Microorganism Growth & Metabolism (Section 3) - PDF
Document Details
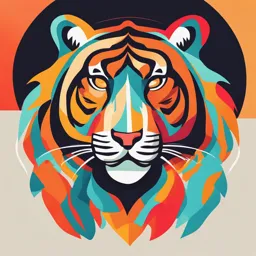
Uploaded by IngeniousCentaur3308
Tags
Related
Summary
This document covers the growth and metabolism of microorganisms, focusing on various aspects such as food sources (energy and biomass), different types of organisms (chemotrophs and phototrophs), oxidation-reduction reactions, electron carriers (NADH). It also explores different metabolic pathways including respiration and fermentation, highlighting the differences, and discussing the role of terminal electron acceptors.
Full Transcript
Section 3: The Growth and Metabolism of Microorganisms Chapters 3, 4, 8, 14, 15 What is food? we need to distinguish two different types of food: 1. food as a source of energy: energy = electrons the electrons can come from three different sources: organic compounds,...
Section 3: The Growth and Metabolism of Microorganisms Chapters 3, 4, 8, 14, 15 What is food? we need to distinguish two different types of food: 1. food as a source of energy: energy = electrons the electrons can come from three different sources: organic compounds, inorganic compounds, or (energized by) light 2. food as a source of biomass to make more cell material: biomass = carbon (also nitrogen, phosphorous, etc.) the carbon can come from different sources: organic material (sugars, etc.) or inorganic carbon (CO2 or HCO3-) What is food? typical amounts of different types of macromolecules in bacterial cells > - need to take in the biomass food to make all this stuff - and enough energy food/electrons to provide power for all the reactions to make all this biomass Physiological Diversity different organisms use different types of energy sources (some can use more than 1) organisms that use chemicals for energy are CHEMOTROPHs use of organic chemicals = chemoorganotroph - use of inorganic chemicals = chemolithotroph Physiological Diversity different organisms use different types of energy sources (some can use more than 1) organisms that use light as their source of energy are PHOTOTROPHS EATING LIGHT Oxidation-Reduction reactions Oxidation-reduction reactions involve the transfer of electrons and are used in the cell to power ATP synthesis - Does not matter whether the source of energy is chemicals, or what kind of chemicals, or if it is light ATP and phosphate bonds ATP is the main energy source used inside cells, but other compounds with phosphate bonds are also used Oxidation-reduction reactions Oxidation-reduction reactions are always coupled electron donors are oxidized electron acceptors are reduced Reduction Potentials different substances have different properties for whether they will donate electrons or accept electrons in redox reactions quantify this as “E” = reduction potential electrons in different substances have different amounts of energy The Redox Tower substances commonly involved in these energy- > related redox reactions in cells have E values that range from -0.6 to +0.8 High = neg positive low = “The Redox Tower”: arrange the substances vertically, with the most negative Es on the top and the most positive Es on the bottom The Redox Tower the electrons want to travel down the tower so, for any pair of substances in a redox reaction, things higher in the tower will donate - electrons, and the substance lower in the tower will accept - electrons moving electrons down the tower releases energy pushing an electron up the tower requires energy nee The Electron Tower the farther apart the E values of a donor-acceptor pair are, the more energy that is released transfer of electrons from glucose to 2 different partners Bigger! at different positions on the to know * don't need tower releases different the numbers amounts of energy NADH: an electron carrier electrons from redox reactions often end up in NAD (or NADP), which is a coenzyme that acts as a diffusible electron carrier NAD: nicotinamide-adenine dinucleotide NADP: NAD-phosphate E = -0.32 V (high on the electron tower and therefore NADH is a good electron donor) acts as a storage molecule - for high energy electrons NADH: an electron carrier electrons are transferred between substrates and products via NADH through the activities of specific enzymes Catabolic Diversity chemotroph = eats chemicals for energy Chemoorganotroph = eats organic chemicals for energy electrons are “harvested” through either aerobic respiration, anaerobic respiration, or fermentation Catabolic Diversity chemotroph = eats chemicals for energy Chemolithotroph = eats inorganic chemicals for energy