Chapter 3 Toward Defining Nature of Engineering in the Next Generation Science Standards Era PDF
Document Details
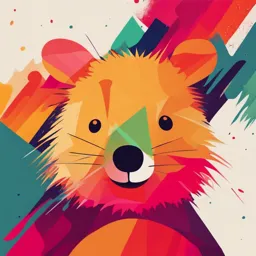
Uploaded by CuteBromeliad
null
Hasan Deniz, Ezgi Yesilyurt, Steven J. Newman, and Erdogan Kaya
Tags
Related
- Science and Engineering for Grades 6-12: Investigation and Design at the Center (2019) PDF
- Science and Engineering for Grades 6-12 Investigation and Design at the Center (2019) PDF
- Complete Chemistry Textbook Grade 9 - Pakistan 2022-2023 PDF
- Environmental Studies Comprehensive Notes PDF
- Children's Rural Science Knowledge (2011) PDF
- Advanced Research Methods in Materials Engineering (PDF)
Summary
This chapter introduces the concept of the nature of engineering (NOE) and discusses its importance in K-12 engineering education. It examines the similarities between NOE and the nature of science (NOS), highlighting their role in scientific literacy and STEM education. The chapter also explores various aspects of NOE and suggests strategies for incorporating them into the engineering design process.
Full Transcript
Chapter 3 Toward Defining Nature of Engineering in the Next Generation Science Standards Era Hasan Deniz, Ezgi Yesilyurt, Steven J. Newman, and Erdogan Kaya 3.1 Introduction The Next Generation Science Standards (NGSS Lead States, 2013) placed a special emphasis on engineering and identified eng...
Chapter 3 Toward Defining Nature of Engineering in the Next Generation Science Standards Era Hasan Deniz, Ezgi Yesilyurt, Steven J. Newman, and Erdogan Kaya 3.1 Introduction The Next Generation Science Standards (NGSS Lead States, 2013) placed a special emphasis on engineering and identified engineering as a discipline to be included in science classrooms in the United States. Engineering secured a higher status in the NGSS compared to the previous science education policy documents (AAAS 1993; NRC 1996). Engineering like science can be conceived as including three domains: (a) engineering as a body of knowledge, (b) engineering as a set of practices, and (c) engineering as a way of knowing (nature of engineering) (Hartman, 2016). It is reasonable to think that there is a parallel between NOE and nature of science (NOS) aspects when we read NOE conceptualizations (Hartman, 2016; Karataş, 2009; NRC, 2012) in light of NOS conceptualizations (Lederman, 2007; Osborne, Collins, Ratcliffe, Millar, & Duschl, 2003). NOS is a well-established research area in science education. Therefore, the developing NOE research agenda and the attempts to conceptualize NOE ideas can be informed by the historical trajectory of NOS scholarship in science education. One can realize that NOE conceptions included in the NGSS are similar to NOS conceptions included in Appendix H of the NGSS and the agreed upon NOS conceptions (Lederman, 2007; Osborne et al., 2003). Understanding NOS is considered as an essential component of scientific liter- acy (AAAS 1993; Abd-El-Khalick & Lederman, 2000; NRC, 2000) since it pro- vides an insight into how scientific knowledge develops in real world. Similarly, NOE can be considered as an important component of larger STEM literacy because H. Deniz (*) · E. Yesilyurt · E. Kaya University of Nevada Las Vegas, Las Vegas, NV, USA e-mail: [email protected] S. J. Newman Indiana University, Bloomington, IN, USA © Springer Nature Switzerland AG 2020 33 V. L. Akerson, G. A. Buck (eds.), Critical Questions in STEM Education, Contemporary Trends and Issues in Science Education 51, https://doi.org/10.1007/978-3-030-57646-2_3 34 H. Deniz et al. it helps people to appreciate creative, subjective, tentative, social, and socio-cultural aspects of engineering design process while engineers develop solutions to human problems. In line with other chapter authors in this book, we view STEM as mean- ingful interdependence among all disciplines of STEM. In other words, includes all individual disciplines of STEM (science, technology, engineering, and mathemat- ics) in a way that is meaningful and showcases the interdependence of the fields. Epistemic beliefs about engineering (NOE views) can influence the knowledge acquisition, interpretation of problems, and the selection of problem-solving strate- gies during the engineering problem solving (McNeill, Douglass, Koro-Ljungberg, Therriault, & Krause, 2016). From this perspective, NOE should be an integral part of an effective engineering education (Karatas, Goktas, & Bodner, 2010). In this chapter, we aimed to discern NOE aspects relevant to K-12 engineering education from the literature, the Framework (NRC, 2012), and to suggest ways to teach NOE integrated with the engineering design process. 