Iron Deficiency Anemia, ACD, & Abnormal Heme Synthesis - 3- Iron deficiency anemia + ACD+abnormal heme synthesis with notes.PDF
Document Details
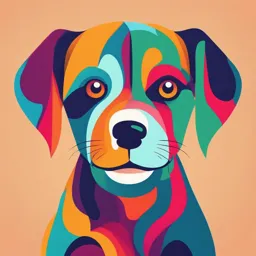
Uploaded by BestKnownPine
Dr. Johnny Amer
Tags
Summary
This document provides information on different types of anemia, including iron deficiency anemia, anemia of chronic disease (ACD), and issues related to abnormal heme synthesis. It details various factors, diagrams, and associated notes.
Full Transcript
1- Iron deficiency anemia 2- Anemia of Chronic disorders 3- Sideroblastic Anemia 4- Thalassemia 5- Lead poisoning جوين عامر.د Sites of defective hemoglobin synthesis that can result in Microcytic Hypochromic anemia. Note (i) Inflammato...
1- Iron deficiency anemia 2- Anemia of Chronic disorders 3- Sideroblastic Anemia 4- Thalassemia 5- Lead poisoning جوين عامر.د Sites of defective hemoglobin synthesis that can result in Microcytic Hypochromic anemia. Note (i) Inflammatory cytokines suppress the proliferation of erythroid precursors in the bone marrow; (ii) inflammatory cytokines inhibit the release of erythropoietin (EPO) from the kidney (iii) the survival of circulating red cells is shortened Iron deficiency anemia According to the World Research suggests that It also suggests that as Health Organization as many as 80 percent of many as 30 percent of (WHO), iron deficiency people in the world people have anemia due is the top nutritional don't have enough iron to prolonged iron disorder in the world. in their bodies. deficiency. Iron deficiency is the It is the most important This appearance is most common cause of cause of a microcytic caused by a defect in anemia in every country hypochromic anemia. hemoglobin synthesis. of the world. Distribution of Iron Iron-containing compounds in the body are one of two types: Functional compound (hemoglobin, myoglobin, cytochrome, catalase) Compounds that serve as transport (transferrin) or storage (Ferritin and hemosidrein) Iron is found in erythrocytes, macrophages, hepatocytes and enterocytes. The macrophages recycle about 20 times more iron than is absorbed in the gut. This iron recycling provides most of the marrow's daily iron requirement ( about 85%) for erythropoiesis Note Cytochromes are iron containing hemeproteins central to which are heme groups that are primarily responsible for the generation of ATP via electron transport Catalase is a tetramer of four polypeptide chains, each over 500 amino acids long. It contains four porphyrin heme (iron) groups that allow the enzyme to react with the hydrogen peroxide. A tiny fraction of body iron is in labile pool including nontransferrin-bound iron (NTBI) in the plasma and labile iron pool (LIP) within erythrocytes. Both labile-iron forms do not bind to any dedicated proteins and are potentially toxic (1). These free irons involve in electron transfer in the Fenton reaction, resulting in the generation of the hydroxyl radical, one of reactive oxygen species (ROS). Ideally, normal iron balance: amount absorbed = amount lost from the body. The total iron concentration in the body is 40–50 mg of iron/kg of body weight. Men usually have higher amounts than women. Note The daily iron cycle. Most iron is recycled from the erythrocytes to the bone marrow. Only a small amount of iron is lost from the body through loss of iron containing cells. To maintain iron balance, a similar amount of iron is absorbed from the duodenum.. و أحد أجزاء القناة الهضمية وأول جزء من األمعاء الدقيقة يصل بين المعدة والصائم تنفتح في القطعة الثانية منه كال من. سم وله أربعة قطع تشبه المضلع30-25 طوله. ويفصله عن المعدة البواب،القناتين الصفراوية والبنكرياسية Mucous membranes line the digestive, respiratory, and reproductive tracts and are the primary barrier between the external world and the interior of the body Desquamation, commonly called skin peeling, is the shedding of the outermost membrane or layer of a tissue, such as the skin. Body Iron transport The transport and storage of iron is largely mediated by three proteins: transferrin, the transferrin receptor 1 (TfR1) and ferritin. Transferrin can contain up to two atoms of iron. It delivers iron to tissues that have transferrin receptors, especially erythroblasts in the bone marrow which incorporate the iron into hemoglobin, the transferrin is then reutilized. At the end of their life, red cells are broken down in the macrophages and the iron is released from hemoglobin, enters the plasma and provides most of the iron on transferrin. Only a small proportion of plasma transferrin iron comes from dietary iron, absorbed through the intestine. Some iron is stored in the macrophages as ferritin and hemosiderin The levels of ferritin and TfRl are linked to iron status so that iron overload causes a rise in tissue ferritin and a fall in TfRl, whereas in iron deficiency, ferritin is low and TfRl increased. When plasma iron is raised and transferrin is saturated and the amount of iron