Enzymes PDF
Document Details
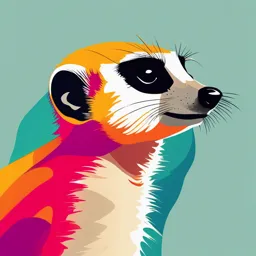
Uploaded by Ryderhunt14
Tags
Summary
This document appears to be about enzymes. Enzymes are biological catalysts that speed up chemical reactions in cells.
Full Transcript
1 2 The law of conservation of energy (which you likely learned about in Science 10 or Chemistry 11) states that energy can not be created or destroyed, but it can be transformed from one form to another. Chemical reactions (including those reactions that occur in the body) involve energy transfer...
1 2 The law of conservation of energy (which you likely learned about in Science 10 or Chemistry 11) states that energy can not be created or destroyed, but it can be transformed from one form to another. Chemical reactions (including those reactions that occur in the body) involve energy transfer. Energy is required to break bonds and energy is released when chemical bonds form. When a chemical reaction occurs, atoms in the reactants rearrange their chemical bonds to make products. The new arrangement of bonds does not have the same chemical energy as the bonds in the reactants. 3 In biology, the most common energy that we refer to is Gibbs Free energy. Gibbs free energy is the energy that is available to do useful work. If you’ve taken chemistry 12, you’ve learned about enthalpy and entropy, and learned to classify reactions as exothermic or endothermic. You’ve also learned about how to determine if a reaction will reach equilibrium, go to completion, or not occur, based on changes in enthalpy and entropy. Gibbs free energy combines enthalpy and entropy into one value to determine if a reaction will be spontaneous or not. 4 Scientist use free energy diagrams to describe how free energy changes during reactions. The difference between the energy of the products and the reactants is called ∆G, or change in free energy Free energy diagrams can be used to predict whether or not reactions will be spontaneous (occur on their own), or if the reaction will require external sources of energy (like ATP). Studying free energy changes is important in biology because is helps us to explain how cells harness energy from chemical reactions to perform jobs like building molecules, transporting substances, and contracting muscles. The vertical axis shows free energy (G) and the horizontal axis shows reaction progress. 5 In an exergonic reaction, free energy decreases as the reaction proceeds (starting with reactants to finishing with the products). Exergonic reactions are spontaneous (occur on their own). Energy is released. ΔG is negative (The symbol Δ is delta – it represents a change). Free energy is converted into energy that can be used in other processes. In an endergonic reaction, free energy increases as the reaction proceeds. Endergonic reactions are non-spontaneous (they do not occur naturally under the given conditions). Energy is absorbed. ΔG is positive. In the body, energy is often provided by ATP, the energy currency of the cell. 6 Exergonic reactions result when larger, more complex molecules are broken down into simpler molecules. These reactions are also referred to as catabolic reactions. (Think: cats break stuff into smaller pieces). Examples that we have seen include cellular respiration, where the glucose molecule is broken down by the mitochondria. This releases energy that is stored by ATP. Another example is during digestion. Large polymers, such as starch are broken down into monomers, such as glucose. This releases energy. Endergonic reactions result when simple molecules come together and form more complex molecules. We’ve seen these types of reactions in the previous unit. During protein synthesis, long chains of amino acids are synthesized. This requires energy from ATP. As well, during mRNA transcription, or during DNA replication, nucleotides are joined together, using ATP as the energy source. Metabolism includes all of the chemical reactions (exergonic and endergonic) that occur in the body. 7 Here is another diagram to show how exergonic and endergonic reactions are related, and the role that ATP plays. 8 So what happens between reactants and products? How do we get there? Label: Energy of reactants (left side top); Energy of products (right side top), Activation energy (middle), ΔG (middle right). This free energy diagram represents a endergonic reaction The energy needed to “activate” or start the chemical reaction is called the activation energy. The state where bonds are being broken and formed at the same time is called the transition state, or activated complex. This state is highly unstable, and cannot be directly observed. Forming this requires an input of energy. The higher the activation energy barrier, the slower the reaction is. 9 Label: Activation energy with an enzyme (left side); Activation energy without an enzyme (right side) and highlight the lines. To speed up reactions that have high activation energy, or high energy needs, chemists use inorganic catalysts. In biological systems, the catalyst is an enzyme. If you remember from the previous units, enzymes are generally proteins. They have specific shapes (caused by their tertiary and quaternary structures) that give them their function. Catalysts (including enzymes) lower the activation energy of reactions. This speeds up the reaction. It does not change the energy of the overall reaction (ΔG). 10 Enzymes are like little molecular machines or robots. Most enzymes are one-task machines made of proteins. They can only complete one action, like a worker at one station of an assembly line. Each enzyme has a unique job, a unique substrate, and a unique active site. Enzymes speed up or catalyze biochemical reactions. In this diagram, the substrate is the small glucose molecule that fits into the enzyme (in purple/black). You can see the 3D structure of the protein that makes up the enzyme, and that it is made of more than one polypeptide (quaternary structure). Interactions between the R-groups of the amino acids make the protein fold this way. 11 In a solution containing the substrate and the enzyme, the two molecules bounce around in the solution until the substrate bumps into the enzyme in just the right orientation so that it binds with the active site. Once this occurs, the enzyme and substrate are stuck together as the enzyme-substrate complex. The active site breaks the bonds in the substrate (here, the pentagon is broken off the rest of the substrate. At this point, the products are released from the enzyme, and the enzyme is free to catalyze more reactions. 