3.2 Nature of Engineering Unlike NOS, there is no consensus about NOE conceptions relevant to K-12 educa- tion (Karataş, Bodner, & Unal, 2016). However, there is a need to establish an agreed-upon list of nature of engineering (NOE) aspects to teach NOE as an integral part of the engineering design process described in the NGSS. One can attempt to create a list of NOE aspects by tapping into NOS literature (Karataş, 2009), con- ducting a Delphi study (Hartman, 2016) and by surveying state standards (Carr, Bennett IV, & Strobel, 2012). Karataş (2009) developed a list of NOE aspects based on literature. The list included the notions that (1) engineering solutions are tentative, (2) they require creativity, imagination, and the ability to integrate knowledge from other disci- plines, (3) they utilize current scientific and mathematical theories, and learn from previous successes and failures, (4) they require decision making based on certain criteria and constraints as well ethical considerations, (5) they are socially and cul- turally embedded, (6) they are goal-oriented addressing a specific human need or desire, (7) they require holistic thinking, and (8) they may vary because there might be more than one solution to the same problem. Hartman (2016) conducted a 3-phase Delphi study to identify NOE aspects rel- evant to K-12 engineering education. At least 61 subjects participated in first, sec- ond, and third round of the study. Participants included 15 K-12 science teachers, 18 K-12 engineering teachers, 17 science education faculty, and 15 engineering education faculty. Hartman (2016) identified eight NOE aspects. These aspects include the notions that (1) there are multiple solutions to the same problem, (2) engineering solutions require creativity, (3) engineering solutions are reached through an iterative engineering design process involving learning from failure, (4) engineering solutions utilize mathematical, visual, and physical modeling, (5) engi- neering solutions require communication, (6) engineering solutions are evaluated 3 Toward Defining Nature of Engineering in the Next Generation Science Standards Era 35 based on certain criteria and constraints, (7) engineering solutions require collabo- ration and teamwork, and (8) engineering is a unique way of knowing which has similarities and differences with science and other disciplines. These eight NOE aspects were selected based on consensus and stability criteria. Consensus criterion indicates that at least 75% of participants rated each NOE aspect at four or greater on a 5-point scale (4-important, 5-very important) and stability criterion means that for each NOE aspect a similar level of consensus was achieved in both round two and round three. After achieving a consensual list of NOE aspects as a result of a Delphi study, Hartman (2016) suggested major revisions on this list. He recom- mended to include the design process to the list because the design process was the second most highly rated NOE aspect in the second round but this particular aspect was not included in the third round due to stability criterion. He also noted that the Delphi study participants warned against teaching the engineering design process as a list of steps similar to the steps of the so-called scientific method. Hartman also suggested to treat creative, communicative, and collaborative NOE aspects as prac- tices of engineering rather than NOE aspects. Hartman differentiated unique way of knowing NOE aspect from other NOE aspects by stating that “this is an overarching concept in engineering and not an aspect of the nature of engineering” p.124). After these major revisions, Hartman’s NOE list included five NOE aspects: design driven, divergent, iterative model-driven, and constrained by criteria. Carr et al. (2012) surveyed all 50 states academic standards to identify instances of engineering content in existing standards to attempt to determine if a consensus on the big ideas of engineering may already exist. Engineering skills and knowledge were found in 41 states’ standards. Word analysis was performed on both state engi- neering and design standards to visually portray the “big ideas” of engineering. The top five “big idea” words identified in engineering standards were: design, technol- ogy, use, process, and problem (Carr et al., 2012). The top five verbs prevalent in the design standards were: need, criteria, constraints, model, and data (Carr et al., 2012). When the standards were compiled, an inconclusive consensus of 19 “big ideas” of what consisted of engineering was found. Hence, this further demonstrates that potential NOE aspects have been present in existing state standards, yet never explicitly termed and defined to be included in national standards. Even though the term nature of engineering (NOE) was not used in the Framework (NRC, 2012) and the NGSS, these documents include NOE ideas throughout if one looks specifically for them. Table 3.1 illustrates the NOE aspects that we discerned from the Framework (NRC, 2012) and the NGSS. Excerpts that are provided in Table 3.1 are by no means exhaustive. We chose to include these particular excerpts because they clearly reflect their corresponding NOE aspect. The italic text in Table 3.1 represents our own definition of NOE aspects. 