electrons are “harvested” through either aerobic respiration or anaerobic respiration there is no fermentation of inorganic chemicals Carbohydrate Catabolism ATP is made by different mechanisms in respiration versus fermentation amount of total energy that the cell gets is different fermentation is usually only performed when respiration is not possible (e.g., because there is no electron acceptor available) Carbohydrate Catabolism respiration: fermentation: oxidative phosphorylation substrate-level phosphorylation ATP made by transfer of phosphate from a use proton motive force chemical substrate to ADP to generate ATP - - - Both ATP Synthesize Glycolysis as an illustration Glycolysis Fermentation Respiration Glycolysis as an illustration glycolysis reactions generate ATP, pyruvate and NADH cells can then use pyruvate for RESPIRATION or FERMENTATION respiration happens when there is an electron acceptor available (e.g. O2 or NO3-) fermentation happens when there are no electron acceptors available to the cell Glycolysis reactions glucose is converted to 2 molecules of pyruvate uses 2 ATP but produces 4 ATP and 2 NADH total yield = 2 ATP + 2 NADH Respiration – Citric acid cycle (CAC) the pyruvic acid (pyruvate) is completely - oxidized to CO2 - 1 ATP released during - the citric acid cycle and 5 NADH (= mobile - high energy electrons) 2 pyruvates, so total yield from 1 glucose is 2X this Electron Transport in respiration, this is where most of the energy from the original “food” is converted to ATP energy is released during a series of redox reactions in the Electron Transport Chain (ETC) NADH from the CAC is the e- donor to the ETC pass electrons in steps to a terminal electron acceptor each step releases energy many different terminal electron acceptors can be used e.g. O2, NO3-, Fe3+ Electron Transport - transfer of the electrons from NADH through a series of protein complexes in the cytoplasmic membrane is coupled to the movement of protons (H+) from inside the cell to outside How do cells use the H+ gradient? membrane prevents the H+s from coming back into the cell: higher H+ concentration accumulates outside = the “proton motive force”, which is a source of energy Chemiosmosis H+s move down electrochemical gradient through an ATPase and ADP gets converted to ATP 3H+ per ATP = 34 ATP from 1 glucose when O2 is the terminal electron acceptor Adding it all up total energy yield from 1 glucose for aerobic respiration = 38 ATP TAL 34 of which are generated because of the NADH that feeds the ~ electron transport chain Fermentation Glycolysis fermentation occurs when there is no terminal electron acceptor to allow the ETC to function the cells need to recycle E the NADH back to NAD to allow more glycolysis to Fermentation occur and keep ATP i production going leads to production of “fermentation products” provides much less energy than respiration Fermentation when the cell cannot run an ETC because there is no terminal electron acceptor, they need to recycle the NADH to NAD, otherwise the ATP generating reactions above can’t continue use NADH to reduce the pyruvate produces NAD and “fermentation products” (= waste products that are released from the cell) Fermentation a perfect summary view of fermentation in our example: organic compound was glucose energy-rich compound was what was made in the glycolysis reactions oxidized compound was the pyruvate Fermentation different organisms produce different fermentation products (remember Louis Pasteur) some of our favorite microbial fermentation waste products Aerobic respiration aerobic respiration: O2 is terminal electron acceptor; generates H2O as waste Escherichia coli aerobic respiration NADH provides e- and O2 is reduced to produce H2O Aerobic versus anaerobic respiration aerobic respiration: O2 is terminal electron acceptor; generates H2O as waste anaerobic respiration: something other than O2 (SO42-, Fe3+, NO3-, etc.) is the terminal electron acceptor; generates different waste products Anaerobic Respiration for chemoorganotrophs, this still involves “eating” an organic compound (such as glucose), but the process of using the electrons (NADH) to make the proton motive force is slightly different because O2 is not used at the end of the ETC Anaerobic Respiration less energy released than aerobic because of the E values of these acceptors compared with O2 O2 is down at the bottom of the redox tower so it is the best possible terminal electron acceptor for allowing the release of the most energy electrons from something high on the tower (e.g., glucose) provide