Urine Concentration and Dilution (G29) PDF
Document Details
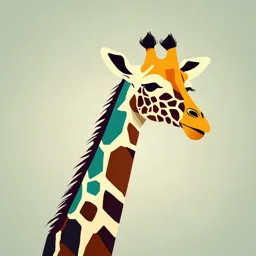
Uploaded by VerifiablePrologue3527
Tags
Summary
This document covers the mechanisms of urine concentration and dilution, focusing on the processes involved in the kidneys. It details the role of various structures and systems, outlining the interplay between blood flow, solutes, and water in maintaining homeostasis.
Full Transcript
urea excretion is determined by these three things. The concentration of urea in the plasma, the glomerular filtration rate, and the renal tubular reabsorption. Patients with renal disease have large reductions in GFR, which increases the concentration of urea in the plasma. This then causes a highe...
urea excretion is determined by these three things. The concentration of urea in the plasma, the glomerular filtration rate, and the renal tubular reabsorption. Patients with renal disease have large reductions in GFR, which increases the concentration of urea in the plasma. This then causes a higher rate to be filtered, causing the urea urea to be excreted. Despite the decreased GFR in the proximal tubule, 40 to 50% of the filtered urea is reabsorbed, although concentration in the tubular fluid remains high because urea diffuses far less than water at the same time. There are some secretion of urea into the tubular fluid in the thin loop of hennelly, and that is facilitated by the UT A2 transporter, and that is in combination. And you see this here. And of course, that's in combination with the UT, A1, UT three and uh, 80 H that we talked about on the last slide. Blood flows provided to the renal modular to supply the metabolic needs of the cells. But without a special system, this blood flow would diminish the activity of the counter current multiplier system. Therefore, there are two special features for renal medullary blood flow that are listed here. The blood flow is low, accounting for less than 5% of total total renal blood flow, and the phase of Recta serves as a counter current exchanger, minimizing the washout of solutes from the interstitial. Uh, we're going to go over that now. Blood enters and leaves the medulla via the vas recta, which is highly permeable to solutes in the blood except for plasma proteins, just like other capillaries. As the blood descends into the medulla, it becomes progressively more concentrated, partially by solutes entering and partially by the loss of water into the interstitium. By the time the blood leak, uh, reaches the tips of the base of recta, it has a concentration of 1200 milli osmosis, the same as the interstitium. As blood ascends back out of the medulla interstitium. Water moves into the capillaries, and it becomes more dilute because of this U-shape. And how the concentration in the vasa rectum mirrors the concentration of the nephron. The vas erected does not prevent the medulla osmolarity from being dissipated. The summary of changes in osmolarity and urine volume are shown in this chart. We go through the individual segments in the following slides. About 65% of most filtered electrolytes are reabsorbed in the proximal tubule. However, it is also highly permeable to water. So whenever solutes are reabsorbed, water also diffuses, and therefore the osmolarity, the fluid remains about the same as the plasma infiltrate. And you can see that here that has been circled. Um, and also here in this segment. As fluid flows down the ascending loop of Henley, water is reabsorbed into the medulla. Aquaporins one. Channels are present in this limb and it is highly permeable to water, less so to sodium and chloride. Therefore, the osmolarity gradually increases until it is around 1200 milli osmosis, and you can see that here as the osmolarity increases. Not quite a straight line, but close. Because sodium was concentrated when the water was removed in the descending limb. Passive reabsorption of sodium dilutes the fluid remaining in the tubule. The ascending limb is essentially impermeable to water but reabsorb sodium. You can see the circled segments here. The thick ascending loop of Henley is also impermeable to water, but large amounts of sodium, chloride, potassium, and other ions are actively transported out of the tubule and into the interstitial. Therefore, the fluid becomes very dilute, falling to a concentration of around 140 million moles. The early distal tubule has properties similar to the thick ascending loop. So the tubular fluid is further diluted to about 100 million moles. In the late distal tubule and cortical collecting tubules. The osmolarity of the fluid depends on the level of ADHD. With high levels, these tubes are highly permeable to water and significant amount of water is reabsorbed. Since urea cannot pass through these tubules, it results in increased concentration. In the absence of ADH, little water is reabsorbed and active reabsorption of solutes continues, further diluting the tubular fluid. The concentration of fluid in the intermediary collecting ducts depends upon ADHD and the surrounding medullary interstitium osmolarity that is established by the counter current mechanism in the presence of ADHD. Ducks are highly permeable to water, which diffuses from the tubule into the interstitium until osmotic equilibrium is reached. Since the inner medullary collecting ducts have specific urea transporters that facilitate diffusion, when urea is concentrated, it diffuses out of the tubule lumen into the interstitium, contributing to the osmolarity of the interstitial. Two important points are that the kidney can can, when needed, excrete a highly concentrated urine that contains little sodium. And secondly, large quantities of dilute urine can be excreted without excreting sodium. Lastly, there is an obligatory urine volume that is dictated by the concentrating ability of the kidneys and the amount of solutes that must be excreted. For example, if 600 million osmosis solutes must be excreted each day, this requires 2.5l of urine. Since the maximum urine concentrating ability is 1200 milliliters moles per liter. The regulation of extracellular fluid osmolarity and sodium concentration are closely linked because sodium is the most abundant extracellular ion. Sodium is normally regulated closely between 104 and 145 MQ per liter, while the osmolarity of plasma averages 300 million moles per liter. Osmolarity determines the distribution of fluid between the intracellular and extracellular compartments, and typically does not vary more than 2 to 3%. Sodium and its associated anions accounts for 94% of the solutes