36 H. Deniz et al. Table 3.1 Descriptions of Nature of Engineering (NOE) Aspects NOE Aspect Description Demarcation (What is Engineering is systematically engaging in the practice of design to engineering? What achieve solutions for specific problems. Engineers apply their makes engineering understanding of the natural world (scientific knowledge) to design different from other solutions for real world problems. disciplines?) In the K-12 context, “science” is generally taken to mean the traditional natural sciences: physics, chemistry, biology, and (more recently) earth, space, and environmental sciences…We use the term “engineering” in a very broad sense to mean any engagement in a systematic practice of design to achieve solutions to particular human problems. Likewise, we broadly use the term technology to include all types of human-made systems and processes-not in the limited sense often in schools that equates technology with modern computational and communications devices. Technologies result when engineers apply their understanding of natural world and of human behavior to design ways to satisfy human needs and wants. (NRC, 2012, p. 11–12) Engineering design Engineering design process is viewed as both domain knowledge and process (EDP) the central practice of engineering in the Framework (NRC, 2012). There is an overall agreement on the components of the engineering design process described below. The core idea of engineering design includes three component ideas: Define, design, and optimize A. Define: Defining and delimiting engineering problems involves stating the problem to be solved as clearly as possible in terms of criteria for success and constraints or limits. B. Design: Designing solutions to engineering problems begin with generating a number of possible solutions. These potential solutions are then evaluated to assess which ones best meet the criteria and constraints of the problem. C. Optimize: Optimizing the design solution involves a process in which solutions are systematically tested and refined and the final design is improved by trading off less important features for those that are more important (NGSS Lead States, 2013, Appendix I, p. 104) Empirical basis Engineers optimize their design solutions and compare alternative solutions based on evidence obtained from test data. … engineers engage in testing that will contribute data for informing proposed designs. A civil engineer, for example, cannot design a new highway without measuring the terrain and collecting data about the nature of the soil and water flows (NRC, 2012, p. 45) Engineers use investigation both to gain data essential for specifying design criteria or parameters and to test their designs. Like scientists, engineers must identify relevant variables, decide how they will be measured, and collect data for analysis. Their investigations help them to identify how effective, efficient, and durable their designs may be under a range of conditions (NRC, 2012, p. 50) (continued) 3 Toward Defining Nature of Engineering in the Next Generation Science Standards Era 37 Table 3.1 (continued) NOE Aspect Description Tentativeness Engineering design solutions are subject to change. Engineering design solutions can be revised to better achieve the desired goal or they can be revised to satisfy different criteria. Phases of engineering design process do not always follow in order, any more than do the “steps” of scientific inquiry. At any phase, a problem solver can redefine the problem or generate new solutions to replace an idea that is just not working out (NGSS Lead States, 2013, Appendix I, page 104). Creativity Creativity and imagination of engineers play a major role during the engineering design process. The role of creativity and imagination is not limited to any specific component of the engineering design process. Engineering and science are similar in that both involve creative processes, and neither uses just one method (NRC, 2012, p. 46). Subjectivity There is no unique solution to an engineering design problem. While there can be many solutions to the same problem, some of these solutions may be more suited to meet the criteria and constraints of the problem. There is usually no single best solution but rather a range of solutions. Which one is the optimal choice depends on the criteria used for making evaluations (NRC, 2012, p. 52). Social aspects of Engineering is not a solitary pursuit. Engineering design solutions engineering are constructed through social negotiation. Despite their individual differences, members of an engineering community share common understandings, traditions, and values. This social dimension enhances the quality of engineering design solutions. The work of engineers, like the work of scientists, involves both individual and cooperative effort; and it requires specialized