different amounts of energy depending where they end up down the tower Anaerobic respiration is common many different compounds can be e- acceptors anoxic environments: freshwater and marine sediments soils, subsurface, etc. just like aerobic respiration it uses an electron transport chain to create a PMF that is then used to make ATP Anaerobic Respiration 3 examples of anaerobic respiration: Denitrification, also known as dissimilative nitrate (NO3-) reduction Sulfate (SO42-) reduction Proton (H+) reduction Escherichia coli aerobic respiration NADH provides e- and O2 reduced to produce H2O E. coli anaerobic respiration with NO3- NO3- used as the terminal e- acceptor NADH provides e- and NO3- reduced to produce NO2- E. coli aerobic vs. anaerobic 8 H+ per NADH 6 H+ per NADH less H+ get pumped (= less PMF and less energy gained) when using NO3- because NO3- is higher up on the redox tower than O2 Sulfate-reducing bacteria sulfate (SO42-) is an abundant compound in the environment, especially the ocean, and it makes a good TEA different reduced S compounds can be produced and released, but often is H2S less energy obtained when using sulfate as the electron acceptor, compared S can exist in many different to oxygen and nitrate oxidation states, and different S sulfate compounds can be used as TEAs ↳ even less of food E/electron nitrate oxygen Sulfate-reducing bacteria ATP synthesis in this case, the source of the electrons is lactate, which get transferred to ferredoxin (Fd, like NADH but a protein) Fd transfers the electrons pass to the ETC to pump H+s and the sulfate is reduced in steps to HS- Proton Reduction Pyrococcus furiosus: a hyperthermophile (optimal T = 100 °C) glycolysis and fermentation of the pyruvate to acetate produces some ATP; also electron transfers to the protein ferredoxin (generates Fdred, instead of making NADH) the Fdred then transfers the electrons back to 2H+ to produce H2 and this also powers pumping of 2 Na+ outside the cell Proton Reduction Pyrococcus furiosus: a hyperthermophile (optimal T = 100 °C) the Na+ gradient then used for ATP synthesis this is an example of an organism using a sodium motive force instead of proton motive force for ATP synthesis; exact same principle, but it’s a Na+ gradient across the membrane instead of H+ Proton Reduction the environment where Pyrococcus likes to live (hot and anoxic) is thought to be like things were like on the early Earth when life first was evolving Pyrococcus is also an early diverging lineage of Archaea so, this simple mechanism of generating an ion gradient across the membrane with a single membrane-bound protein is likely representative of the first respiration mechanisms that evolved Chemolithotrophic metabolism inorganic chemicals as the source of energy includes aerobic and anaerobic respiration (no fermentation) most are autotrophs: source of carbon is CO2 others are heterotrophs: use organic carbon for biosynthesis, which makes them mixotrophs (inorganic chemicals for energy and organic chemicals for carbon) The Calvin cycle autotrophs: conversion of CO2 to organic carbon, also called carbon fixation carbon fixation, by the Calvin cycle, requires ATP and NADH uses a lot of energy: 18 ATP + 12 NADH required to make 1 glucose from 6 CO2 The Calvin cycle key enzyme in CO2 fixation is ribulose bisphosphate carboxylase (RubisCO), which is inhibited by O2 but many autotrophs are aerobes and using O2 for respiration some autotrophs sequester their RubisCO in compartments called carboxysomes – allows concentration of CO2 inside and keeps O2 away Chemolithotrophs different substances have different numbers of electrons available for release and different amounts of energy some use O2 as terminal electron acceptor for aerobic respiration, but others do anaerobic respiration Hydrogen oxidation and methanogenesis Some Archaea oxidize hydrogen (H2) and produce methane, CH4, as a waste product of their respiration Found in freshwater sediments, sewage, animal intestines same process as we saw for previous examples: electrons feed into an ETC to make a PMF, which is used to make ATP in this case the TEA is CO2, which gets reduced to produce CH4 Hydrogen oxidation and methanogenesis Some Archaea produce methane, CH4, as a waste product of their respiration Found in freshwater sediments, sewage, animal intestines stir up the sediments in a swamp while holding a large funnel over top, large amount of methane gets released and collect in the funnel = explosion! of methane metabolic activity pipe vents in landfills are there to prevent build-up