3. NERVOUS SYSTEM.pptx
Document Details
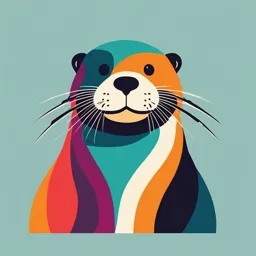
Uploaded by LionheartedSodalite
Tags
Full Transcript
NERVOUS SYSTEM Body’s master controlling and communicating system Three functions Sensory input Gathers information from sensory receptors Integration Processes and interprets sensory input Motor output Activates effector organs to c...
NERVOUS SYSTEM Body’s master controlling and communicating system Three functions Sensory input Gathers information from sensory receptors Integration Processes and interprets sensory input Motor output Activates effector organs to cause a response Central nervous system (CNS) Brain and spinal cord Integrating and command center Interprets sensory input Dictates motor responses The central nervous system contains more than 100 billion neurons. Incoming signals enter this neuron through synapses located mostly on the neuronal dendrites, but also on the cell body. For different types of neurons, there may be only a few hundred or as many as 200,000 such synaptic connections from input fibers. Conversely, the output signal travels by way of a single axon leaving the neuron. Then, this axon has many separate branches to other parts of the nervous system or peripheral body. A special feature of most synapses is that the signal normally passes only in the forward direction, from the axon of a preceding neuron to dendrites on cell membranes of subsequent neurons. This forces the signal to travel in required directions for performing specific nervous functions Peripheral nervous system (PNS) Nerves extending from brain and spinal cord Carry impulses to and from the CNS Two Functional Subdivisions of the PNS Sensory division “afferent division” Nerve fibers conveying impulses to the CNS Somatic afferent fibers convey impulses from the skin, muscles, and joints Visceral afferent fibers convey impulses from visceral organs Motor division , “efferent division” Nerve fibers conveying impulses from the CNS Sensory Part of the Nervous System—Sensory Receptors Most activities of the nervous system are initiated by sensory experiences that excite sensory receptors. These sensory experiences can either cause immediate reactions from the brain, or memories of the experiences can be stored in the brain for minutes, weeks, or years and determine bodily reactions at some future date. This information enters the central nervous system through peripheral nerves and is conducted immediately to multiple sensory areas in (1) the spinal cord at all levels. (2) the reticular substance of the medulla, pons, and mesencephalon of the brain. (3) the cerebellum. (4) the thalamus. (5) areas of the cerebral cortex. Motor Part of the Nervous System— Effectors The most important eventual role of the nervous system is to control the various bodily activities. These activities are collectively called motor functions of the nervous system. muscles and glands are called effectors because they are the actual anatomical structures that perform the functions dictated by the nerve signals. Processing of Information—“Integrative” Function of the Nervous System One of the most important functions of the nervous system is to process incoming information in such a way that appropriate mental and motor responses will occur. More than 99 percent of all sensory information is discarded by the brain as irrelevant and unimportant. When important sensory information excites the mind, it is immediately channeled into proper integrative and motor regions of the brain to cause desired responses. This channeling and processing of information is called the integrative function of the nervous system. Major Levels of Central Nervous System Function Spinal Cord Level Even after the spinal cord has been cut in the high neck region, many highly organized spinal cord functions still occur. For instance, neuronal circuits in the cord can cause (1) walking movements, (2) reflexes that withdraw portions of the body from painful objects, (3) reflexes that stiffen the legs to support the body against gravity, and (4) reflexes that control local blood vessels, gastrointestinal movements, or urinary excretion. In fact, the upper levels of the nervous system often operate not by sending signals directly to the periphery of the body but by sending signals to the control centers of the cord, simply “commanding” the cord centers to perform their functions. Lower Brain or Subcortical Level Many, if not most, of what we call subconscious activities of the body are controlled in the lower areas of the