transferred to cells (e,g, those of the liver, bone marrow, pancreas and heart) is increased and this is the basis of the pathological changes associated with iron overload. Note Hemosiderin or haemosiderin is an iron-storage complex. It is only found within cells (as opposed to circulating in blood) and appears to be a complex of ferritin, denatured ferritin and other material. Ferritin is primarily an intracellular protein, but small amounts enter the blood through active secretion or cell lysis. The amount of circulating ferritin parallels the concentration of storage iron in the body. Therefore, serum ferritin concentration is used as an index of iron stores: 1 ng/mL of serum ferritin indicates about 8 mg of storage iron. Ferritin- Spherical shaped protein is a water - soluble protein – iron complex of molecular weight 465 000. Ferritin without iron in its shell is called apoferritin It contains up to 20% of its weight as iron. Each molecule of apoferritin may bind up to 4000 – 5000 atoms of iron. Ferritin stores iron in a non-toxic form. Ferritin has a ferroxidase activity which involves the conversion of iron from the ferrous (Fe2+) to ferric (Fe 3+) forms. This prevents the formation of free radicals via the Fenton Reaction. Hemosiderin is an insoluble protein – iron (liver Kupffer cells and BM macrographs) complex of varying composition containing approximately 37% iron by weight. It is derived from partial lysosomal digestion of aggregates of ferritin molecules and is visible in macrophages and other cells by light microscopy after staining by Perls ’ (Prussian blue) reaction. Iron in ferritin and haemosiderin is in the ferric form. Absorption of Iron #1 what goes in must come out Dietary iron Non-heme iron (Fe+3 ) present in vegetables. exists in two Heme-iron (Fe+2 ) present in red forms: Note: Nonheme iron is the most common form ingested meats. worldwide; heme iron is more common in Western countries (providing about 10–15% of daily iron requirements). Heme iron is more readily absorbed than non-heme iron Absorption of Iron DcytB the proton coupled folate transporter (PCFT) Note Non-heme Iron Gastric acid solubilizes this form of iron at the luminal border of enterocytes. Fe+3 is converted (reduced) to Fe+2 via duodenal cytochrome- B reductase (DcytB). Ferrous iron is then transported across the luminal (apical) membrane of enterocytes via divalent metal transporter 1 (DMT1). Heme iron The low pH and proteolytic enzymes of the stomach act to release heme from hemoproteins. Heme uptake by enterocytes is most likely via the heme carrier protein 1 (HCP1) together with the proton coupled folate transporter (PCFT) in receptor- mediated endocytosis. The major physiological roles of PCFTs are in mediating the intestinal absorption of folate (Vitamin B9), and its delivery to the central nervous system. Once inside the cell, iron is released from heme by heme oxygenase. The iron then enters the same iron pool as the nonheme iron Heme is split from globin in the intestine. Then transported to the enterocytes via heme carrier protein (HCP1) Inside the enterocytes, heme is catabolized by heme oxygenase and release Fe+2 Note In the enterocytes, the iron can be stored as ferritin or transported across the basolateral membrane into the plasma via ferroportin 1. Export is facilitated by the ferroxidase hephaestin which oxidizes Fe+2 to Fe+3 , the form of iron required for binding to apotransferrin. Note Hephaestin is a copper-containing ferroxidase that requires adequate amounts of copper for its function hephaestin (a homologue of the plasma protein ceruloplasmin). Thus, it is not surprising that copper deficiency is associated with abnormal iron metabolism. Export of iron from non- intestinal cells, including macrophages, requires ceruloplasmin, which also converts Fe+ + to Fe+ + + for binding to transferrin. Note Hepcidin is a protein that in humans is encoded by the HAMP gene. Hepcidin is a key regulator of the entry of iron into the circulation in mammals. In states in which the hepcidin level is abnormally high such as inflammation, serum iron falls due to iron trapping within macrophages and liver cells and decreased gut iron absorption. This typically leads to anemia due to an inadequate amount of serum iron being available for developing red cells. When the hepcidin level is abnormally low such as in hemochromatosis, iron overload occurs due to excessive ferroportin mediated iron influx. Non-heme Iron Gastric acid solubilizes this form of iron at the luminal border of enterocytes. Fe+3 is converted (reduced) to Fe+2 via duodenal cytochrome- B reductase (DcytB). Ferrous iron is then transported across the luminal (apical) membrane of enterocytes via divalent metal transporter 1 (DMT1). Heme iron Heme is split from globin in the intestine. Then transported to the enterocytes via heme carrier protein (HCP1) Inside the enterocytes, heme is catabolized by heme oxygenase and release Fe+2 