Genetics 2: Use of Genetics in Clinical Work PDF
Document Details
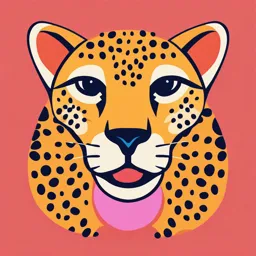
Uploaded by CheeryTinWhistle
University of Bristol
Emi Barker
Tags
Related
- Clinical Genetics in Medical Practice PDF
- ILM: Clinical Genetics Basics PDF (July 2024)
- Clinical Bacteriology (Lec) PDF
- Cystic Fibrosis Genetics: From Molecular Understanding to Clinical Application (2015) PDF
- Principles of Clinical Cytogenetics and Genome Analysis PDF
- An Introduction To Clinical Human Genetics PDF
Summary
This document covers the use of genetics in clinical work, defining genotype and phenotype, and providing examples of differential gene expression like coat color. It explores single nucleotide polymorphisms (SNPs), genome mapping, and genetic screening in animals. The document also describes different types of mutations and their consequences. Lastly, it offers guidance on interpreting results and predicting outcomes.
Full Transcript
Genetics 2: Use of Genetics in Clinical Work VETS10018/ASPL1/LEC Emi Barker BSc (hons) BVSc (hons) PhD PGCertTLHE DipECVIM-CA FRCVS RCVS Recognised and EBVS® European Veterinary Specialist in Small Animal Internal Medicine Clinical Lead in Infectious Diseases [email protected] Summary...
Genetics 2: Use of Genetics in Clinical Work VETS10018/ASPL1/LEC Emi Barker BSc (hons) BVSc (hons) PhD PGCertTLHE DipECVIM-CA FRCVS RCVS Recognised and EBVS® European Veterinary Specialist in Small Animal Internal Medicine Clinical Lead in Infectious Diseases [email protected] Summary Intended Learning Outcomes: Be able to define genotype and phenotype – and explain the Genotype vs. difference between the two Be able to use basic coat colour as an example of differential phenotype gene expression (dominance, recessive, epistaxis, Single nucleotide lyonization) Be able to explain what a single nucleotide polymorphisms is polymorphisms and how it can be used in genome mapping List the uses of genome mapping Point mutations / List the types of point mutations / variants, list the variants consequences of these mutations on translation and transcription and provide examples of each Genetic Be able to list the uses of genetic screening Be able to predict likelihood of affected / carrier in offspring, screening based on parental genotype, and parental genotype based on offspring phenotype Have a basic understanding of blood groups and why they are important Understand benefits and limitations of genotyping for feline blood type Genotype vs. phenotype Each individual has two copies (alleles) of each gene, one inherited from each parent (= genotype) – Exception: if the gene is on the X or Y-chromosome All somatic cells in the same animal carry identical genes – but different cells have different functions and develop inside different organs The expressed phenotype of cells / organisms influenced by: – Genotype – the alleles present – Inherited epigenetic factors (e.g. DNA methylation) – Non-inherited environmental factors (e.g. temperature, cytokines) Genetic mutations / variants Alterations to the genetic code can increase, modify or stop the production of a specific protein Consequences of alterations to the coding sequence of the genetic code on the consequential amino acid sequence can be easy to predict, and to a lesser extent the effect on the individual Consequences of alterations to the non-coding sequence are much more difficult (to impossible) to predict Basic mammalian genetics Dominance – Dominant allele (usually) produces a functional protein Only one copy required to affect the phenotype May be complete or partial (incomplete) – Recessive allele (usually) produces a reduced / faulty protein Both recessive copies required to affect the phenotype – Some alleles are ‘co-dominant’ Allele b may be recessive to allele B but dominant to third allele b’ Dominance does not indicate whether an allele is beneficial, detrimental, or neutral Example of application: Equivalent genes are found Basic coat colour in dogs in many other mammalian species Dominance / recessive allele interaction – Black/brown – controls type of eumelanin produced B is dominant and produces black eumelanin b is recessive and produces brown eumelanin – Extension – controls the proportion of eumelanin and pheomelanin E extends the amount of eumelanin into the hair e reduces eumelanin and increases pheomelanin (yellow/red) Epistasis – i.e. gene interaction – Absence of extension – results in yellow coat but the nose colour will reflect the type of eumelanin produced Labrador coat colour Yellow Brown Labrador Labrador (brown nose) bb, E- bb, ee Yellow Labrador Black Labrador (black nose) B-, E- B-, ee Single nucleotide polymorphisms (SNPs) At any single position within the genome sequence nucleotide differences may occur These differences can be passed down from one generation to the next and their presence used as (linkage) markers for the presence of nearby genes controlling traits of interest – Genome-wide association studies (GWAS) using SNPs can focus the search for target genes – Analysis of SNPs can be massively parallel, automated and high-throughput Genome mapping in animals Identify the gene(s) responsible for a genetic trait of economic or clinical importance – Model for human disease – Map genes in animal pedigrees – Eliminate affected individuals and carriers from the gene pool Marker-assisted selection – Use in conventional breeding