of methane and explosions Chemolithotrophs Sulfur Oxidation sulfur can exist in different oxidation states and different bacteria use different forms Common examples : > - Beggiatoa (bacteria) oxidizes S in steps to SO42- and can store intermediate S0 as internal granules > - Sulfolobus (archaea) grows attached to crystalline sulfur, dissolves the crystal and brings S into the cell for metabolism, also releases H2SO4 and so makes the environment very acidic Bioenergetic pathways Sulfur Oxidation sulfur oxiding bacteria and archaea utilize specialized sulfur metabolism enzymes (Sox and Dsr) to take electrons from the S compounds and transfer them to the ETC generates a PMF for ATP synthesis Reverse electron flow these sulfur compounds have less negative E values than NADH, so the cells have to do reverse electron flow (push electrons uphill) to generate NADH for CO2 fixation ↳ force rxn against the energetics - Sneed for Synthesis CO2 reverse electron flow sacrifices some of the energy from the PMF and so the overall yield of ATP ends up being reduced Iron (Fe2+) oxidation (e.g. Acidithiobacillus) at pH 7, Fe2+ is not stable in presence of O2 but is spontaneously oxidized to Fe3+ - at acid pH Fe2+ is stable, so most iron-oxidizing bacteria are acidophiles because their food is only found in acidic places oxidation of Fe2+ happens on the outside of the OM environment Aq. insolubla leads to precipitation of Fe(OH)3 and acidification of environment due to H+ production Iron oxidation (e.g. Acidithiobacillus) periplasmic protein (rusticyanin) takes the e- and feeds it into an ETC for creation of a PMF which is used to make ATP also have to do strongecient reverse electron PStation flow to generate NADH for CO2 fixation Iron oxidation water contaminated with acidic mine waste usually appears “rusty” from the activities of iron-oxidizing prokaryotes get a very low energy yield so they need to metabolize large amount of Fe2+, grow very slowly Fe2+ E value very close to O2 at pH 2 low pl Nitrifying Bacteria: Nitrification - ↳ produces nitrate inorganic nitrogen e- donors are NH3 and NO2- results in production of NO3- Nitrate was only thought to be done in steps by different groups of aerobic organisms that act in sequence (NH3 to NO2- and then NO2- to NO3-) but that changed with discovery of anammox bacteria = anoxic ammonia oxidation convert inorganic to N2 Ammonia Oxidation NH3 oxidized to NO2- > - take electrons from the NH3 and release NO2- - the electrons feed into an ETC which generates a - PMF which is used to synthesize ATP - O2 is e- acceptor - NADH synthesized by Not the efficient - reverse electron flow most ↳ force run to take place Nitrite Oxidation NO2- oxidation to NO3- take electrons from NO2- and release NO3- the electrons feed into an ETC which generates a PMF which is used to synthesize ATP O2 is e- acceptor NADH synthesized by reverse electron flow Anammox Fig 13.28 Brocadia anammoxidans - unusual bacterium that lacks - peptidoglycan and contains membrane compartments inside cell - - S ↳ ladders oxidized reactivity reduced anammoxosomes: membrane-bound compartments with unusual lipids (ladderane lipids) that form a very dense bilayer, which prevents release of toxic reaction products - (N2H4) into the cytoplasm "Hydrocyne" ↳ disrupts metabolic activities [ETC Anammox process uses both NH3 (NH4+ on the figure) and NO2- and produces N2 as the released end-product now realized to be an important part of sewage treatment processing - a lot of the nitrogen material gets released as N2 gas (i.e., less pollution) these bacteria are strict anaerobes (cannot be around O2) but they need to get their NO2- from the aerobic nitrifying bacteria we saw earlier, and so they live in close association~ like with them Sewage in proximity lives in a system ew" exist in NH3-rich habitats where aerobic and anaerobic microenvironments are in close proximity (such as sewage) Phototrophs: hetero versus auto organisms that use light as their source of energy are phototrophs Photoheterotrophs get their Photoautotrophs get carbon from organic compounds their carbon from CO2 (e.g., glucose or pyruvate) Phototrophs - anoxy versus oxy some phototrophs (purple some phototrophs and green bacteria) do not (cyanobacteria) produce produce O2 = anoxygenic O2 = oxygenic because they get their electrons from different sources Phototrophs - anoxy versus oxy anoxygenic phototrophs get their electrons from a variety of different compounds many use sulfur compounds such as H2S or S0 "Great Oxidation Event" oxygenic phototrophs get their