Next Generation Sequencing (NGS) PDF
Document Details
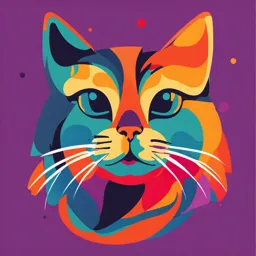
Uploaded by WinningHoneysuckle
UCLan
Dr Temba Mudariki
Tags
Summary
This document covers Next Generation Sequencing (NGS), a suite of modern technologies for rapid DNA and RNA sequencing. It details the history, methodologies, and applications of NGS in science and medicine, including aspects of data analysis and its uses in cancer genomics and personalized medicine.
Full Transcript
Next Generation Sequencing (NGS) Dr Temba Mudariki Learning Outcomes 1. Understand the Basics of NGS Technology 2. Comprehend NGS Workflow 3. Recognize NGS Modalities 4. Explore NGS Applications 5. Analyse NGS Data and Understand Its Challenges 6. Evaluate Ethical and Societal Consideratio...
Next Generation Sequencing (NGS) Dr Temba Mudariki Learning Outcomes 1. Understand the Basics of NGS Technology 2. Comprehend NGS Workflow 3. Recognize NGS Modalities 4. Explore NGS Applications 5. Analyse NGS Data and Understand Its Challenges 6. Evaluate Ethical and Societal Considerations 7. Assess Future Directions The Dawn of Sequencing Early Work Pioneering Techniques- Frederick Sanger's methods for sequencing proteins laid the groundwork for DNA sequencing. Foundational Developments- Sanger's protein sequencing work was critical in building the conceptual framework for nucleotide sequencing. Sanger Sequencing Innovation in 1977- Introduction of chain-termination (dideoxy) sequencing. Nobel Prize Achievement- Sanger's method was groundbreaking and earned him the Nobel Prize. Decades of Dominance- Sanger sequencing set the standard for DNA sequencing for over 30 years. The Age of Sanger Sequencing Manual Sequencing Labor-Intensive Methods: Early Sanger sequencing required meticulous manual effort. Radiolabel Detection: Utilized radiolabelled nucleotides for visualizing DNA fragments. Automated Sequencing Technological Leap: The 1980s saw advancements in automation and the introduction of fluorescent dyes. Genomic Milestones: These improvements permitted the sequencing of complete genomes, including the Human Genome Project initiated in 1990. The Advent of Next Generation Sequencing (NGS) Early 2000s Technology Shift Massive Parallel Sequencing: NGS technologies allowed simultaneous sequencing of millions of DNA fragments. Cost & Speed Revolution: Dramatic reductions in both the cost and time required for DNA sequencing. 454 Sequencing 454 Life Sciences Innovation: Launched one of the first NGS platforms with pyrosequencing technology in 2005. The Advent of Next Generation Sequencing (NGS) Illumina Sequencing High-Throughput Leader: Shortly after 454, Illumina released its sequencing platform, which quickly became a market leader. Reversible Terminator Method: Known for its high-throughput capabilities and accuracy. SOLiD Sequencing Applied Biosystems' Contribution: Developed the SOLiD system, which utilized a unique method of sequencing by ligation and two-base encoding. Expansion of NGS Ion Torrent Hydrogen Ion Detection: Life Technologies introduced a faster, economical sequencing method by detecting hydrogen ions. Speed & Cost Efficiency: Ion Torrent technology emphasized a quicker, more affordable approach to sequencing. Oxford Nanopore Long-Read Capability: Enabled the sequencing of long DNA strands in real-time. Electrical Conductivity Measurement: Utilized a novel approach by measuring changes in conductivity as DNA passes through a nanopore. The Modern Era and Beyond Single-Molecule Sequencing Direct Observation: Advances in single-molecule sequencing allow the direct observation of DNA synthesis without prior amplification. Synthetic Biology and CRISPR Genome Engineering: Sequencing is now used in tandem with synthetic biology and CRISPR, pushing the boundaries of genome editing. Portable Devices In-Field Sequencing: Devices like the MinION make sequencing accessible outside traditional laboratory settings. Rapid Diagnostics: Miniaturization paves the way for immediate diagnostic applications, from remote locations to clinical settings. Impact on Science and Medicine Human Genome Project Milestone Completion: The 2003 completion of the Human Genome Project was a landmark achievement but highlighted the need for faster, cheaper methods. Genomic Medicine Routine Diagnostics and Treatment: NGS has revolutionized genomic medicine, allowing for comprehensive genome and exome sequencing in clinical settings. Population Genomics Insights into Diversity and Disease: Sequencing at the population level provides valuable data on human variation, evolutionary patterns, and disease markers. Summary of Future Directions Cost and Speed Affordability Goal: Aiming to achieve and surpass the '$1,000 genome' milestone. Technological Advances: Ongoing efforts to further decrease costs and increase sequencing speed. Integration of AI Complex Data Analysis: Leveraging AI and machine learning to manage and interpret the vast amounts of data generated by sequencing. Introduction to Next Generation