knowledge (NRC, 2012, p. 28). Engineers cannot produce new or improved technologies if the advantages of their designs are not communicated clearly and persuasively. Engineers need to be able to express their ideas, orally and in writing, with the use of tables, graphs, drawings, or models and by engaging in extended discussions with peers. Moreover, as with scientists, they need to be able to derive meaning from colleagues’ texts, evaluate the information, and apply it usefully. In engineering and science alike, new technologies are now routinely available that extend the possibilities for collaboration and communication (NRC, 2012, p. 53). (continued) 38 H. Deniz et al. Table 3.1 (continued) NOE Aspect Description Social and cultural Engineering is a human activity. There is a continued interaction embeddedness between engineering and society. Sociocultural factors influence the engineering design process, and in turn, engineering influences the society. These social and cultural factors include social composition, religion, worldview, political, and economic factors. Not only do science and engineering affect society, but society’s decisions (whether made through market forces or political processes) influence the work of scientists and engineers. These decisions sometimes establish goals and priorities for improving or replacing technologies; at other times they set limits, such as in regulating the extraction of raw materials or in setting allowable levels of pollution from mining, farming, and industry (NRC, 2012, p. 213) Criteria or constraints Engineers make decisions based on predetermined criteria and constraints during the engineering design process. Making gains on one criterion often involves losing on another criterion or other criteria. Engineers must contend with a variety of limitations, or constraints, when they engage in design. Constraints, which frame the salient conditions under which the problem must be solved, may be physical, economic, legal, political, social, ethical, aesthetic, or related to time and place. In terms of quantitative measurements, constraints may include limits on cost, size, weight, or performance, for example. And although constraints place restrictions on a design not all of them are permanent or absolute (NRC, 2012, p. 205). Failure-laden Failure in engineering design is inevitable and provides opportunities for improving design solutions. Engineers engage in multiple iterations to enhance the quality of their products and systems. Tests are often designed to identify failure points or difficulties, which suggest the elements of the design that need to be improved (NRC, 2012, p. 207). Engineers often look for and analyze patterns, too. For example, they may diagnose patterns of failure of a designed system under test in order to improve the design (NRC, 2012, p. 86). 3.3 Research on NOE The number of studies examining students’ NOE views is limited (e.g., Capobianco, Diefes-Dux, Mena, & Weller, 2011; Fralick et al., 2009; Karataş et al., 2016). The research on NOE views focused mostly on examining students’ knowledge about engineering and engineers’ work. The Draw an Engineer Test (DAET), which is a modified version of the Draw a Scientist Test (DAST), has been commonly used by the researchers with regard to students’ views of engineering (Knight & Cunningham, 2004). This instrument asks learners to draw an engineer at work and provide expla- nations for their drawings. Studies using DAET illustrated that students had limited knowledge about engineering. They usually associated engineering with building and fixing, and engineers with workers and laborers. Knight and Cunningham 3 Toward Defining Nature of Engineering in the Next Generation Science Standards Era 39 (2004), for instance, studying with 384 K-12 students found that most of the stu- dents drew artifacts of the building and fixing such as heavy machinery and hard hat, and products of engineering such as cars, machines and engines. The students’ drawings illustrated that students perceived engineers as construction workers or mechanics. Only a few students portrayed engineers thinking and sketching their designs on a paper. Likewise, Fralick, Kearn, Thompson, and Lyons (2009) attempted to compare and contrast the 1600 middle school students’ conceptions of engineers and scien- tists through DAST and DAET instruments. Students’ drawings showed that stu- dents perceived engineering as a profession mainly involving physical labor. Specifically, they drew engineers as a doer or a worker bee. They portrayed engi- neers wearing laborers’ clothing in outdoor and operating vehicles, or building structures. Compared to their drawings of scientists, most students depicted engi- neers with no inferred action which illustrated that students lack knowledge about the engineering design process. Further, students portrayed engineers less scholarly than scientists. While they drew