electrons from H2O, which results in the release of O2 Capturing light energy light is captured with pigments, which are associated with specific proteins most common and abundant pigment is Chlorophyll a ring structure with Mg in the middle - many double bonds which are important for light absorption Chlorophyll structure is slightly different in different species and the different structures absorb different wavelengths Chlorophyll in plants, algae, and cyanobacteria the chlorophyll absorbs the blue and red light, so the cells look green Bacteriochlorophyll in purple and green phototrophic bacteria (anoxygenic phototrophs) slightly different structure (green and yellow highlighted regions) compared to chlorophyll, so it absorbs different wavelengths Bacteriochlorophyll this pigment allows these bacteria to absorb light outside of our visible range, in the infra-red final colour of the cells is partly because of the chlorophyll or - bacteriochlorophyll but also because of other pigments - - Cellular Photosynthetic Structures the pigments are not floating around free in the cell pigments exist in association with specific proteins these protein-pigment complexes are called photosystems, and the photosystems are embedded in membranes eukaryotes: invaginations of chloroplast inner membrane (= thylakoids) prokaryotes: in the cytoplasmic membrane (or invaginations of CM) Cellular Photosynthetic Structures clusters of algal cells with green chloroplasts visible inside within the chloroplasts are specialized membrane systems called thylakoids Cellular Photosynthetic Structures cyanobacteria also contain membranes inside the cell that resemble the chloroplast thylakoids i n s ~ provide more surface in the cell so they can have more photosystems, absorb more light and get more energy Photosystems and membranes in Purple Bacteria Chromatophores: Lamellae: also from membrane invaginations invaginations of CM that form vesicles in the cytoplasm Reaction centres and antennas electron transfer process for phototrophs begins at the reaction centre (RC) ↳ where ryn's start for phototrophy many also contain antenna or light-harvesting complexes Light harvesting complex #I rxn center (LHCs) that capture light and pass the energy to the RC Like a satellite get t these LHCs allow cells to capture light from a greater surface area of the cell and also from different wavelengths light of Reaction centre structure protein protein Mn center complex protein pigments are buried inside a bunch of proteins ~ need eachother to work... do not work out the other structure of this RC from a purple bacterium = 1988 Nobel prize Phycobilisomes of Cyanobacteria phycobilisomes are the antenna pigment-protein complexes - antenna complex like satellite that absorb light energy and pass it to the RC in cyanobacteria this pigment is what makes some cyanobacteria blue- contain a different pigment green in colour than the reaction centers acts as a large light- can see them in harvesting “antenna” the EM in the RC ↳ don't contain chlorophyll... but chlorphyll is phototrophy but helps - aren't essential to Phycobilisomes of Cyanobacteria phycocyanin → allophycocyanin → chlorophyll a (RC) energy transfer highest absorbing E middle Shoter , further the different components absorb different wavelengths, and the longest energy is passed into the RC RC is essential to phototrophy Anoxygenic phototrophs use different antennas some purple bacteria have 2 different sets of antenna complexes with specific arrangements around the RC with the extra membrane surface area and antenna complexes, the cells can absorb more light and get more energy Anoxygenic phototrophs use different antennas light harvesting chlorosommes GreenSulfur bacteria Slice green sulfur bacteria (GSB) have their antennas in special protein-pigment structures called chlorosomes that are pressed up against the cytoplasmic membrane where the RC is located Chlorosomes of Green Sulfur Bacteria Bag of pigment absorbing light ~ chlorosomes are packed full of pigment ↳ high density of bacteriochlorophyll capture light energy and pass it to the RC in the cyotplasmic membrane very efficient at light capture and GSB can be found in locations with very little light Phototrophs at the bottom of the ocean? “Green sulfur bacteria are common near the sediments of sulfidic aquatic systems and can even carry out photosynthesis in the darkness of the deep ocean by using infrared radiation - emitted from hydrothermal vents” p. 434 points of from light in the darkest the can't see there are phototrophs ocean we