Sequencing (NGS) What is Next Generation Sequencing (NGS)? Definition: A suite of state-of-the-art technologies for rapid DNA and RNA sequencing Advantages Over Sanger Sequencing: Increased speed, reduced cost, higher throughput Key Features of NGS High-Throughput: Sequences millions to billions of fragments simultaneously Scalability: Suitable for both small and large-scale sequencing projects Digital Output: Generates digital data for analysis Reduced Cost & Time: More accessible than ever before Components of NGS 1. Library Preparation: Fragmented DNA or cDNA with attached adapters 2. Amplification: Enrichment of DNA quantity 3. Sequencing: Either by synthesis or ligation 4. Data Analysis: Bioinformatics for read alignment and variant identification. Overview of NGS 1. Sample Preparation: DNA extraction and fragmentation, RNA reverse transcription 2. Library Construction: Attaching adapters to DNA fragments 3. Cluster Generation: Amplification of DNA on a flow cell 4. Sequencing: Incorporation of fluorescent nucleotides and imaging 5. Data Analysis: From raw data to variant calls NGS vs Sanger Sequencing Throughput: NGS vastly outperforms in parallel sequencing capacity Read Length: Sanger offers longer reads; NGS has shorter but more numerous reads Cost: NGS is more economical for large-scale projects Accuracy: Sanger remains the gold standard for precision in longer sequences Application of NGS Genomics: Genome sequencing, discovering SNPs, and genotyping Transcriptomics: RNA-Seq for expression profiling and new transcripts Epigenomics: Mapping DNA methylation and chromatin structure Metagenomics: Analyzing environmental microbial communities Clinical Diagnostics: Identifying genetic disease markers, advancing personalized medicine Sample Preparation in NGS Workflow Purpose Objective: To procure high-quality nucleic acids for sequencing. Process Extraction: Isolation of DNA/RNA via chemical or mechanical techniques. Quantification & Quality Assessment: Using spectrophotometry or fluorometry to determine purity and concentration. Fragmentation: Breaking down DNA to optimal sizes for sequencing. Library Construction for NGS Purpose Objective: To ready DNA/RNA for sequencing by making it compatible with NGS technology. Process End Repair: Blunting DNA fragment ends. A-Tailing: Adding an 'A' nucleotide to the 3' ends. Adapter Ligation: Attaching adapters crucial for sequencing. Size Selection: Choosing fragments of the correct length. Amplification: Enriching the library via PCR. Illumina Sequencing Methodology Sequencing by Synthesis: Incorporates fluorescently-labeled nucleotides. Signal Detection: Each nucleotide addition emits a unique fluorescence captured by the system. Precision and High-Throughput Technology: Sequencing by synthesis with reversible terminators. Read Lengths: Ranging from 75 to 300 bases for diverse applications. Throughput: Capable of generating up to billions of reads. Accuracy: Notably high, especially for short reads. Applications: Ideal for an extensive array of sequencing projects. Ion Torrent Sequencing Methodology Semiconductor Sequencing: Detects hydrogen ions released during DNA polymerization. Translation of Chemical to Digital: Ion detection is converted into sequence data. Real-Time Detection with Semiconductor Tech Technology: DNA polymerization-induced hydrogen ion detection. Read Lengths: Shorter, up to 400 bases. Throughput: Fits small to medium-scale projects. Accuracy: Slightly lower, challenges with homopolymer stretches. Applications: Targeted and microbial sequencing, among others. Oxford Nanopore Sequencing Methodology Nanopore Sequencing: Measures changes in electrical conductivity as DNA passes through a nanopore. Long-Read Capability: Enables reading of longer DNA sequences in real-time. Long Reads and Portability Technology: Ionic current changes as nucleic acids pass through nanopores. Read Lengths: Exceptionally long, enabling complex assembly tasks. Throughput: Scale varies by device, from on-the-go to lab-scale. Accuracy: Lower per read but improvable; useful for structural insights. Applications: Comprehensive for genome assembly and RNA analysis. Data Analysis Pipeline in NGS Primary Analysis: Turning Raw Data into Sequences Base Calling: Transforming signals (fluorescent or electrical) into nucleotide sequences. Quality Control: Ensuring sequence integrity by trimming and cleaning data. Data Analysis Pipeline in NGS Secondary Analysis: Understanding the Genetic Code Alignment: Positioning reads against a reference genome or constructing contigs. Variant Calling: Detecting genetic differences like SNPs, indels, and structural changes. Data Analysis Pipeline in NGS Tertiary Analysis: Beyond Sequencing Functional Annotation: Linking variants to genes and their biological roles. Data Interpretation: Associating genetic information with traits or diseases. Comparative Analysis: Exploring genetic diversity within and between populations. Matching NGS Modalities with Research Needs Key Considerations