brain— in the medulla, pons, mesencephalon, hypothalamus, thalamus, cerebellum, and basal ganglia. For instance, subconscious control of arterial pressure and respiration is achieved mainly in the medulla and pons. Control of equilibrium is a combined function of the older portions of the cerebellum and the reticular substance of the medulla, pons, and mesencephalon. Feeding reflexes, such as salivation and licking of the lips in response to the taste of food, are controlled by areas in the medulla, pons, mesencephalon, amygdala, and hypothalamus. And many emotional patterns, such as anger, excitement, sexual response, reaction to pain, and reaction to pleasure, can still occur after destruction of much of the cerebral cortex. Higher Brain or Cortical Level the cerebral cortex is an extremely large memory storehouse. The cortex never functions alone but always in association with lower centers of the nervous system. Without the cerebral cortex, the functions of the lower brain centers are often imprecise. The vast storehouse of cortical information usually converts these functions to determinative and precise operations. Finally, the cerebral cortex is essential for most of our thought processes, but it cannot function by itself. In fact, it is the lower brain centers, not the cortex, that initiate wakefulness in the cerebral cortex, thus opening its bank of memories to the thinking machinery of the brain. Thus, each portion of the nervous system performs specific functions. But it is the cortex that opens a world of stored information for use by the mind. Central Nervous System Synapses Information is transmitted in the central nervous system mainly in the form of nerve action potentials, called simply “nerve impulses,” through a succession of neurons, one after another. However, in addition, each impulse (1) may be blocked in its transmission from one neuron to the next, (2) may be changed from a single impulse into repetitive impulses, or (3) may be integrated with impulses from other SYNAPSE Information from one neuron flows to another neuron across a synapse. The synapse is a small gap separating neurons. The synapse consists of: a presynaptic ending that contains neurotransmitters, mitochondria and other cell organelles, a postsynaptic ending that contains receptor sites for neurotransmitters and, a synaptic cleft or space between the presynaptic and postsynaptic endings. It is about 20nm wide ACTION POTENTIAL Appears when region of excitable membrane depolarizes to threshold Steps involved – Membrane depolarization and sodium channel activation – Sodium channel inactivation – Potassium channel activation – Return to normal permeability NERVE IMPULSE Action potential travels along an axon Information passes from presynaptic neuron to postsynaptic cell NEUROTRANSMITTERS. An action potential cannot cross the synaptic cleft between neurones. Instead the nerve impulse is carried by chemicals called neurotransmitters. These chemicals are made by the cell that is sending the impulse (the pre-synaptic neurone) and stored in synaptic vesicles at the end of the axon. The cell that is receiving the nerve impulse (the post-synaptic neurone) has chemical-gated ion channels in its membrane, called neuroreceptors. These have specific binding sites for the neurotransmitters. HOW THE IMPULSE IS TRANSMITTED ACROSS THE SYNAPTIC CLEFT Action potential reaches the presynaptic terminal. Which opens voltage gated calcium channels Influx of calcium occurs. Synaptic vesicles fuse with the membrane(exocytosis). Neurotransmitter are released into synaptic cleft and diffuse to post synaptic terminal. Neurotransmitter binds to neuroreceptor on postsynaptic membrane. Causes Na channels to open and Na flow into postsynaptic membrane. If threshold is reached then action potential is initiated. Neurotransmitter is broken down by specific enzymes into synaptic cleft. Cholinergic synapses Release acetylcholine (ACh) Information flows across synaptic cleft Synaptic delay occurs as calcium influx and neurotransmitter release take appreciable amounts of time ACh broken down – Choline reabsorbed by presynaptic neurons and recycled Synaptic fatigue occurs when stores of ACh are exhausted DIFFERENT TYPES OF SYNAPSES Fundamental classification Working classification Anatomical classification Fundamental classification There are two fundamentally different types of synapse: 1.chemical synapse, {the presynaptic neuron releases a chemical called a neurotransmitter that binds to receptors located in the postsynaptic cell, usually embedded in the plasma membrane. Binding of the neurotransmitter to a receptor can affect the postsynaptic cell in a wide variety of ways.