Accessory or Antenna Pigments some use different pigments in the antenna complexes Phycobilins (in cyanobacteria) Bacteriochlorophyll (found in purple and green bacteria) Carotenoids (all; also function in S photoprotection) carotenoids are important for -see protection from damage from light energy (photooxidation), light harvesting, and also affect cell colour - Protection light affect cell colors , harvesting , Accessory or Antenna Pigments “purple bacteria” are not always purple - different purple bacteria have different colours because of the differences in the carotenoid pigments they make - - than oxygenic Simpler & Anoxygenic photosynthesis the light absorbed by phototrophs is used to convert low-energy electrons into high-energy electrons the high-energy electrons ETC & Low E then go into an electron high E transport chain, which pumps Rxn Center - No loss protons out of the cell to make ATP (etc.) or gain of e- * Names other than LHC : RC don't matter. in this case the electron transport chain is actually a circle because the electron returns to the RC Anoxygenic photosynthesis e- travels in a circle: starts at the RC and goes through the ETC and then back to the RC cyclic photophosphorylation 1. e- goes in a circle 2. light provides the energy 3. results in phosphorylation of ADP to make ATP Anoxygenic photosynthesis different types of anoxygenic phototrophs use different ETC components purple bacteria have to use REF for NADH synthesis for CO2 fixation because the e- that leaves the RC is lower on electron tower than NADH green sulfur bacteria don’t have the same problem (they use Fd in place of NADH) and the e- that leaves the RC is above Fd on tower autotrophs versus heterotrophs some electrons leave the cycle (to go to NADH or Fd) why they need an external sources of electrons, often 2 sometimes - are stolon : need Goes in cycle some kind of sulfur compound an e donor from an external source to replenish the cycle inorganic organic autotrophs versus heterotrophs Carbon for biomass ↳ some electrons leave the cycle (to go to NADH or Fd) Recap : - Need food forei for biomass some phototrophs use light to make ATP but then use organic carbon for biosynthesis (photoheterotrophs, mixotrophs) Where are these things? > - most anoxygenic phototrophs do not like O2, but they obviously need light so they are found at locations with little or no O2 but where light is still available 7 m deep in a lake where the top is aerobic, but the bottom is anaerobic and contains H2S (gradient) I chemical of the lake above physical properties sulfur spring, the purple bacteria are normally below the surface but can be seen if it is stirred up Where are these things? most anoxygenic phototrophs do not like O2, but they obviously need light so they are found at locations with little or no O2 but where light is still available sometimes get favorable conditions that allow a visible bloom of them on the surface layer Cyanobacteria many different types of cyanobacteria: unicellular, colonial, filamentous Prochlorococcus 1 >.01.1 1 10 50 Chlorophyll a concentration (mg/m3) Prochlorococcus is the most abundant cyanobacterium on the planet (was only discovered in 1980s!) does not contain phycobillisomes so does not have the phycocyanin pigments and looks green as opposed to blue-green Oxygenic Photosynthesis cyanobacteria, algae (and plants) 2 separate but connected light-using reactions (Photosystems I and II) electrons come from H2O and end up in NADH PMF generated by the electron transfer reactions used to make ATP Oxygenic Photosynthesis light energizes the electron twice, once at each photosystem PSII is first, then PSI e- leaves to make NADH Oxygenic Photosynthesis the electron does not end up back in the same place so the process is called noncyclic photophosphorylation anoxygenic: cyclic photophosphorylation The Calvin cycle Phototrophs that use the light energy to power conversion of CO2 to organic carbon are autotrophs = photosynthetic Phototrophs that use organic carbon for biosynthesis are not autotrophs and are not photosynthetic = photoheterotrophs Microbes, CO2, and Climate Change CO2 is an important greenhouse gas reflects long wavelength electromagnetic radiation and prevents heat loss to outer space anthropogenic emissions have caused increases in atmospheric CO2 we need to reduce CO2 emissions and scientists are also trying to find ways to remove CO2 from atmosphere Can microbes solve climate change? one suggestion is to fertilize the ocean to stimulate the CO2 fixation by marine photosynthetic microbes Ocean