for Platform Selection Project Scale: Throughput needs based on genome size and sample quantity. Complexity: Requirement for longer reads to unravel structural intricacies. Resolution: High accuracy and depth for detecting SNPs and small variants. Budget: Costs can vary widely; consider per run and per base expense. Operational Factors: User-friendliness, processing time, and data analysis demands. Conclusive Thoughts on Platform Selection Tailoring Technology to Research Diversity of Platforms: Each NGS modality serves different scientific purposes. Strategic Decision-Making: Balancing scientific aims with practical constraints is vital. Alignment with Goals: Ensure the chosen NGS technology matches research intents. Application of NGS in Science and Medicine Transformative Impacts of NGS Technologies Whole-Genome Sequencing (WGS): Research: Unlocks comprehensive genomic understanding. Clinical Diagnostics: Powers genetic disorder identification. Public Health: Monitors pathogen dynamics. Application of NGS in Science and Medicine Targeted Resequencing: Exome Sequencing: Efficient focus on protein-coding regions. Application of NGS in Science and Medicine RNA-Seq: Gene Expression: Measures transcriptional changes. Alternative Splicing: Reveals transcript diversity. Non-coding RNA: Investigates regulatory RNA roles. Application of NGS in Science and Medicine Epigenetics: Bisulfite Sequencing: Assesses DNA methylation patterns. Metagenomics: Microbial Diversity: Profiles community composition. Functional Analysis: Infers microbiota functions. Single-Cell Sequencing: Breakthroughs: Illuminates cellular diversity and function. Personalized Medicine: Customized Treatment: NGS guides therapy selection. Pioneering Advances Across Biological Research Broadening Horizons with NGS Unparalleled Resolution: Every cell, gene, and molecule tells a story. Expanding Applications: From basic research to clinical practice. Evolving Technology: Continuous advancements redefine possibilities. NGS in Cancer Genomics: A Case Study Precision Medicine in Action Intervention: Patient Background: Enrollment in a trial for a novel, mutation-specific EGFR inhibitor. 54-year-old female with Outcome: advanced NSCLC. Positive response to treatment with Limited response to traditional significant tumour reduction. chemotherapy. NGS Application: Whole-exome sequencing reveals a rare EGFR exon 20 insertion mutation. Discussion 1. Navigating NGS Data Interpretation: NGS Impact on Personalized Care Challenges in clinical data analysis. 1. Precision Diagnosis with NGS: Ethical dimensions: privacy and Role of NGS in pinpointing specific incidental findings. mutations. Influence on tailoring therapies to 2. Envisioning the Future: genetic profiles. NGS's potential to revolutionize 2. Advantages of Targeted Therapy: cancer treatment. Comparison with traditional cancer Broader medical applications of NGS treatments. technologies. NGS's role in improving patient prognosis. NGS Data Challenge in Clinical Settings Overcoming the Information Avalanche The Data Deluge: Single genome sequencing results in >200GB of data. Storing the Genomic Trove: On-site vs. cloud solutions and associated trade-offs. Streamlining Data Flow: Importance of databases and LIMS for data integrity. Formulating data lifecycle policies. Computational Requirements for NGS Data Analysis Powering Through the Data Processing Demands: Necessity for HPC clusters or cloud computing for NGS data. Navigating Bioinformatics Pipelines: Steps: Alignment, Variant Calling, Annotation. Complexity in software selection and parameter optimization. Making Sense of Genomic Data From Data to Discovery Data Complexity: The challenge of distilling biological insights from extensive datasets. Variant Interpretation: Distinguishing pathogenic mutations. Leveraging databases for informed analysis. Visualization and Analytics: Tools for data representation. Machine learning's role in genomic pattern identification. Ethical Dimensions of NGS Data Balancing Innovation with Responsibility Incidental Findings: Strategies for managing and communicating unexpected genetic revelations. Data Privacy: Upholding the confidentiality of genetic data in research and clinical settings. Privacy, Consent, and Data Sharing in NGS Navigating the Privacy Landscape Confidentiality Concerns: The inherent sensitivity of genetic data. Principles of Data Sharing: Enhancing research while safeguarding privacy. Informed Consent: Clarifying the use and risks associated with data sharing. Regulatory Frameworks: Overview of HIPAA and GDPR guidelines. Summary and Future Prospects Innovations on the Horizon Data Synthesis: Integrating multi-omic data for Technological Advances: comprehensive analysis. Improving accuracy, longer Smart Analysis: reads, increased speed, and AI and machine learning's evolving reduced costs. role in genomics. Expanding the Scope: Evolving Ethics: Single-cell genomics and The necessity for updated ethical portable sequencing. frameworks.