scientists thinking and with books, and mathemati- cal symbols, they portrayed engineers mostly with mechanics tools. All in all, the students had more informed views with respect to the field of science than they do about engineering. They had insufficient knowledge about the cognitive and cre- ative aspects of the engineering design process. Similarly, Capobianco et al. (2011) interviewed elementary students from each grade by using the DAET instrument to explore their conceptions of engineers. Students’ drawings and interview analyses revealed four emergent themes with respect to students’ conceptions about engineers. First, students depicted engineers primarily as mechanics who work on vehicles. Several younger students portrayed engineers as an object and an engine. Second, several students perceived engineers as tradesmen who fix and build roads and objects. Third, students depicted techni- cians who work on electronic devices (e.g., computer, television, telephones). These students thought that the engineering profession requires technical skills. Last, few students, only fourth and fifth-grade students, conceived that the engineering pro- fession involves designing buildings, electronic devices, and vehicles. Although few upper elementary students seemed to have relatively more informed views as com- pared to lower elementary students, the majority of the fourth and fifth-grade stu- dents still perceived engineering as involving mainly mechanical labor. In light of their findings, the researchers made a list of key attributes of an engineer for engi- neering education. These attributes included creativity, working in teams, using sci- ence, mathematics and technology, solving human problems, and designing everything around us. Although the DAET instrument allows us to capture students’ general ideas about engineers and engineering, it does not provide detailed information with regard to students’ NOE conceptions across NOE aspects that we described in Table 3.1. For this reason, Karatas, Micklos and Bodner (2011) conducted interview sessions using pictures of engineering artifacts along with sixth grade students’ own drawings to assess their NOE conceptions with regard to the definition of engineer- ing, the engineering design process, the demarcation between science and 40 H. Deniz et al. engineering, and the role of engineering in society. Although students in this study thought that building structures, fixing and assembling vehicles as part of the design process, nearly half of the students perceived engineering as an active and dynamic process including planning, designing, creating and testing phases. However, none of the students addressed all phases of the design process and they focused only on two or three phases of the engineering design process. Therefore, the study indi- cated that students envisioned engineers as skilled craftsmen. As for the demarca- tion between science and engineering, most of the students struggled to differentiate between science and engineering while only a few students stressed that engineers design and build products while scientists work on nature. Later, Karataş et al. (2016) developed a 12-item views of the nature of engineer- ing (VNOE) questionnaire to explore the first-year engineering students’ views engineering and design process. The inductive data analyses indicated that majority of students perceived engineering profession involving the mental tasks including sketching, conceptual representations of the design, and overseeing the construc- tion. Most of the students thought that engineering involves problem-solving pro- cess which aims at improving engineering designs and solving human and environmental problems. On the other hand, students failed to explain the differ- ences between science and engineering and to mention the role of science and math knowledge, and teamwork in engineering design. This study showed the importance of using questions specifically addressing NOE aspects to provide a comprehensive analysis of students’ views of NOE. However, the authors did not address the valid- ity and reliability issues regarding the instrument. Overall, studies indicated that K-12 students held uninformed views of NOE aspects. On the other hand, no valid and reliable instrument is available in the related literature to fully capture K-12 students’ NOE conceptions. It is, therefore, necessary to develop a consensus list of NOE aspects relevant to K-12 education, which can pave the way for the development of valid and reliable instruments to assess learners’ NOE views. 