Renal Tubular Reabsorption and Secretion PDF
Document Details
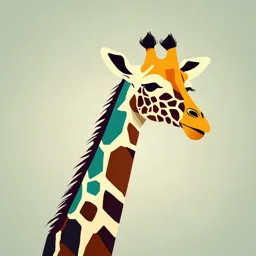
Uploaded by VerifiablePrologue3527
Tags
Summary
This document discusses renal tubular reabsorption and secretion, focusing on the mechanisms of transport for various substances across the renal tubule. It covers active and passive transport, including examples of specific molecules like glucose and amino acids, and the role of different pressures in this process.
Full Transcript
In some parts of the tubule, especially the proximal tubule. Reabsorption of large molecules such as proteins, occurs via pino ptosis. In the tubular lumen, the protein attaches to the brush border, the brush border, then endogenous, and encapsulate the protein, forming a vessel. Once inside the cel...
In some parts of the tubule, especially the proximal tubule. Reabsorption of large molecules such as proteins, occurs via pino ptosis. In the tubular lumen, the protein attaches to the brush border, the brush border, then endogenous, and encapsulate the protein, forming a vessel. Once inside the cell, the protein is digested into its constituent amino acids, which are then reabsorbed through the basal lateral membrane. Since Pino said ptosis requires energy, it is considered active transport. I'm sure you remember this slide from chapter two. The filtration is non-selective. That is, if a substance is not bound to protein, it is filtered. Ethical Mary lists the amount dependent upon its concentration in the plasma. There is a large amount of glomerular filtration as compared to urine. Therefore, there is a high amount of tubule reabsorption as compared to urinary excretion. This means that a small change in glomerular filtration or tubular reabsorption can cause a large change in urinary excretion. We discussed this before when we discuss glomerular filtration. The same principle applies to tubular reabsorption. For example, a 10% decrease in tubular reabsorption would increase urinary volume from 1.5 to 19.3 liters a day. This tubular reabsorption is highly selective. Some substances, such as glucose and amino acids, are reabsorbed almost completely, whereas others, such as sodium and chloride, are variable depending on the needs of the body. And lastly, in the last category, waste products such as urea are poorly reabsorbed and excreted in large amounts. The mechanism for reabsorption can be controlled independently of each other, allowing the selective reabsorption or secretion of individual substances. In the image, you can see different paths for reabsorption from the tubular lumen, across the tubular epithelial cells, through the renal interstitium, and back into the blood. Now we can start to talk about those mechanisms. More specifically, to be reabsorbed, substances must first be transported across the tubular epithelial membrane into the renal interstitial fluid, and then through the peer to peer capillary membrane back into the blood. This can involve active or passive transport. So they must go from. The, uh, tubular lumen, uh, where you have filtrate and it must go through either the trans cellular or the cellular pathway, uh, through the brush border into the tubular cell and then transported out of the tube or cell into the. Interstitial fluid and then through pressures into the blood. Um. These solutes can pass through the cell membrane or through the spaces between the cell junctions. And so this is the cellular pathway that in between the cell junctions um passes through the tubular capillary walls. And the blood is mediated by hydrostatic and colloid osmotic pressures similar to venous pressures. Uh venous reabsorption we have discussed before. So that's where this occurs is the venous reabsorption. This picture on the bottom left gives a little better visual representation of the spaces. It shows of course the tubular lumen the tubular epithelial cells the basement membrane the interstitial fluid which is important. It's not represented here. It just shows the bulk flow here in this big image. But you can see the interstitial fluid here. And it kind of makes a better visual representation. Um, and then of course the period tubular capillary here. Uh, so the transport of solutes here is mediated by these pressures that we've discussed before. Active transport moves of solute against an electrochemical gradient and requires energy. An example of this is a sodium potassium ATPase pump. You can see an image of the sodium potassium ATP pump here. Energy is needed to transport sodium potassium against its electrochemical gradient. So here's the sodium potassium ATPase pump. You're transporting sodium out of the cell, you know, against its electrochemical gradient and potassium into the cell against its electrochemical gradient. Um, secondary active transport is coupled indirectly to an energy source. For example, here with the glucose, um, it's coupled via a ion gradient. Um, the sodium potassium part we just discussed creates a low, um intracellular concentration of sodium. So by pumping the sodium out, a low concentration of sodium is intracellular. We've talked about that before. Um, this causes sodium to diffuse down its