining serum from a person or animal that has produced antibodies against a certain pathogen and then injecting it into someone else. This is typically used in emergencies for the treatment of rabies and botulism. Lymphocytes The immune system relies on lymphocytes to wage war against invading pathogens. Lymphocytes fall into one of three classes: natural killer cells, T lymphocytes, and B lymphocytes. I. T Lymphocytes Commonly called T cells, T lymphocytes develop from stem cells in red bone marrow. II. B Lymphocytes Commonly called B cells, B lymphocytes also begin life as stem cells in red bone marrow. Unlike T cells, B cells remain in bone marrow until they are fully mature. That Makes Sense To differentiate between T cells and B cells, remember that T cells mature in the Thymus while B cells mature in the Bone marrow. Antibodies Key players in the body’s immune system, antibodies are gamma globulin proteins formed by B cells and found in plasma and body secretions. Also known as immunoglobulins (Ig), antibodies consist of chains of protein joined in a way that resembles a capital letter Y or T. An antigen is any molecule that triggers an immune response. Any foreign substance is said to be antigenic. The immune system learns to distinguish between “self ” and “non-self ” cells before birth so that it attacks only those substances that aren’t part of the body. The Body AT WORK The body manufactures five classes of antibodies: IgA, IgD, IgE, IgG, and IgM. i. IgA: Populates mucous membranes in the intestines, respiratory tract, and urinary tract; also found in saliva, tears, and breast milk ii. IgD: Exists in the blood in very small amounts; may activate basophils and mast cells iii. IgE: Involved in allergic reactions iv. IgG: Is the primary antibody of the secondary immune response; also the most abundant of all the immunoglobulins, making up 80% of all circulating antibodies v. IgM: Active in the primary immune response; also involved in agglutination of incompatible blood types Cellular Immunity Cellular immunity destroys pathogens that exist within a cell. To accomplish this, it employs three classes of T cells: Cytotoxic T cells (also called killer T cells—not to be confused with natural killer cells), which carry out the attack Helper T cells, which play a supportive role Memory T cells, which remember the pathogen in case of future invasion Following the attack, some of the cytotoxic T cells and helper T cells become memory T cells. These numerous, long-lived cells retain a memory of this particular pathogen. If re-exposure to the same antigen occurs, these cells can launch a quick attack. FAST FACT ✓ IgG is the only antibody that can cross the placenta to impart temporary immunity to the fetus. ✓ Because the cellular immune system is programmed to attack any cell not identified with the “self,” it also attacks and tries to destroy transplanted organs and tissues. Humoral Immunity Humoral immunity differs from cellular immunity in that it focuses on pathogens outside the cell. Also, instead of destroying the antigen directly, it uses antibodies to mark them for later destruction. The Body AT WORK Antibodies use a range of tactics to defeat antigens, including: Binding to the antigen’s attachment points, preventing it from attaching to a human cell Triggering agglutination (as in the antigen-antibody reaction), thus helping to contain the antigen and also make it easier for phagocytes to do their work Promoting the binding of complement proteins to the invading cell, thus setting off the complement cascade, which ends with the destruction of the invading microorganism FAST FACT The human immune system can produce billions of different antibodies, each of which responds to a different antigen. The Body AT WORK When the body is exposed to a particular antigen for the first time, the immune system must complete a number of different tasks before it can attack the invading antigens. For example, it must create B cells that, in turn, must differentiate into plasma cells. Once that is completed, the plasma cells can begin producing antibodies. This initial reaction is called the primary response. The amount of time between exposure to a new antigen and a rising level of blood antibodies against that antigen (called the antibody titer) ranges from three to six days. Once the attack is over and the antigen has been removed from the body, memory B cells remain. Even though the number of antibodies decline, if the same antigen should invade again, the memory B cells can immediately begin to divide. This allows for rapid production of memory and plasma cells, which can then create large numbers of antibodies against the antigen. This response, called the secondary response, takes only hours as opposed to days. Consequently, the body can attack the antigen before it has time to produce symptoms.