3.4 Teaching NOE After the release of the NGSS, mounting pressure has been applied to in-service and pre-service teachers to be able to incorporate both science and engineering into les- son plans via cross-cutting concepts. As highlighted through a recent editorial piece (Akerson et al., 2018), concerns over how to get current in-service and pre-service science teachers to become proficient, not only in teaching NOS, but furthermore NOE, when science educators have not been able to successfully help (or at least most) K-12 science teachers conceptualize NOS, their home discipline (Akerson et al., 2018). This presents a compounding problem in the teacher preparation pipe- line—the science teacher educators with no engineering backgrounds are preparing the science teachers, who also have no engineering backgrounds (Akerson et al., 2018). Despite this dire situation, science educators can model teaching NOE in professional development programs and science teaching methods courses by con- sidering effective features of explicit-reflective NOS teaching (Adibelli-Sahin & Deniz, 2017). 3 Toward Defining Nature of Engineering in the Next Generation Science Standards Era 41 Rich NOS literature can offer insights about how to teach NOE aspects as well as NOS aspects. Students should experience the engineering design process in their science classes but the activity itself should not be enough. Students should reflect on the epistemological aspects of the engineering design process while they are actively engaged in the engineering design activity. To this end, the science and engineering education community should achieve a consensus list of NOE aspects relevant to K-12 education and decide which NOE aspects will be taught in each grade level at what level of sophistication similar to the NOS aspects presented in Appendix H of the Next Generation Science Standards. The research with regard to what kinds of strategies are effective in improving students’ NOE views is limited. However, NOS literature is rich with studies sug- gesting that engaging students in inquiry activities does not necessarily improve their NOS views if the students are not intentionally introduced to NOS ideas through explicit-reflective NOS instruction (Lederman, 2007). Explicit-reflective NOE instruction should intentionally draw students’ attention to relevant NOE aspects during the engineering design activity and encourage students to revise their NOE ideas in light of their experience during the engineering design activity. While explicit part of this instruction refers to making NOE aspects visible to the students, the reflective part refers to encouraging learners to revise their NOE ideas in light of their engineering design experiences. For example, Deniz, Yesilyurt, and Kaya (in press) provided a practical example of how explicit-reflective instruction can be adapted to teach NOE aspects. Authors described in detail how they used picture books to teach selected NOE aspects to grades 3–5 elementary students using an explicit-reflective instruction embedded within an engineering design activity. The authors aligned each phase of the engineering design process with a particu- lar picture book. The authors described how they used picture books to reflect on students’ experience in a particular engineering design process phase from the per- spective of targeted NOE ideas by the book reading. Each book targeted minimum one and maximum three NOE ideas. For example, during create phase of the engi- neering design process, the authors read the picture book The Most Magnificent Thing (Spires, 2014). This book tells the story of a girl building a special scooter. The picture book conveys the idea that engineers use their creativity and imagination, and should be persistent in the face of failure to successfully complete their projects. By reading this picture book the authors explicitly stated that engineers could revise their design ideas (tentative NOE) by using their creativity and imagination (creative NOE) while persevering in the face of failure (failure-laden NOE). The authors also supported their explicit NOE instruction by referring to a NOE poster strategically placed in the classroom. The authors reminded students to refer to the NOE poster during the engineering design process and they used the poster to explicitly identify NOE aspects during the reflective debriefing activity at the end of the engineering design challenge. The debriefing activity involved specifically prepared questions addressing each targeted NOE aspect. For example, authors asked questions such as “In what ways did you use your creativity and imagination during the engineering design process?” and “Did each group develop the same design?” to start the reflec- tion around creative NOE aspect and subjective NOE aspect, respectively. 