electrochemical gradient from the tubular into the cell. Um. It brings glucose with it. The transporter brings glucose with it from the tubule. So therefore the glucose is, uh, transported intracellular via secondary active transport. The same goes here with amino acids. Epithelial cells are held together by tight junctions. But behind these tight junctions by a pair of cellular pathway solutes can be reabsorbed through the trans cellular pathway, through the tubular cells or between the cells by moving across the tight junctions and then through the intracellular spaces through the pair of cellular pathways. So the visual representation pair cellular pathway in the trans cellular pathway, uh, for example, sodium moves through both routes, mostly through the trans cellular pathway, although in the proximal tubule water is reabsorbed across the of cellular pathway. Primary active transport moves solutes against an electrochemical gradient. For example, here with this ATPase pump across the proximal tubular membrane on the basal lateral side of the tubular epithelial cell. The cell membrane has an extensive system of the sodium potassium pumps that use energy from the ATP, from ATP, to transport sodium ions out of the cell and into the interstitium this creates. Low intracellular sodium and high intracellular potassium. Uh, creating a negative charge of -70 millivolts, uh, within the cell and a sodium concentration of around 12. I'm just going to write it up here. So it's separate 12 MCU. Intracellular as compared to 140 MQ. In the tubular lumen. Um, this creates a strong concentration gradient. Uh. From the tubular lumen into the cell. So this creates a strong drive, uh, strong concentration gradient for movement from tubular women to tubular epithelial cells. Uh, at the same time, this electrochemical, uh, electrical. Gradient drives sodium into the cell as well. The active reabsorption of sodium by the sodium potassium pump occurs in most parts of the tubule. Certain parts, uh, of the tubule. There are even more provisions for sodium reabsorption into the cell. For example, in the proximal tubule there's an extensive brush border. And you can see the brush border illustrated here. Um, on the luminal side of the tubular epithelial cells that multiplies the surface area for reabsorption by about 20 fold. Uh, in some areas, there's also carrier proteins that can provide facilitated diffusion of sodium. And we'll talk more about that. In summary, there are three steps for the net reabsorption of sodium from the tubular lumen into the blood. One sodium diffuses from the tubular lumen across the apical membrane down and electrochemical gradient established by the sodium potassium ATPase pump. Two sodium is transported across the basal outer membrane against an electrochemical gradient by the sodium potassium ATPase pump. Three sodium water and other substances are reabsorbed from the interstitial fluid into the paired tubule capillaries by a passive process driven by hydrostatic and colloid osmotic pressure gradients. In secondary active transport, two or more substances interact with a specific carrier molecule or membrane protein and are transported across a membrane. One of the substances in this illustration, sodium uh, diffuses down its electrochemical gradient, providing the energy to drive another substance against its electrochemical gradient. Therefore energy is not required directly from ATP. In this image, sodium is transported from the tubular lumen down its concentration gradient. Um. Which provides for the secondary active transport of glucose and amino acids. Uh, in the proximal tubule. This requires a specific carrier protein in the brush border that combines with a sodium ion and an amino acid or glucose molecule. At the same time, after entering the tubular cell, the glucose and amino acids exit the basal basal membrane by diffusion, driven by their high interest concentration. The sodium and glucose cotransporter in the brush border are referred to as Sgt one and two transporters. 90% of the filtered glucose is reabsorbed by GT two transporters and the other 10% by GLP one uh. Later, in the proximal tubule on the basal lateral side, glucose diffuses out of the cell with the help of a glucose transporter. Uh, here. Or it's referred to as glute one and glute to their transport on the basal lateral side does not directly use ATP. ATP, although is dependent upon energy expended by the sodium potassium pump maintaining the electrochemical gradient. Thus glucose is secondary active transport because the glucose is reabsorbed against its concentration gradient. Here. Uh, but the energy it uses is secondary to the primary active transport of sodium. Any time a step in the process is through secondary active transport, the entire process is referred to as secondary active transport. For example, the glucose reabsorption at the luminal membrane is down here. This at the luminal membrane is down. Um. Its concentration gradient, but it is dependent upon the secondary active transport from the tubular lumen. Therefore, both steps are referred to as secondary active transport. So both these steps, even though