in the extracellular plasma, with glucose and urea accounting for the other 3 to 5%. But since urea usually permeates most cell membranes, it exerts little osmotic pressure. Molarity can roughly be estimated from plasma sodium concentration. Sodium ions in the extracellular fluid and its associated anions are the principal determinants of fluid movement across the cell membranes. Therefore, the control of sodium ion concentration and osmolarity are synonymous and can be discussed simultaneously. The two primary systems, especially involved in regulating the concentration of sodium and osmolarity of extracellular fluid are the osmo receptor system and the thirst mechanism. When osmolarity increases above normal because of water deficit, the Osmo receptor and feedback system works this way. Increases in extracellular fluid osmolarity or sodium concentration, causes osmo receptor cells in the anterior hypothalamus to shrink, which causes them to send nerve signals to additional cells in the super optic nuclei, which then sends messages down the stock to the posterior pituitary. Stimulation to the posterior pituitary causes ADHD be released from vessels in nerve endings, and enters the bloodstream and is transported to the kidneys, where it stimulates the insertion of aquaporins into the late distal tubule, cortical collecting ducts, and medullary collecting ducts. The increased water permeability causes increased water reabsorption and the excretion of small volume of concentrated urine, which causes the excretion of sodium and other solutes while conserving water, uh, correcting the high osmolarity. The opposite sequence occurs when, uh, fluid osmolarity becomes to dilute. Uh, when osmolarity is low, less ADH is formed. Water permeability is decreased in the renal tubules and less water is reabsorbed. 88 is synthesized in the super optic and pair ventricular nuclei of the hypothalamus. Both these nuclei have axonal extensions into the posterior pituitary. Uh. 80 age is synthesized and then transported down these axons to their tips in the posterior pituitary gland. When the super optic and of ventricular nuclei are stimulated by increased osmolarity, nerve impulses pass down these nerve endings, changing their permeability and allowing calcium entry. Calcium enters the nerve. Uh. Causing secretary vesicles to release ADHD. ADHD then carried away in the capillary blood to the uh, of the posterior pituitary and into the circulation. Uh. This secretion is rapid. ADHD levels can increase several fold within minutes. In addition to osmolarity causing 88 secretion releases, also stimulated by the cardiovascular reflexes that respond to decreases in blood pressure and or blood volume. This includes arterial barrier receptor reflexes and cardiopulmonary reflexes. These reflex pathways are in high and low pressure regions of the circulation. Afferent stimuli are then carried by the vagus and also pharyngeal nerves, which are then relayed to the hypothalamic nuclei. Aid release is considerably more sensitive to small changes in osmolarity than to similar percentage changes in blood volume. Plasma osmolarity changes of only a few percent are sufficient to increase ADHD levels, but ADHD levels do not change that much until blood volume is reduced by about 10%. Therefore, the daily regulation of Ada secretion during simple dehydration is affected mainly by changes in plasma osmolarity. There are some things that can cause Ada secretion. Uh, some other things that can cause Ada secretion also. And they're shown on that table here. Fluid loss is minimized by the Osmo receptor feedback system. Adequate intake, however, is necessary to counterbalance any losses that do occur through breathing, sweating, or the GI tract. This fluid intake is regulated by thirst mechanisms. Many of the same factors that stimulate Ach release also increase thirst. The thirst center is located in an anterior ventral wall, the third ventricle, and other regions, uh, and it functions as Osmo receptors in the same way that the Osmo receptors do that stimulate ADH release. The most important factors to stimulate thirst is increased extracellular fluid osmolarity. This of course causes intracellular dehydration in the thirst centers. Decreases in extracellular fluid volume and arterial pressure can also stimulate thirst by independent pathways. Another important stimuli for thirst is angiotensin two. Angiotensin two acts upon regions outside the blood brain barrier. Angiotensin two is stimulated by the factors associated with hypokalemia and low blood pressure, so its stimulation on the thirst center helps restore blood volume and pressure. Mouth and mucous membrane dryness can, uh, elicit the thirst sensation as well. And also gastrointestinal. Uh. And pharyngeal stimulation. The kidneys must continually excrete an obligatory amount of water. Even in a dehydrated person, to rid the body of excess solutes that are ingested or produced by metabolism. Water is also continually lost to evaporation due to breathing and from sweating, as well as the GI tract. The threshold for drinking is only about two emic per liter above normal osmolarity. This is when the thirst mechanism is activated, causing the desire to drink. Only. A small change in osmolarity causes a desire to drink, which of course restores osmolarity to normal. In this way, cellular fluid osmolarity is precisely controlled and healthy person. The Osmo receptor eight and thirst mechanisms work in parallel to regulate this, even with sodium intake as high as six times normal. This is only a small effect on plasma sodium concentration, as long as the aid and thirst mechanisms are functioning normally. That is what is shown in the graph here. The red line shows increased sodium intake. Uh, but cellular concentration remains the same. Um, here. And you can see increased amount of sodium intake. Uh, but plasma sodium concentration remains the same. Um. The blue line shows the effect of ADHD. If the ADHD systems are blocked, the ADHD and thirst mechanisms are blocked here with the blue line and you can see the plasma concentration increasing greatly with increased sodium intake. Even if one of the systems fail, the other can still control osmolarity with reasonable effectiveness. As long as there's enough fluid intake to balance obligatory urine volume. However, if both ADHD and thirst mechanisms fail simultaneously, sodium concentration and osmolarity will be unable to be controlled. No other feedback mechanism is capable of adequately regulating plasma sodium concentration and osmolarity.