programmes to increase frequency of desirable traits – Introgression from one population into another e.g. introducing fecundity genes from Chinese pigs into European breeds DNA sequencing using Sanger technique Based on properties of dideoxynucleotide (ddNTPs) – Modified nucleotides that contain a hydrogen group on the 3’ carbon instead of a hydroxyl group (OH) – When integrated into a DNA sequence they prevent the addition of further nucleotides thus stop the elongation of the DNA chain Reactions containing individual ddNTPs (i.e. ddGTP, ddATP, ddTTP and ddCTP) can be run separately on a gel or labeled with different fluorophores and processed in the same reaction A C G T DNA sequencing using pyrosequencing Combination of biochemistry, physics, optics and phenomenal computing power Illumina MiSeq sequencer Example of application: Sensory neuropathy in the Border Collie GWAS study Manhattan plot – Two populations compared – one with target trait, the other without – Each dot signifies a SNP – Genomic coordinates are on the X-axis, with the negative logarithm of the association P- value for each SNP on the Y- axis – Investigation can then be focused on candidate genes in this region Example of application: Sensory neuropathy in the Border Collie Region contained 27 genes One was FAM134B (associated with hereditary sensory neuropathy in humans) = candidate gene Sequencing of FAM134B exons (coding sequence) was unremarkable A 6.47MB inversion was identified with breakpoints in intron 3 of FAM134B and in an upstream intergenic region All affected dogs (no controls) were homozygous for this inversion Point mutations / variants Insertion, deletion, or substitution of base pairs (NB: not all are SNPs) Alteration of the exonic sequence can result in: – Silent – no change to the amino acid sequence – Missense – a different amino acid is encoded – Nonsense – a premature STOP codon is introduced – Frame shift – change in amino acid sequence from that point on Alteration of the intronic sequence can result in: – No changes – Altered splicing sites – change in the amino acid sequence – Altered gene expression (increased or decreased) Autosomal dominant Characteristics – Defect seen in every generation – Every affected offspring has at least one affected parent – Normal offspring from affected parents will produce normal offspring – Equal numbers of male and females will be affected – If the defect is rare, but not lethal: Most matings that produce an affected individual will be aa x Aa Segregation ratio will be 0.5 Examples – Feline polycystic kidney disease – nonsense point mutation in PKD1 – Scottish fold osteodystrophy (incomplete penetrance) – missense point mutation in TRPV4 Autosomal recessive Characteristics – Defect may skip a generation – All offspring of affected parents (where both affected) will be affected – “Normal” parents of an affected individual must be a carrier – Equal numbers of male and females will be affected – If the defect is rare, but not lethal: Most matings which produce an affected individual will be Aa x Aa Segregation ratio will be 0.25 Examples – Hyperuricosuria in Dalmatian dogs – missense point mutation in SLC2A9 – Avermectin sensitivity in Collie dogs – frame-shift mutation (4bp deletion) in ABCB1 X-linked dominant Characteristics – Every affected offspring has at least one affected parent – Affected males (i.e. XAY-) mated to normal females (i.e. XaXa) will transmit the defect to all their daughters and none of their sons – Unless the defect is very common: Affected females will be heterozygous (i.e. XAXa) and therefore when mated to a normal male the defect will be transmitted to half the offspring Example – X-linked dominant hereditary nephritis in Samoyed – nonsense point mutation in COL4A5 Sedwards63 CC BY-SA 4.0 X-linked recessive Characteristics – May skip a generation – Incidence in male >> female – When the defect is rare: Affected individuals will be males and will have inherited the gene from the dam Example – Haemophilia A (Factor VIII deficiency) in Havanese dogs – SINE (short interspersed nuclear element) insert in F8 – The causative mutation of haemophilia in most other breeds (including cocker spaniels) is unknown Why perform genetic screening? Identify carriers – e.g. recessive disorders – Gradually remove from the gene pool – Avoid mating two carriers – reduce risk of breeding affected individuals Identify affected – e.g. with late onset presentation – Remove affected cats from the gene pool / counsel owners – Avoid mating affected / carriers – reduce risk of breeding affected cats Select for desirable traits that are recessive / polygenetic – Direct mating to increase likelihood of obtaining desirable offspring How to perform genetic screening? Collect a sample containing DNA from patient: – Collected by vet or owner – Cheek swab (contamination risk if suckling) or blood sample (invasive) – Might need to record microchip ID at time of sampling (official record) Submit sample to laboratory: – Currently most tests are for individual mutations / variants Specific to species and may be specific to breed NB: may be more than one mutation / variant per phenotype Some (most) mutations / variants are unknown Interpret results Interpreting results Results may be reported as: – Normal or homozygous ‘wild type’ alleles – Carrier (heterozygous for recessive allele) – Affected (homozygous for recessive