this one is down its concentration gradient, is referred to a secondary active transport. Some substances are secreted into the tubules by secondary active transport, which often involves counter transport of the substances with sodium ions. Energy is created by the downhill movement of one substance. In this image. Sodium. So here, uh, which enables the uphill movement of a secondary substance in the opposite direction here with hydrogen. Uh, the example here is the counter transport and act of secretion of hydrogen ions that is coupled to sodium reabsorption. This is mediated by the sodium hydrogen exchanger protein. In the luminal membrane of the proximal tubule, a sodium ion is transported to the interior of the cell. Why? While hydrogen ions are forced outward into the tubular lumen. For most substances that are actively reabsorbed. There is a limit to the rate at which this reabsorption can occur. This limit is due to the saturation of the specific transport systems. When the tubular load exceeds the capacity of the carrier proteins and specific enzymes involved. Glucose reabsorption is a good example. When the filtered glucose exceeds the capacity of the tubules to reabsorb it. Urinary excretion occurs. The transport maximum for glucose is about 375mg a minute, whereas the typical amount of filtered glucose is only 125mg a minute. But with large increases in GFR or plasma glucose concentration, the amount of glucose in the tubule can exceed this 375mg a minute. You can see on the graph that as the filtered load increases, transport increases. So here you, uh, filtered and transport together. Um. Until it becomes close to the plateau, which is, uh, the transport maximum, so that here you have the plateau, uh, the transport maximum. Um, glucose appears in the urine slightly before the transport maximum was reached. Since not all nephrons have the same transport maximum and some excreted glucose occurs before others, some glucose excretion occurs before others in certain nephrons. Of course, when filtered load as above. Reabsorption limits, uh, the rest will be secreted. This is shown with the green excretion line. So you can see the excretion increases in the urine as you reach, uh, the transport maximum. Um, as the filtered load increases. Some substances that are actively reabsorbed do not demonstrate a transport maximum. This occurs when the rate of transport is determined by other factors such as the electrochemical gradient, the permeability of the membrane for the substance, or the time that the substance remains within the tubule. This, of course, depends on the tubular flow rate. For example, in the proximal tubule, the reabsorption of sodium is usually far greater than the actual rate of sodium reabsorption, because sodium leaks back into the tubular lumen through loose junctions. Therefore, sodium transport in the proximal tubules obeys mainly gradient time transportation principles rather than transport maximum rates. The higher the concentration of sodium in the tubule lumen, the more reabsorption takes place. So, uh, sodium leaks back through. Uh, the Epatha junctions are back in the tubule lumen. So the higher the concentration here in the tubular lumen, the less will leak back, because it is opposed by this concentration gradient, uh, in more distal parts of the nephron. The epithelial cells have a much tighter junction. And these areas exhibit transport maximum because it's dependent upon this mechanism. Transport maximums can also be influenced by hormones such as aldosterone. When solutes are transported out of the tubule by any mechanism, their concentrations inside the tubule will decrease, while at the same time, concentrations inside the renal interstitium will increase. This creates a concentration difference that causes osmosis in the same direction. The proximal tubule is highly permeable to water, and water reabsorption occurs so rapidly that there's only a small concentration gradient for solutes across the tubular membrane. A large part of this water flow occurs through aquaporins in the cell membranes, as well as tight junctions between epithelial cells. In more distal parts of the nephron. Beginning in the loop of Hennelly, the tight junctions become far less permeable to water so that water can not move as easily. However, antidiuretic hormone or ADHD greatly increases the water permeability in the distal and collecting tubules. So in summary, the in the proximal tubule and the descending loop of Hindley. Water permeability is always high due to the bonded expression of aqua and one water channels in the ascending loop of Hindley, water permeability is always low, so there's almost no water reabsorption. And in the distal tubules, collecting tubules and collecting ducts. Water permeability is caused by aquaporins that are dependent upon the presence of ADHD. When sodium is reabsorbed from the tubular lumen. Negative ions such as chloride are transported along with the sodium. Because of electrical potentials, sodium movement leaves the inside of the lumen negatively charged, causing chloride to diffuse passively through the cellular pathway. As sodium and water are reabsorbed