} 2.electrical synapse, {the presynaptic and postsynaptic cell membranes are connected by channels that are capable of passing electrical current, causing voltage changes in the presynaptic cell to induce voltage changes in the postsynaptic cell.} Working classification 1.Excitatory ion channel synapses - 2. inhibitory ion channels – 3.Non channel synapses – 4.Neuromuscular junctions - 5.Electrical synapses - 1.Excitatory ion channel synapses - neuroreceptors are Na+ channels. When Na+ channels open, local depolarisaition occurs, if threshold is reached then action potential is initated The resting membrane potential everywhere in the soma is −65 millivolts. The rapid influx of positively charged sodium ions to the interior neutralizes part of the negativity of the resting membrane potential. This positive increase in voltage above the normal resting neuronal potential— that is, to a less negative value—is called the excitatory postsynaptic potential (or EPSP) because if this potential rises high enough in the positive direction, it will elicit an action potential in the postsynaptic neuron, thus exciting it. Discharge of a single presynaptic terminal can never increase the neuronal potential from −65 millivolts all the way up to −45 millivolts. An increase of this magnitude requires simultaneous discharge of many terminals—about 40 to 80 for the usual anterior motor neuron—at the same time or in rapid succession. This occurs by a process called summation. SPATIAL SUMMATION: This effect of summing simultaneous postsynaptic potentials by activating multiple terminals on widely spaced areas of the neuronal membrane is called spatial summation. TEMPORAL SUMMATION: Each time a presynaptic terminal fires, the released transmitter substance opens the membrane channels for at most a millisecond or so. But the changed postsynaptic potential lasts up to 15 milliseconds after the synaptic membrane channels have already closed. Therefore, a second opening of the same channels can increase the postsynaptic potential to a still greater level, and the more rapid the rate of stimulation, the greater the postsynaptic potential becomes. Thus, successive discharges from a single presynaptic terminal, if they occur rapidly enough, can add to one another; that is, they can “summate.” This type of summation is called temporal summation. 2.inhibitory ion channels – neuroreceptors are Cl- channels. When Cl- channels open, hyperpolarisation occurs, making action potential less likely. Opening the chloride channels will allow negatively charged chloride ions to move from the extracellular fluid to the interior, which will make the interior membrane potential more negative than normal, approaching the −70 millivolt level. Opening potassium channels will allow positively charged potassium ions to move to the exterior, and this will also make the interior membrane potential more negative than usual. Thus, both chloride influx and potassium efflux increase the degree of intracellular negativity, which is called hyperpolarization. This inhibits the neuron because the membrane potential is even more negative than the normal intracellular potential. Therefore, an increase in negativity beyond the normal resting membrane potential level is called an inhibitory postsynaptic potential (IPSP). 3.Non channel synapses – neuroreceptors are membrane-bound enzymes. When activated, they catalyse the 'messenger chemical', which in turn can affect the sensitivity of the ion channel receptors in the cell. 4.Neuromuscular junctions – synapses formed between motor neurones and muscle cells. Always use the neurotransmitter acetylcholine, and are always excitatory 5.Electrical synapses – the membranes of the two cells actually touch and they share proteins. The action potential can pass directly from one membrane to the next. Anatomical classification 1. Axosomatic synapse: In this, axon of one neuron ends on soma of another neuron. 2. Axodendritic synapse: Axon of first neuron terminates on dendrite of next neuron. 3. Axoaxonic synapse: The axon of one neuron terminates on axon of another neuron. 