42 H. Deniz et al. 3.5 Conclusion Nature of engineering research is at its infancy. In order to advance NOE as a new research agenda, we need to make progress on three fronts: (a) achieving consensus around NOE aspects relevant to K-12 education, (b) developing valid and reliable instruments to assess students’ and teachers’ NOE views, and (c) developing effective methods to teach NOE. In order to achieve the same level of rigor in NOS research, achieving consensus around NOE aspects, and then developing valid and reliable instruments to assess these agreed-upon NOE aspects are of primary importance. There were some initial attempts to achieve consensus on NOE engineering ideas relevant to K-12 education (Hartman, 2016; Karataş, 2009), but these attempts still did not establish a secure base for NOE aspects similar to agreed-upon NOS aspects (Lederman, 2007). Recently, the Framework (NRC, 2012) and the Next Generation Science Standards (NGSS Lead States, 2013) portrayed certain NOE ideas without defining NOE as a construct similar to NOS portrayed in Appendix H of the NGSS. Having these valid and reliable instruments can help us to develop more effec- tive methods to teach NOE. The current NOE research primarily uses interviews and Draw an Engineer Test (DAET) to assess learners’ NOE views. Nature of engineering needs valid and reliable open-ended instruments similar to the Views of Nature of Science (VNOS) instruments (Lederman, Abd-El-Khalick, Bell, & Schwartz, 2002). Thanks to the rich NOS literature, we have a significant number of research articles reporting about which instructional approaches are more effective in improving learners’ NOS views (Lederman, 2007). We also have a significant num- ber of practitioner articles (Lederman, Bartels, Lederman, & Gnanakkan, 2014; McComas, 2004) and book chapters (McComas, 1998; McComas, 2020) describing effective methods to teach NOS in K-12 education. Fledgling NOE scholarship can easily borrow from this rich NOS literature to design effective teaching strategies in improving learners’ NOE views. For instance, we should not expect learners to improve their NOE views simply because they participated in an engineering design activity. The NOS literature is filled with studies reporting that learners may not improve their NOS views even if they participate in a scientific inquiry (Abd-El- Khalick & Lederman, 2000; Bell, Blair, Crawford, & Lederman, 2003). Engineering literacy has been defined as (a) discuss, critique, and make decisions about national, local, and personal issues that involve engineering solutions, (b) understand and explain how basic societal needs (e.g., water, food, and energy) are processed, produced, and transported, (c) solve basic problems faced in everyday life by employing concepts and models of science, technology, and mathematics (Chae, 2010). We believe that engineering design process along with its epistemological aspects (NOE) should be integrated to science curriculum at each grade level as it was recommended by the NGSS. This integration can be successfully achieved in both elementary (Deniz, Kaya, & Yesilyurt, 2018) and secondary grades (Roehrig, Moore, Wang, & Park, 2012). Through a more integrated approach, students can be exposed to engineering earlier and more, thereby increasing their own engineering literacy. 3 Toward Defining Nature of Engineering in the Next Generation Science Standards Era 43 References Abd-El-Khalick, F., & Lederman, N. G. (2000). Improving science teachers’ conceptions of the nature of science: A critical review of the literature. International Journal of Science Education, 22(7), 665–701. Adibelli-Sahin, E., & Deniz, H. (2017). Elementary teachers’ perceptions about the effective features of explicit reflective nature of science instruction. International Journal of Science Education, 39(6), 761–790. Akerson, V. L., Burgess, A., Gerber, A., Guo, M., Khan, T. A., & Newman, S. (2018). Disentangling the meaning of STEM: Implications for science education and science teacher education. Journal of Science Teacher Education, 29(1), 1–8. American Association for the Advancement of Science. (1993). Benchmarks for science literacy: Project 2061. New York: Oxford University Press. Bell, R. L., Blair, L. M., Crawford, B. A., & Lederman, N. G. (2003). Just do it? Impact of a sci- ence apprenticeship program on high school students’ understanding of the nature of science and scientific inquiry. Journal of Research in Science Teaching, 40(5), 487–509. Capobianco, B. M., Diefes-Dux, H. A., Mena, I., & Weller, J. (2011). What is an engineer? Implications of elementary school student conceptions for engineering education. Journal of Engineering Education, 100(2), 304–328. Carr, R. L., Bennett IV, L. D., & Strobel, J. (2012). Engineering in the K-12 STEM standards of the 50 US states: An analysis of presence and extent. Journal of Engineering Education, 101(3), 539–564. Chae, Y. (2010). AC 2010-1287: Core concepts for engineering literacy: The interrelationship among STEM disciplines. Age, 15(1). Deniz, H., Kaya, E., & Yesilyurt, E. (2018). The soda can crusher challenge: Exposing elementary students to the engineering design process. Science & Children, 56(2), 74–78. Deniz, H., Yesilyurt, E., & Kaya, E. (in press). Teaching nature of engineering with picture books. Science & Children. Fralick, B., Kearn, J., Thompson, S., & Lyons, J. (2009). How