from the tubular lumen, the chloride ions are concentrated, causing a concentrated gradient to develop in passive diffusion to occur. Thus, the active reabsorption of sodium is closely coupled to the passive reabsorption of chloride caused by the electrical potential and the chloride concentration gradient. Chloride ions are also reabsorbed by secondary active transport across the luminal membrane. Urea is also passively reabsorbed from the tubule, but to a much lesser extent than chloride as water is reabsorbed from the tubules. Concentration increases and a concentration gradient gradient favoring reabsorption occurs. However, the tubule is not easily permeable to urea, so that only about half of the filtered urea is reabsorbed by the tubules. The remaining 50% passes into the urine, allowing for large amounts to be excreted. Another waste product of metabolism is creatinine. The tubular membrane is essentially impermeable to it because it is a large molecule, and therefore none of the creating that is filtered is reabsorbed, and virtually all is excreted in the urine. 65% of the filtered sodium and water are reabsorbed in the proximal tubule. This high capacity results from its special cellular characteristics. The proximal tubule has a large number of mitochondria to provide energy and support powerful active transport processes. It also has a large brush border on the luminal side of the membrane, and extensive channels on the basal or side that together provide an extensive surface area on both sides of the epithelial cell for rapid transport of sodium and other substances. There is extensive protein carrier molecules for Co transport mechanisms of sodium, along with other organic nutrients, as well as counter transport mechanisms for the secretion of hydrogen ions. The sodium potassium pump provides the major force for reabsorption of sodium chloride and water. Much of the glucose, amino acids, and other solutes are absorbed by Co transportation during the first half of the proximal tubule. Higher and higher concentrations of chloride in the second half are then absorbed. The amount of sodium in the tubular fluid decreases. The concentration remains the same because water permeability is so great. Water is absorbed at the same rate as sodium. Glucose, amino acids and bicarbonate are avidly reabsorbed, and their concentrations decrease markedly in the proximal tubule. Solutes such as creatinine increase in concentration, making the osmolarity remain the same. Organic acids and bases such as bile salts, oxalate, urate, and catecholamines are also excreted uh in the proximal tubule. In addition to the waste products of metabolism, harmful drugs and toxins are excreted in tubules as well as para amino acid. Uh para amino hipp uric acid is cleared so rapidly that it can be used to estimate renal plasma flow. The loop of Henley consists of three functionally different segments the thin descending, the thin ascending, and the thick ascending. The thin descending and thin ascending have thin epithelial membranes with no brush border. Few mitochondria and low levels of metabolic activity. The function of the thin descending is to allow for simple diffusion. It is highly permeable to water and moderately permeable, permeable to most solids. 20% of the filtered water is reabsorbed in the loop of Henley. Almost all of it in the thin descending limb. Both portions of the ascending limb are impermeable to water, which is important for concentrating urine. The thick epithelial cells of the ascending limb have high metabolic activity and are capable of active sodium chloride and potassium reabsorption. An important component for reabsorption in the thick of sending limb is a sodium potassium ATP pump in the epithelial cell basal outer membrane. So this guy here. The reabsorption of solutes in the segment is closely linked to the capability of this pump to maintain the low intracellular sodium concentration. So low sodium intracellular. Um. Which of course, this provides the favorable gradient for the movement of sodium from the tubular fluid into the cell. Uh, this this provides the gradient for the secondary active transport of the one sodium two chloride one potassium transporter in the thick ascending. So this person, this, uh, transporter. There's also a sodium hydrogen counter transporter for sodium reabsorption and hydrogen secretion. The reabsorption of magnesium, calcium, potassium, and more. Sodium is caused by the slightly positive charge of the tubular lumen. So you see here the positive charge of the tubular and driving positively charged. Um. Salutes through and out. Uh, the thick segment of the ascending loop is virtually impermeable to water. And therefore, by absorbing these solutes, uh, the water becomes very dilute. This is important for diluting and concentrating properties of the kidney. This decisive action for loop diuretics, which inhibit the sodium potassium two chloride transporter. So you can see on the slide here it says Lasix, um, is inhibiting this transporter. First portion of the distal tubule forms the macula denser, that is part of the juxtaposed Mary-Lou complex, which provides feedback