Iron Fertilization Phototrophs in the ocean need macronutrients N, P, and can’t grow without these BUT many regions are high in N and P but still have little phototroph activity… These are high-nutrient low- >.01.1 1 10 50 chlorophyll (HNLC) regions The reason the chlorophyll concentration is low in some areas John Martin, Moss Landing is because of low micro- Marine Laboratories, nutrients, especially limited for California State University: iron “Give me half a tanker of iron and I’ll give you an ice age.” Ocean Iron Fertilization Between 1993-2005, 12 large-scale ocean iron enrichment experiments were carried out in HNLC regions Blooms of phototrophic microorganisms were observed a iron dump So, is it a good idea to Remote sensing map of ocean chlorophyll in use iron fertilization to North Pacific HNLC region during an iron sequester carbon in the fertilization experiment. deep ocean? Ocean Iron Fertilization It is now widely accepted by scientists that this is a very bad idea! All other cellular material (such as N and P) would also be sequestered and lost, so the environment would become depleted of other nutrients that were originally there Could make the fertilized regions turn anaerobic Plankton blooms can produce toxic by-products the United Nations Banned by Microbes and Climate Change microbes act as the “unseen majority” on Earth microbes can affect climate change, but they will also be affected by climate change, and this is going to be a very important part of what happens to our planet (and us!) Microbial growth Needs for growth: Food for energy (electrons) Food for biomass (organic or inorganic carbon) Other food for biomass - macronutrients: N, P, S, K, Mg) Micronutrients: - trace elements, metals, growth factors - many function as co-factors in enzymes and are required for the enzyme function Microbial growth In addition to availability of the appropriate food (electrons and biomass) and other nutrients, there are multiple key factors that affect growth O2 temperature pH salt concentration pressure O2 and Growth aerobes: aerobic respiration, must have O2 anaerobes: anaerobic metabolism (fermentation or anaerobic resp.), harmed by O2 facultative aerobes: aerobic or anaerobic respiration, better in O2 so more growth in the oxic zone microaerophiles: require a small amount of O2 for aerobic respiration (but a lot is bad) aerotolerant anaerobes: only capable of fermentation, do not perform respiration, but can tolerate O2 O2 and Growth why is oxygen toxic to some bacteria (obligate anaerobes)? even if it is not being used by a cell for respiration, O2 will react with redox compounds in the cell, which can generate toxic oxygen compounds that damage cellular material O2 and Growth why is oxygen toxic to some bacteria? place cells into a drop of H2O2 and see bubbling due to release of O2 due to catalase enzyme aerotolerant organisms have enzymes that deal with the toxic oxygen compounds O2 and Growth working with strict anaerobes is a PITA and requires special lab equipment anaerobic jar glove box to allow handling of cultures Temperature and growth organisms are adapted to a specific temperature range Temperature affects growth in 2 main ways: 1. protein stability and enzyme activity 2. membrane viscosity and stability (which also affects membrane-localized processes such as chemiosmosis and nutrient transport) Cardinal growth temperatures every organism has 3 “cardinal temperatures” different organisms have different CTs organisms will grow at temperatures other than their optimum, but their growth rate is reduced Temperature classes for microbes adaptations for growth in the cold: proteins/enzymes more flexible, membranes stay fluid at colder temperatures adaptations for growth at high temps: enzymes more stable, membranes only fluid at warmer temperatures (remember Archaea with lipid monolayers) Psychrophiles every habitat on earth is occupied by microbes, even permanently frozen locations e.g., ice from permanently frozen seawater near Antarctica - brown colour is from pigmented algae but it also contains prokaryotes water in permanently ice- covered lakes in Antarctica, glaciers Psychrophiles every habitat on earth is occupied by microbes, even permanently frozen locations snow algae - different species can be different colours Thermophiles quite a large diversity of organisms that grow at high temperatures, such as in hot springs superheated hotsprings (Yellowstone Park) immerse a glass microscope slide in the boiling water and microbes grow on it Thermophilic cyanobacteria quite a large