12 This diagram shows a more realistic depiction of the actual molecules and their shapes. The enzyme is a protein, which has been folded. The specific order of the amino acids, and their properties determines the tertiary/quaternary structure of the protein. This creates a particular shape, the active site, which will bind to the substrate and catalyze a reaction. In digestion, the reactions are all catabolic – meaning they are breaking down compounds. However, some enzymes also catalyze anabolic reactions (e.g. polymerase facilitating synthesis of DNA) 13 Active site: The site where the enzyme binds to and changes the substrate. 14 Inhibitor: A molecule that decreases the reaction rate. Competitive Inhibitor It fits into the active site. It blocks the substrate from entering the active site. 15 Allosteric Site: Sites where other molecules, besides the substrate, can bind to and change the enzyme’s ability to do its job (activate or inhibit) Non-competitive Inhibitor: A molecule that fits into an allosteric site and causes the enzyme to work less efficiently or slower. 16 Activator: A molecule that fits into an allosteric site and causes the enzyme to work more efficiently or faster. Cofactor: Bind to an allosteric site and enhance the enzyme’s activity. May be organic or inorganic. Common inorganic cofactors are minerals (e.g. magnesium, zinc, copper). Coenzymes: An organic, nonprotein molecule that assists the enzyme. Includes vitamins (e.g. Vitamin C and Vitamin B3) 17 Each enzyme (1, 2, and 3) have their own job in the process. Enzyme 1 breaks substrate A into substrate B and a side product (the pentagram). Enzyme 2 breaks substrate B into substrate C and a side product (the square). Enzyme 3 breaks substrate C into the end product (the circle) and the last side product (the triangle). In the overall reaction, Substrate B and Substrate C are considered intermediates. and change the shape of the active site. 18 If a cell is using a pathway to break down a molecule into a useful end product, how does the cell decide when it has enough of the end product? It uses a technique called Feedback Inhibition. The end product serves as an inhibitor for the first enzyme in the reaction mechanism. It may compete for the active site (competitive inhibitor), or it may fit into an allosteric site and change the shape of the active site. Is the picture example competitive or non-competitive inhibition? (The end product fits into an allosteric site and it changes the shape of the active site so the substrate no longer binds. It must be an example of non-competitive feedback inhibition) 19 The lock and key model was proposed in 1899 by Emil Fischer. He noticed that each enzyme was very specific to its type of substrate, and used a specific key fitting in a lock as an analogy to how enzymes work. The Induced fit model of enzyme action was proposed in 1958 by Daniel Koshland. It improved on Fischer’s model and helped to explain why some enzymes will catalyze reactions involving more than one type of substrate. 20 Here is a comparison of the two models. In the lower picture, you can see how the active site changes shape to accommodate the substrate. 21 The graph shown on the right shows the production of product over time with and without an enzyme present. As shown, in the absence of an enzyme the reaction proceeds at a steady, relatively, slower rate. With an enzyme present the reaction rate is significantly increased and the end point is reached sooner than an uncatalysed reaction. 22 Because enzymes are proteins, they are very much affected by their environment. The 3D shape of an enzyme determines its function, and that shape is held by different types of chemical bonds that are affected by the conditions. As well, chemical reactions themselves can be influenced by different factors, including concentration and temperature. 23 Enzymes help with both of these. 24 25 As temperature increases, molecules move faster and collide more frequently, increasing the reaction rate. Above a certain temperature, the enzyme denatures as bonds break, causing a decrease in the reaction rate. Increasing temperature increases the speed that the molecules are moving (kinetic energy). As temperature increases, the rate increases. However, enzymes are proteins. Increasing the temperature also increases the vibrational energy of the bonds that hold the enzyme together. At a certain point (denaturation), the bonds stretch enough that they change the shape of the enzyme. At this point, the enzyme is denatured and no longer functions. The reaction rate quickly decreases. 26 The 3D shape of enzymes is influenced by H+ ions (pH) in the solution. Each enzyme has a particular pH that it works best in. At higher or lower pH, the reaction rate decreases. The secondary, tertiary, and quaternary structures of the enzyme (it’s a protein!) are, in part, held together by ionic and hydrogen bonds. These bonds are influenced by the concentration of hydrogen ions (pH). Each enzymes has an optimal pH that it functions best in. At the optimum pH, the concentration of hydrogen ions gives the enzyme its most complementary shape. Outside of that range, the enzyme denatures and the reaction rate decreases as bonds in the enzyme break. 27 More substrate = More collisions At a certain concentration, the active sites of enzymes will all be occupied (saturation point) and rate will no longer increase. Increasing substrate concentration increases the number of potential collisions between the substrate and the enzyme. However, after a certain concentration, any increase will have no effect, since every enzyme is already occupied with a substrate. Each enzyme is working at its maximum rate. We call this the “saturation point” The only way to increase the reaction rate after this saturation point, is to increase the concentration of the enzyme (bottom picture) 28 More enzymes = More collisions At a certain concentration, all substrate is bound to an active sites of the enzymes (saturation point) and rate will no longer increase. Increasing the amount of enzyme also increase the frequency that the enzyme and substrate will collide. As a result, enzyme-substrate complexes form more quickly and the rate of reaction increases. However, there is a limit, as event6ually there will be more enzyme molecules than substrate. Some enzymes become redundant as they won’t have any substrate to bind. Any further increase in enzyme concentration has no further effect on the reaction rate. 29 Increasing the surface area of a solid substrate will increase the number of collisions between the substrate and the enzyme. This will increase the reaction rate, until all active sites are occupied. 30 Reaction rate can be increased with the addition of a cofactor/coenzyme, which activates the enzyme. Competitive inhibitors slow the reaction rate by competing with the substrate for the active site. Non-competitive inhibitors slow the reaction by changing the shape of the active site through allosteric binding. 31