and control of GFR and blood flow. Uh, that we've talked about before. The distal tubule was referred to as the diluting segment because it also absorbed sodium potassium chloride, but is virtually impermeable to water and urea. The thighs I diabetics inhibit the sodium chloride cotransporter here. The second half of the distal tubule and cortical collecting ducts has similar functional characteristics. They're composed of principal cells which reabsorb sodium and water and secrete potassium, and intercalated cells, which reabsorb potassium and secrete hydrogen into the tubular lumen. We'll cover those cells more thoroughly. Coming up. The principal cells perform sodium reabsorption and potassium secretion that is dependent upon the activity of the sodium potassium pump in the basal lateral membrane. So once again, the sodium potassium pump in the basal latter membrane uh. This causes potassium to be pumped into the cell. Uh, and it maintains a high intracellular potassium concentration. This potassium then diffuses down its concentration gradient into the tubular lumen. Is the primary site of action of potassium sparing diuretics and sodium channel blockers. The sodium channel blockers inhibit the entry of sodium into the tubular cells. Uh, here, as you can see with the sign, um. Which reduces the activity of the sodium potassium pump that then decreases the transport of potassium into the cell. It reduces the secretion of potassium into the tubular fluid. The intercalated cells make up 30 to 40% of the cells in the collecting tubules and collecting ducks. They play a major role in acid base regulation. There are two types. Type A secretes hydrogen using a hydrogen ATP pump and hydrogen potassium ATP transporter. Type B has the opposite function of the type A, uh. Type B secretes bicarbonate ions into the tubule lumen and reabsorb hydrogen. Type A is to correct acidosis. Type B is to correct alkalosis. Both types of cells utilize carbonic anhydrase to make hydrogen and bicarbonate intracellular. The chloride bicarbonate powder transporter on the apical membrane is called pinion. So this pinion transporter comes up later when chronic metabolic alkalosis is present. The number of type B intercalated cells increases when there's chronic acidosis. Type A cells increase. The functional summary of the late distal tubule and cortical collecting tubule. Uh, are these things? Um, one it's impermeable to urea. Both portions reabsorb sodium, primarily controlled by aldosterone, and also secrete potassium. Type A, intercalated cells actively secrete hydrogen, and type B intercalated cells secrete bicarbonate. The permeability of the late tubule and cortical collecting ducts to water is controlled by 80 or vasopressin. The Magellanic collecting ducts reabsorb less than 5% of the filtered water and sodium. But they are the final site for processing urine and therefore play critical role in determining the final output of water and solids. The cells are nearly cuboid, all in shape, with smooth surfaces that lack the brush border, while they also have very few mitochondria. Permeability of water is controlled by antidiuretic hormone with high levels. Water's water is avidly reabsorbed, which of course reduces urine volume and concentrates it. There are special urea transporters to facilitate diffusion, tubular cells, and eventually the paired tubular capillary. This raises the osmolality and increases the kidney's ability to form concentrated urine. Lastly, the Magellanic collecting duct secretes hydrogen ions against a large concentration gradient. Whether solutes become concentrated is determined by the relative degree of reabsorption of that solute versus the reabsorption of water. The changes in concentration are represented in this graph. As the filtrate moves along the tubular system. Concentrations rise higher if more water is reabsorbed than solute, or fall. If more solute is reabsorbed than water, you can see substances such as creatinine and urea become highly concentrated in the urine. So Korea and Korea you can see their concentrations raise. These substances are not needed by the body, and the kidneys have become adapted to minimally reabsorb them or even secrete them. Other substances, such as glucose and amino acids at the bottom. Uh, obviously glucose and amino acids here. Um. Are strongly reabsorbed because they are needed by the body. You can see the substances inulin in the graph as well. So there's inulin. Um, inulin is used to measure GFR. It is not reabsorbed or secreted by renal tubules. So changes in concentration reflect changes in water reabsorption at the end of the proximal tubule. You can see the inulin ratios around three proximal tubule in. So ratios around three. Uh, this means that the concentration is three times greater than when it was filtered, meaning only one third of the filtered water remains and two third has two thirds has been reabsorbed. It is essential to maintain a precise balance between tubular absorption and glomerular filtration. There are multiple nervous, hormonal, and local control mechanisms that regulate this. An important feature is some solutes can be regulated independently