middle schoolers draw engineers and scientists. Journal of Science Education and Technology, 18(1), 60–73. Hartman, B. D. (2016). Aspects of the nature of engineering for K-12 science education: A Delphi study [Doctoral Dissertation, Oregon State University]. https://ir.library.oregonstate.edu/ concern/graduate_thesis_or_dissertations/t148fk693. Karataş, F. Ö. (2009). First-year engineering students’ views of engineering (Doctoral disserta- tion). Retrieved from Proquest. Karataş, F. Ö., Bodner, G. M., & Unal, S. (2016). First-year engineering students’ views of the nature of engineering: Implications for engineering programmes. European Journal of Engineering Education, 41(1), 1–22. Karatas, F.O., Goktas, Y., & Bodner, G. M. (2010). An argument about nature of engineer- ing (NOE) and placing the NOE into engineering education curriculum. In Proceedings of Turkey’s vision 2023 conference series: International engineering education conference, 4–6 November, Antalya, Turkey. Karatas, F. O., Micklos, A., & Bodner, G. M. (2011). Sixth-grade Students’ views of the nature of engineering and images of engineers. Journal of Science Education and Technology, 20(2), 123–135. Knight, M., & Cunningham, C. (2004, June). Draw an engineer test (DAET): Development of a tool to investigate students’ ideas about engineers and engineering. In ASEE annual conference and exposition (Vol. 2004). Lederman, J., Bartels, S., Lederman, N., & Gnanakkan, D. (2014). Demystifying nature of science. Science and Children, 52(1), 40–45. 44 H. Deniz et al. Lederman, N. G. (2007). Nature of science: Past, present, and future. In S. K. Abell & N. G. Lederman (Eds.), Handbook of research on science education (pp. 831–879). Mahwah, NJ: Erlbaum. Lederman, N. G., Abd-El-Khalick, F., Bell, R. L., & Schwartz, R. S. (2002). Views of nature of sci- ence questionnaire: Toward valid and meaningful assessment of learners’ conceptions of nature of science. Journal of Research in Science Teaching, 39(6), 497–521. McComas, W. F. (1998). The nature of science in science education: Rationales and strategies. Dordrecht, The Netherlands: Kluwer Academic. McComas, W. F. (2004). Keys to teaching nature of science. Science Teacher, 71(9), 24–27. McComas, W. F. (2020). Nature of science in science instruction: Rationales and strategies Springer. McNeill, N. J., Douglass, E. P., Koro-Ljunberg, M., Therriault, D., & Krause, I. (2016). Undergraduate students’ beliefs about engineering problem solving. Journal of Engineering Education, 105(4), 560–584. National Research Council. (1996). National science education standards. Washington, DC: National Academy Press. National Research Council. (2000). Inquiry and the national science education standards. Washington, DC: National Academy Press. National Research Council. (2012). A framework for K-12 science education: Practices, crosscut- ting concepts, and Core ideas. Committee on a conceptual framework for new K-12 science education standards. Board on science education, division of behavioral and social sciences and education. Washington, DC: The National Academies. NGSS Lead States. (2013). Next generation science standards: For states, by states. Washington, DC: The National Academies Press. Osborne, J. F., Collins, S., Ratcliffe, M., Millar, R., & Duschl, R. (2003). What ‘ideas about sci- ence’ should be taught in school science? A Delphi study of the ‘expert’ community. Journal of Research in Science Teaching, 40(7), 692–720. Roehrig, G. H., Moore, T. J., Wang, H. H., & Park, M. S. (2012). Is adding the E enough? Investigating the impact of K-12 engineering standards on the implementation of STEM inte- gration. School Science and Mathematics, 112(1), 31–44. Spires, A. (2014). The most magnificent thing. Tonawanda, NY: Kids Can Press. Hasan Deniz is a Professor of Science Education at University of Nevada Las Vegas. His research focuses on preservice and inservice teachers’ ideas about Nature of Science-Nature of Engineering, Engineering Education, and Evolution Education. He is the Director of Center for Math, Science, and Engineering Education at University of Nevada Las Vegas. Ezgi Yesilyurt is an Assistant Professor of Life Science Education at Weber State University. Her research focuses on preservice and inservice teachers’ ideas about Nature of Engineering, Engineering Education, and Evolution Education. Steven J. Newman is a PhD student in Science Education at Indiana University. He transitioned from a background in scientific bench research to further education in teaching science at the col- lege level. His research interests are on examining the interaction of co-teaching on teacher knowl- edge and student-teacher interactions. Erdogan Kaya is an Assistant Professor of Computer Science Education at George Mason University. His research focuses on preservice and inservice teachers’ ideas about Nature of Engineering, Engineering Education, and Computer Science education.