Note Cytochrome-b5 reductase (also known as methemoglobin reductase) is a NADH-dependent enzyme that converts methemoglobin to hemoglobin. It contains FAD and catalyzes the reaction: NADH + H+ + 2 ferricytochrome b5 = NAD+ + 2 ferrocytochrome b5 The low pH and proteolytic enzymes of the stomach act to release heme from hemoproteins. Heme uptake by enterocytes is most likely via the heme carrier protein 1 (HCP1) together with the proton coupled folate transporter (PCFT) in receptor-mediated endocytosis. Once inside the cell, iron is released from heme by heme oxygenase. In the enterocytes, the iron can be stored as ferritin or transported across the basolateral membrane into the plasma via ferroportin 1. Export is facilitated by the ferroxidase hephaestin which oxidizes Fe+2 to Fe+3 , the form of iron required for binding to apotransferrin. The efficiency of intestinal absorption of iron increases in response to accelerated erythropoietic activity. Thus, bleeding, hemolysis or hypoxia results in accelerated erythrocytes production and enhanced absorption of iron. Iron Transport Transferrin (apotransferrin): Half life: 18 days and synthesized in the liver It is not lost in delivering iron to cells but returns to plasma and reused. Enough transferrin is present in plasma to bind 250 – 435 µg iron per dL of plasma (Total Iron Binding Capacity- TIBC) Serum iron concentration is about 70 – 200 µg/dL. Therefore, transferrin is about 1/3 saturated with iron (% transferrin saturation) Transferrin Receptors: Number of transferrin receptors expressed per cell (normoblast) is proportional to cellular iron requirement. Cells release their transferrin receptors through proteolytic cleavage as they mature. These cleaved receptors referred to as serum or soluble transferrin receptors (with increased erythropoiesis, soluble transferrin receptors in the plasma increase). Note Proteins often undergo proteolytic processing by specific proteolytic enzymes (proteases/peptidases) before final maturation of the protein The mechanism of iron uptake by TfR involves a selective affinity of the receptor for diferric transferrin.1 The TfR-transferrin complex is internalized and cycled through endosomes, where the iron is released to the cytosol, with the TfR-apotransferrin complex returning to the extracellular surface (Fig. 1). At the surface the apotransferrin dissociates to be replaced by diferrictransferrin, repeating the cycle. Cellular iron supply and storage Note (1) Iron binds to apotransferrin in the plasma forming monoferric or diferric transferrin. (2) Transferrin binds to transferrin receptors on the cell surface (3). The transferrin–receptor complex enters the cell and the cell membrane fuses forming an endosome (4). An acidic vesicle fuses with the endosome (5) which results in the release of iron (6). The apotransferrin–receptor complex is transported back to the cell surface (7) where the apotransferrin is released from the receptor and enters the plasma for reutilization (8) Iron Studies Low serum levels: Iron deficiency anemia The liver produces more transferrin, presumably attempting to maximize use of the little iron that is available. Transferrin saturation < 16% is an indication of IDA (iron deficiency anemia) while > 50% is an indication of iron overload or hemochromatosis. Ferritin level: It is an indication of iron stores in the body. Decreased serum ferritin level can be the first indication of developing IDA. Care should be used in interpreting serum ferritin levels because it is an acute phase reactant (nonspecific increases can be seen in malignancy, infections and liver diseases) thus ID can be masked in these conditions. Serum transferrin receptor is inversely proportional to the amount of body iron. Also, it is not affected by concurrent disease states. Zinc protoporphyrin (ZPP): zinc is incorporated to protoporphyrin instead of iron when iron is not available. Note Acute-phase proteins are a class of proteins whose plasma concentrations increase (positive acute-phase proteins) or decrease (negative acute-phase proteins) in response to inflammation. This response is called the acute-phase reaction (also called acute-phase response). Inflammatory cells and red blood cells In response to injury, local inflammatory cells (neutrophil granulocytes and macrophages) secrete a number of cytokines into the bloodstream, most notable of which are the interleukins IL1, IL6 and IL8, and TNFα. The liver responds by producing a large number of acute-phase reactants Taken together with serum iron and percent transferrin saturation clinicians usually perform this test when they are concerned about anemia, iron deficiency or iron deficiency anemia. However, because the liver produces transferrin, alterations in function (such as cirrhosis, hepatitis, or liver failure) must be considered when performing this test. It can also be an indirect test of liver function, but is rarely used for this purpose