of others, especially through hormonal control. We will now go go over each of these specifically. To marry. The tubular balance refers to the phenomenon of increased reabsorption rate in response to increased tubular load or inflow. For example, if GFR increases from 125 to 150ml a minute, proximal tubular reabsorption also increases from 81ml a minute to 97.5. Or about 65% of GFR. So. Percentage remains the same. So percentage times of filtrate increases reabsorption. The rate of reabsorption increases as the filtered load increases and the percentage of reabsorption remains is the same constant. Some degree of reabsorption is increased in other tubular segments, but the precise mechanism is not fully understood. This can occur independent of hormones as it can be demonstrated in isolated kidneys. The skull. Mario tubular balance helps prevent overloading of the distal tubular segments when GFR increases. Combined with renal auto regulatory mechanisms, especially the tubular glomerular feedback, these prevent large changes in fluid flow in the distal tubules when arterial pressure or sodium balance changes. Okay. So there's a lot in this slide. So let's go through it slowly. Hydrostatic and colloid osmotic forces govern the rate of reabsorption across the peri tubular capillaries, just as they do with the glomerular capillaries. Uh, more than 99% of the water, and most of the solutes are absorbed from the filtrate as it passes through the renal tubules. The normal rate of tubular capillary reabsorption is 124mm a minute. Of course, this is subject to the hydrostatic and colloid forces that we have discussed before. Net filtration pressure equals capillary pressure minus interstitial hydrostatic pressure minus capillary colloid pressure minus interstitial fluid. Colloid pressure. In this instance we're subtracting the colloid pressure to show the net reabsorption pressure. So let's go through that a little bit more. So reabsorption equals the filtration coefficient times the net forces and the net forces. Equal these. So the capillary pressure, the hydrostatic pressure which is here minus the interstitial hydrostatic pressure. Um. And then the capillary osmotic pressure minus the interstitial osmotic pressure. So, uh, if we said the capillary pressure is 13 minus the interstitial pressure pushing uh into the capillary is six. So those forces are opposing each other. Uh, that leaves us seven. Um. And balance. And that's a seven in the. For filtration, uh, in the direction of excretion. And then we have osmotic pressures. Uh, 32 for the capillary osmotic pressure and a 15 for the interstitial osmotic pressure. So of course you subtract those and you have the balance of 17. And that's 17 in the reabsorption direction. So we have a net reabsorption of ten. Uh, this reabsorption is then multiplied times the, uh, filtration coefficient. And given your overall reabsorption. So the high there is a high filtration coefficient. And that's due to the high hydraulic conductivity and large surface area of the capillaries. And once again hydrostatic pressure, colloid osmotic forces and the large filtration coefficient. The two determinants of period tubular capillary reabsorption, directly influenced by renal hemodynamic changes of the hydrostatic and colloid osmotic pressures. Within the capillaries, arterial pressure raises peri tubular capillary hydrostatic pressure and decreases reabsorption. Increase pressure, uh increases the pressure and the uh outward pressure decreases reabsorption. So smaller reabsorption, uh, an increase in resistance in the afferent or efficient arterials reduces. So if you had increased resistance and after and after and arterials reduced pressure, uh, period to the capillary hydrostatic pressure and increased reabsorption. Um, raising the colloid osmotic pressure increases peri tubular capillary absorption. So if you increase this, you increase this, um, the systemic plasma colloid osmotic pressure raises the tubular capillary colloid osmotic pressure, thereby increasing reabsorption. Of course this is done by raising the plasma protein concentration of the systemic blood. Uh, next, the higher the filtration fraction, which is the amount of, uh, plasma that is filtered into the tubules at the glomerulus. The greater the fraction of plasma filtered through the glomerulus, and the more concentrated the protein becomes in the plasma that remains behind. So the higher the CF, the higher the amount of fluid that is filtered at the glomerulus, and the greater the concentration of the fluid left behind. Um. This increases the filtration fraction and the period tubular capillary reabsorption. Changes in the CF or the filtration coefficient can also change the Re absorption rate. The CF is a measure of the permeability and surface area of the capillaries, so increasing it raises reabsorption and decreasing lowers reabsorption. Uh working through this table, uh, which is table 28 two on page 357 and Guyton um can be a useful memory device. In general forces to increase paired tubular capillary reabsorption also increase reabsorption from the renal tubules. Conversely, hemodynamic changes that inhibit