allele; heterozygous or homozygous for dominant allele) Forward planning – Affected individuals should be monitored for disease progression; prospective owners should be warned – Affected individuals should not be bred from (potentially difficult if heterozygous affected for dominant allele ⇒ reduced gene pool) – Carrier animals can be used cautiously in breeding programmes NB: Whilst it is possible to say Predicting offspring… which genotypes may or may not be present and give an idea of the likely ratios it is NOT possible to be Normal (AA) X Carrier (Aa) specific Predicted ratios – 50% predicted be ‘normal’ A a – 50% predicted to be carriers AA Aa A AA Aa A NB: Whilst it is possible to say Predicting offspring… which genotypes may or may not be present and give an idea of the likely ratios it is NOT possible to be Carrier (Aa) X Carrier (Aa) specific Predicted ratios – 25% predicted be ‘normal’ A a – 50% predicted to be carriers AA Aa – 25% predicted to be A ‘affected Aa aa a Genetic screening – unknown mutation… Determination of carrier status of normal animal (i.e. AA or Aa) – Backcross to a known homozygous recessive (‘aa’) If seven or more normal (A-) offspring are born >99% likelihood that the test animal is not a carrier (i.e. if Aa x aa all Aa = 0.57 = 0.008) – Backcross to a known heterozygous carrier (‘Aa’) If sixteen normal (A-) offspring are born 99% likelihood that the test animal is not a carrier (i.e. if Aa x Aa all AA or Aa = 0.7516 = 0.010) – If any affected (homozygous recessive, aa) offspring are born to either combination test animal is a carrier Blood groups A blood type is a classification of blood – Based on the presence and absence of antibodies and inherited antigenic substances on the surface of red blood cells (RBCs) – Antigens may be proteins, carbohydrates, glycoproteins, or glycolipids (which may also be present on the surface of other cell types) – Several of these red blood cell surface antigens can stem from one allele and collectively form a blood group system Mixing of blood with even only minor antigenic differences differences has the potential to result in significant reactions – Transfusion reactions – Neonatal isoerythrolysis Feline blood groups AB blood group system [NB: completely independent of human ABO system] – Cytidine monophospho-N-acetylneuraminic acid hydroxylase (CMAH) – ’B’ antigen (acetylneuraminic acid) ’A’ antigen (glycolylneuraminic acid) – Two known alleles – A and b (null; recessive) – Rarely type AB cats – both A and B antigens expressed – Alloantibodies All type B cats have strong anti-A antibodies (without prior exposure) ~⅓ type A cats have weak anti-B antibodies Mik red cell antigen – Cats are either positive or negative – Appears the be rare in the UK https://icatcare.org/advice/blood-groups-and-blood-incompatibility/ Feline blood groups prevalence Amongst domestic shorthairs: – 77-95+% of cats are type A – permanent red blood cell sequestration, loss in 3-5 days – DEA 4 => no effect in vitro Blood groups in other species Horse – Complex; 7 systems – A, C, D, K, P, Q and U – The two most potent antigens are Aa and Qa – Horses do not normally have alloantibodies from birth Cattle – Complex; 12 different systems and each system can have many alleles – Groups of alleles act as multiple antigenic determinants and are called phenogroups Neonatal isoerythrolysis Mechanism – Dam produces antibodies against the offspring’s RBC – Newborn absorbs these antibodies from the colostrum within the first 24-48 hrs following birth – Life-threatening haemolysis occurs Mainly a problem in the horse and cat – Horse – mating a group Aa negative dam to a group Aa positive sire producing a group Aa positive foal (usually requires prior exposure) – Cat – mating a type B queen to a type A stud cat producing type A kitten(s) (NB: this can happen at first mating) Avoiding neonatal isoerythrolysis Assess potential – blood type breeding animals if: – History of neonatal isoerythrolysis, – (In cats) if breed has a high incidence of type B (e.g. BSH; Devon Rex) Reduce risk – avoid combinations with potential to mismatch: – Mate Aa negative stallions with Aa negative mares – Mate type B queens to type B stud cats (but… this is a faff, makes type B cats ‘less desirable’, and limits gene pool) Assess risk – test offspring at birth: – Safe offspring treated as normal – At risk offspring hand-reared for 24-48 hours or fostered Online resources for genetic testing Langford Vets – Cat Genetics – https://www.langfordvets.co.uk/diagnostic-laboratories/cat-genetic- faqs/ Veterinary Genetics Laboratory – https://vgl.ucdavis.edu/ Laboklin https://www.laboklin.co.uk/laboklin/GeneticDiseases.jsp Summary Genotype vs. phenotype – Basic canine coat colour as an example Single nucleotide polymorphisms – GWAS Point mutations / variants Genetic screening – Predicting likelihood of affected / carrier in offspring – Blood group genotyping as an example Any Questions or Feedback? If you have any questions relating to this series of lectures – please post to the relevant padlet (chances are someone else is thinking the same thing!). Feedback is also gratefully received – things to improve, things you would like more of, things that did or didn’t work well – either anonymously on the padlet or feel free to email if you have specific queries [email protected] https://uob.padlet.org/emibarker/emi-barker-s-24-25-root-padlet-30nns46t9hq6p8n8