Iron Studies-2 Taken together with serum iron and percent transferrin saturation clinicians usually perform this test when they are concerned about anemia, iron deficiency or iron deficiency anemia. However, because the liver produces transferrin, alterations in function (such as cirrhosis, hepatitis, or liver failure) must be considered when performing this test. It can also be an indirect test of liver function, but is rarely used for this purpose Note Patients with cirrhosis frequently present with high serum ferritin and low transferrin concentrations, reflecting impaired liver function and inflammation. Serum iron concentration has a diurnal variation with highest levels in the morning, so sampling time is an important consideration Normal Physiological Factors That Increase Iron Requirements Menstruation: menstruating females must absorb twice the amount of normal absorption ( about 2mg of iron daily). Pregnancy: the daily iron requirement during pregnancy is about 2-3 mg. Fetus obtains iron from maternal stores, increase maternal blood volume and iron loss at delivery due to bleeding. Infancy/children: rapid growth in infancy (iron is needed for tissue growth). Iron deficient nutrition (milk) during the first 4-5 months of life required adequate iron stores in the fetus. Iron requirements are also high in childhood. Note Menstruation The average daily iron loss in menstruating females is twice that of their male counterparts. To maintain total body iron balance, menstruating females must absorb about 2 mg of iron daily. National statistics from the third National Health and Nutrition Examination Survey from 1988 to 1994 (NHANES III) reveal that 9–11% of adolescent and young adult females are in an iron deficient state and 2–5% have IDA. Pregnancy The daily iron requirement during pregnancy is about 3.4 mg; if spread out as a daily average over the three trimesters, it would be about 1,000 mg per pregnancy. The fetus accumulates of iron from maternal stores via the placenta; added to this is the iron requirement for increased maternal blood volume and iron loss at delivery due to bleeding. Thus, a single pregnancy without supplemental iron could exhaust iron stores. Note Infancy/Children In infancy, rapid growth of body size and hemoglobin mass requires more iron in proportion to food intake than at any other time of life. During the first six months of life, an infant synthesizes of new hemoglobin. In addition, iron is needed for tissue growth. At birth, normal iron stores of 30 mg are adequate to see the infant through the first 4 to 5 months of life but can be depleted quickly in an infant on an iron-deficient, milk-only diet. Premature infants are at an even higher risk of rapid iron depletion because much of the placental transfer of iron occurs in the last trimester of pregnancy, and they have a faster rate of postnatal growth than full-term infants.17 It is recommended that full-term infants begin iron supplements no later than 4 months of age and that low-birth- weight infants begin no later than two months of age.19 Iron requirements are also high in childhood, especially in 1–3 year olds. Data from NHANES III revealed that in 1-yearolds, the prevalence of ID is 13%,18 yet 1–3 year olds, especially those from low income families, have the lowest daily iron intake of any age group.20 A recent study of 1–3-year-old children from urban lower- and middle- class socioeconomic groups showed that 35% had evidence of iron insufficiency in various stages. Of these, 10% had overt IDA.21 Iron is a mineral that the body needs for : 1. Growth and development 2. Make hemoglobin in red blood cells that carries oxygen from the lungs to all parts of the body, and myoglobin, a protein that provides oxygen to muscles 3. Some hormones Recommended daily amounts of iron uptake, in milligram(mg) Male Female Birth to 6 month 0.27 mg 0.27 mg 7 to 12 month 11 mg 11 mg Overall assessment on the prevalence of anemia in Palestinian infant population 29.1% were anemic (HGB less than 11g/dl): 21.1% had mid anemia, 7.6% with moderate anemia while 0.2% had severe anemia. Distribution of Anemia Among Population 7.6% 70.9% 21.3% 0.2% 0% 20% 40% 60% 80% 100% Non-Anemic Mild Moderate Severe Amer J, Anemia 2022 Inverse correlation between body weight and anemia severity among infants. AVERAGE WEIGHT (GRAMS) 9.8 9.8 9.6 9.6 9.3 9.4 9.2 9 NON-ANEMIC MILD P13 0-13 90% >13 Amer J, Anemia 2022 Distribution of Anemia Among Population 7.6% 70.9% 21.3% 0.2% Mentzer Index Among Anemic 0% 50% 100% Population Non-Anemic Mild 100% Moderate Severe 80% 60% 83.2% 40% 20% 16.8% 0% > 13 Mentzer < 13 index Amer J, Anemia 2022 Distribution of Anemia Among Population 7.6% 70.9% 21.3% 0.2% 0% 50% 100% MENTZER INDEX AMONG Non-Anemic Mild Moderate Severe NON-ANEMIC POPULATION 100% More Likely Iron 80% Deficiency Anemia, 60% 93.1% More Likely Beta- 40% Thalassemia, 6.9% 20% 0% Mentzer Mentzer Amer J, Anemia 2022 index >13 index