peri tubular capillary reabsorption also inhibit tubular reabsorption of water and solids. A decrease in the reabsorption in the tubular capillaries caused by either an increased in period tubular capillary hydrostatic pressure or a decrease in period tubular capillary colloid osmotic pressure reduces the uptake. Of fluid and solutes from the interstitium. This in turn decreases the uptake from the lumen. So this interstitial space here, if you increase these pressures or increase the hydrostatic pressure or decrease the colloid osmotic pressure, there's a normal uptake and you're pulling from the interstitium. Change these pressures. You can decrease this reabsorption from the interstitium. The decrease in reabsorption from this interstitium of course, uh, increases colloid osmotic pressure here or hydrostatic pressure and decreases the reabsorption from here. Want salutes and we'll further talk about that. Once salutes entered the interstitial channels by either active transport or passive diffusion. So this is here either by active transport or passive diffusion. Water is then drawn into the interstitial by osmosis. So uh water moves from here by osmosis. The solutes can be pulled into the period tubule capillaries here, as this arrow shows, or diffuse back in the air to the tubular lumen so they can go one of two ways. They either go this way or this way, obviously. Um. The size of this arrow does not have any relation to the strength of the attraction. Um. When paired to build capillary reabsorption is reduced. There is increased hydrostatic pressure and a tendency for solids to leak back into the tubular lumen. So if you decrease pair tubular reabsorption, increased pressure in the interstitium would happen. Increasing pressure in the interstitium obviously forces these solutes to head back out. When paired tube capillary absorption is increased the interstitial fluid. So if you did the opposite if you increase reabsorption this. Decreases. Decreases interstitial, uh, hydrostatic pressure, which increases reabsorption from here. Um. Changes in the pressure of the interstitium greatly affect the reabsorption from the tubular lumen. In pressure, natural eases and decreases. Small increases in arterial pressure can cause marked increases in urinary excretion of sodium and water. Between 75 and 160 arterial pressure. There is a small effect on renal blood flow and GFR. When the capillary hydrostatic pressure is increased, there is a decreased amount of reabsorption from the renal tubules. Third, due to increased are two a pressure angiotensin two formation is decreased, leading to decreased sodium reabsorption and decreased aldosterone secretion. And lastly, sodium transporter proteins are internalized. From the apical membranes of the renal tubule, so this was internalized by vessel and removed from the apical membrane. Uh uh, it's obviously not bringing in sodium from the tubule lumen. Uh, this is an example of a sodium chloride transporter in the, uh, distal tubules. Precise regulation of body fluid volumes and salute. Concentration requires the kidneys to excrete different solutes and water at variable rates. At times it must be able to do this while not affecting the retention or secretion of other solids. These are the most important hormones to provide that control. At the same time, stimulation of the sympathetic nervous system can also decrease sodium and water excretion. Now the Austrian is secreted by the zona glomerulus, the cells of the adrenal cortex, and increases the retention of sodium and water while secreting potassium and hydrogen ions. Now the Austrian stimulates a sodium potassium ATP pump on the basal lateral side of the cortical collecting tubule, while increasing sodium permeability on the apical side through the insertion of sodium channels. The most important stimuli for aldosterone release is increased extracellular potassium concentration and increased angiotensin two levels. Angiotensin two release is stimulated by low sodium or low blood volume or blood pressure. Angiotensin two and the resulting aldosterone causes renal sodium and water retention, restoring fluid and blood pressure. When there is an aldosterone deficiency, such as with Addison's disease, there's marked sodium loss and the accumulation of potassium, as opposed to Khan syndrome, which causes excessive aldosterone secretion and sodium retention and excessive secretion of potassium. Angiotensin two release is caused by low blood pressure or low extracellular fluid volume. It causes the retention of fluid and sodium mainly through these three effects. The constriction of arterials increases sodium and water reabsorption by reducing pair tubular capillary hydrostatic pressure, as well as decreasing renal blood flow, which raises the filtration fraction in the glomerulus. This increases the concentration of proteins and colloid osmotic pressure in the paired tubular capillaries. Angiotensin two stimulates sodium reabsorption in the proximal tubules, the loops of hennelly, the distal tubules, and collecting tubules by stimulating the sodium potassium pump on the basal lateral side of the tubular cells, just like our Doster shown on the previous slide. It also stimulates a sodium hydrogen exchanger on the luminal side in the proximal tubule. Angiotensin two stimulates sodium transport across both the apical and basal lateral surfaces of the epithelial cell membrane in most renal tubular segments. The most important renal action of ADH is to increase the water permeability of the distal tubule, collecting tubule and collecting duct epithelium. In the absence of 80 h, the permeability of the collecting ducts is low, causing the kidneys to excrete large amounts of diluted urine, otherwise known as diabetes insipidus. 