4. DENDRODENDRITIC sss GRAND POSTSYNAPTIC POTENTIAL One neurone can have thousands of synapses on its body and dendrons. So it has many inputs, but only one output. The output through the axon is called the Grand Postsynaptic Potential (GPP) and is the sum of all the excitatory and inhibitory potentials from all that cell’s synapses. If there are more excitatory potentials than inhibitory ones then there will be a GPP, and the neurone will “fire”, but if there are more inhibitory potentials than excitatory ones then there will not be a GPP and the neurone will not fire. “Facilitation” of Neurons Often the summated postsynaptic potential is excitatory but has not risen high enough to reach the threshold for firing by the postsynaptic neuron. When this happens, the neuron is said to be facilitated. That is, its membrane potential is nearer the threshold for firing than normal, but not yet at the firing level. Consequently, another excitatory signal entering the neuron from some other source can then excite the neuron very easily. Diffuse signals in the nervous system often do facilitate large groups of neurons so that they can respond quickly and easily to signals arriving from other sources Synaptic Delay During transmission of a neuronal signal from a presynaptic neuron to a postsynaptic neuron, a certain amount of time is consumed in the process of (1) discharge of the transmitter substance by the presynaptic terminal, (2) diffusion of the transmitter to the postsynaptic neuronal membrane, (3) action of the transmitter on the membrane receptor, (4) action of the receptor to increase the membrane permeability, and (5) inward diffusion of sodium to raise the excitatory postsynaptic potential to a high enough level to elicit an action potential. The minimal period of time required for all these events to take place, even when large numbers of excitatory synapses are stimulated simultaneously, is about 0.5 millisecond. This is called the synaptic delay. Fatigue of Synaptic Transmission When excitatory synapses are repetitively stimulated at a rapid rate, the number of discharges by the postsynaptic neuron is at first very great, but the firing rate becomes progressively less in succeeding milliseconds or seconds. This is called fatigue of synaptic transmission. Fatigue is an exceedingly important characteristic of synaptic function because when areas of the nervous system become overexcited, fatigue causes them to lose this excess excitability after a while. For example, fatigue is probably the most important means by which the excess excitability of the brain during an epileptic seizure is finally subdued so that the seizure ceases. Thus, the development of fatigue is a protective mechanism against excess neuronal activity. The mechanism of fatigue is mainly exhaustion or partial exhaustion of the stores of transmitter substance in the presynaptic terminals. The excitatory terminals on many neurons can store enough excitatory transmitter to cause only about 10,000 action potentials, and the transmitter can be exhausted in only a few seconds to a few minutes of rapid stimulation. Part of the fatigue process probably results from two other factors as well: (1) progressive inactivation of many of the postsynaptic membrane receptors and (2) slow development of abnormal concentrations of ions inside the postsynaptic neuronal cell. Effect of Acidosis or Alkalosis on Synaptic Transmission Most neurons are highly responsive to changes in pH of the surrounding interstitial fluids. Normally, alkalosis greatly increases neuronal excitability. For instance, a rise in arterial blood pH from the 7.4 norm to 7.8 to 8.0 often causes cerebral epileptic seizures because of increased excitability of some or all of the cerebral neurons. Conversely, acidosis greatly depresses neuronal activity; a fall in pH from 7.4 to below 7.0 usually causes a comatose state. For instance, in very severe diabetic or uremic acidosis, coma virtually always develops. Effect of Hypoxia on Synaptic Transmission Neuronal excitability is also highly dependent on an adequate supply of oxygen. Cessation of oxygen for only a few seconds can cause complete inexcitability of some neurons. This is observed when the brain’s blood flow is temporarily interrupted because within 3 to 7 seconds, the person becomes unconscious. Effect of Drugs on Synaptic Transmission Many drugs are known to increase the excitability of neurons, and others are known to decrease excitability. For instance, caffeine, theophylline, and theobromine, which are found in coffee, tea, and cocoa, respectively, all increase neuronal excitability, presumably by reducing the threshold for excitation of neurons. Most anesthetics increase the neuronal membrane threshold for excitation and thereby decrease synaptic transmission at many points in the nervous system.