diversity of organisms that grow at high temperatures, such as in hot springs thermophilic cyanobacteria growing at the outflow of a hotspring V shape in the growth caused by the differences in temperature as water flows out of the spring (cools faster at the sides so growth can occur there) Microbiology is beautiful! Grand Prismatic Spring, Yellowstone National Park gradients of temperature, pH, nutrient availability, etc. cause the multi- coloured appearance in some environments such as hot springs, where the colours result from the microbes and their metabolic activities How hot is too hot? prokaryotes have much higher range of possible growth temperatures than eukaryotes members of the Archaea have highest temperature limits pH and Growth microbes exist that grow in pHs from 1-10, but the internal cell is usually still near neutral H+ and OH- interfere with hydrogen bonding, protein structure, and the Picrophilus: grows optimally at pH 1, outer surface of cells - so need killed by pH ≥ 4 because its membrane special adaptations to grow in is destabilized without high H+ acidic or basic conditions Salt and growth same principle we saw with temperatures nonhalophile: cannot tolerate much salt (freshwater) halotolerant: can tolerate higher salt (tidal, brackish water) halophiles: require salt (marine) extreme halophiles: require high concentrations of salt (hypersaline water) What does growth mean for prokaryotes? we’ve covered getting energy (organo versus litho versus photo) and material for new cellular material (hetero versus auto) – how does that translate into “growth”? most prokaryote cell division is binary fission: the cell increases in size and then divides into 2 “daughter cells” the rate this happens at can be quantified as the generation time = time to increase from 1 cell to 2 What does growth mean for prokaryotes? many organisms known that do not divide by binary fission What does growth mean for prokaryotes? the growing cell needs to make a new copy of the genome for each cell, so the process of DNA replication and cell division are closely coordinated chromosomes move to the periphery of the cell to be removed from the site of cell division (septum formation) What does growth mean for prokaryotes? cell division proteins make sure each new cell gets a copy of the genome and that the septum is formed in the correct place after DNA has segregated into the two sides What does growth mean for prokaryotes? cell division is performed by a multi-protein machine called the “divisome”, which spans the CM and periplasm Cell Division cells need to synthesize more cell wall to elongate and then divide the 2 new cells (last step of division = septum formation) for bacterial cells with peptidoglycan to do this they must make break open the peptidoglycan layer and insert new material -new PG unit brought into the periplasm -autolysin enzyme breaks the sugar chain -transglycosylase enzyme re-closes it after insertion of the new PG units Transpeptidation Transpeptidase enzymes create the peptide cross-links in the growing peptidoglycan the antibiotic penicillin blocks this reaction bacteria that are growing and therefore making new peptidoglycan are killed by lysis in the presence of penicillin because they cannot re-close the openings they made in their peptidoglycan Growth of microbial populations 1 cell divides into 2 daughter cells, so the number of cells doubles each generation generation time varies between organisms and growth conditions e.g., generation time of 30 minutes: Growth of microbial populations 1 cell divides into 2 daughter cells, so the number of cells doubles each generation generation time varies between organisms and growth conditions in conditions where the generation time is constant, cells are doing exponential growth Phases of culture growth in batch cultures, you see distinct phases of growth as the cells respond to their environment Phases of culture growth Lag Phase: cells adapting to the environmental conditions Exponential (or Log) Phase: all cells dividing at a regular rate Stationary Phase: number of cells stops increasing Death Phase: cells start dying (population decreases) Phases of culture growth Stationary Phase: number of cells stops increasing caused by: 1. an essential nutrient has been used up and/or 2. waste products accumulate that stop growth if stationary phase didn’t happen: starting with 1 bacterial cell and generation time of 30 minutes, culture would be 4000X weight of Earth in 48 hours real world for microbes is often most like being in constant stationary phase