80 h binds to specific V2 receptors that are G-protein coupled receptors that cause an increase cyclic adenosine monophosphate, or cyclic AMP and consequently activate protein kinase. These protein kinase is stimulate the placement of aquaporins two proteins on the luminal side of the cellular membrane by exocytosis. These water channels permit rapid diffusion of water through the cells. When the added concentration decreases, the aquaporins two molecules are removed and placed back in the cell cytoplasm. Eight year old natural peptide is released by cardiac atrial cells that are stretched by increased plasma volume and increased atrial blood pressure. ANP directly inhibit inhibits reabsorption of sodium and water by the renal tubules, especially in the collecting ducts, as well as the renal secretion and the subsequent formation of angiotensin two. By decreasing sodium and water absorption, urinary excretion is increased, which helps return the blood volume back to normal atrial naturally. Peptide levels are greatly increased in congestive heart failure when the atria are stretched due to decreased ventricular contraction. Parathyroid hormones. Principal action is to increase tubular reabsorption of calcium, especially in the distal and collecting tubules. It also inhibits phosphate reabsorption in the proximal tubule and increases magnesium absorption in the loop of. Sympathetic stimulation causes activation of alpha adrenergic receptors on the renal tubular epithelial cells. Stimulation also increases renin release and angiotensin two formation, which of course decreases the excretion of sodium. The renal clearance of a substance. Is the volume of plasma that is completely cleared of that substance by the kidneys per amount of time. It provides a useful way of quantifying excretory function of the kidneys. If there's one milligram of substance in each milliliter of plasma and one milligram is excreted into the urine each minute, then one milliliter a minute of the plasma is cleared. The formula is shown here and is calculated from the urine excretion rate, which is the urine concentration of that substance times the urinary flow rate divided by the plasma concentration. If a substance is freely filtered and is not reabsorbed or secreted. Then the rate at which it is excreted in the urine is equal to the filtration rate of the substance by the kidneys. GFR can be calculated using inulin since it is not reabsorbed or secreted. Therefore, all the inulin that is in the urine has been filtered. Ethical. Memoryless. In this illustration, the plasma concentration is 1mg/ml. The urine concentration is 125mg/ml, and the urine flow rate is one milliliter a minute. This means that 125mg a minute of insulin passes into the urine. Thus, 125ml of plasma flowing through the kidneys must be filtered to deliver that amount. Other substances have been used clinically to estimate GFR. And we will focus on the most common, which is creatinine. Creatinine is a byproduct of muscle metabolism and is cleared from the body almost entirely by glomerular filtration. Uh, since it is cleared almost entirely by glomerular filtration, it can be used to assess GFR. It's also not required to be given to the patient like insulin is um, it is secreted, uh, in small amounts by the tubules. So the amount excreted slightly exceeds the amount filtered. Uh, instead of measuring the creatine in urine, you can also measure the plasma concentration and estimate changes in GFR. Since a change in GFR will increase the plasma concentration under normal steady state conditions. Creatinine excretion, uh, equals the rate of creatinine production. Uh, the formula says that GFR is approximately equal to creating clearance, which is equal to the urine concentration of creatinine times urine flow rate, um, over the plasma concentration of creatinine. If a substance is completely cleared from the plasma, that clearance rate is equal to the total renal plasma flow. But since GFR is only about 20% of total plasma flow, if a substance is completely cleared from the plasma, it must be excreted by tubular secretion. The filtration fraction can also be calculated by dividing the GFR by the renal plasma flow. And lastly, the amount of tubular reabsorption or secretion can be calculated if the rates of Gomery filtration and renal excretion are known. For example, if the rates of excretion are less than what was filtered, then there must be some reabsorption. Conversely, if the rate of excretion exceeds the rate of filtration, then there must be some secretion in the tubes.