2.1 Mechanics of Breathing.pptx
Document Details
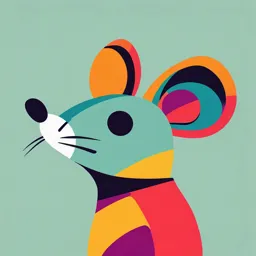
Uploaded by WorkableCreativity2568
TCU
Full Transcript
Mechanics of Breathing Lecture Outline 1.Generation of a Pressure Difference 2.Pressure-Volume Relationships in the Respiratory System 3.Interaction of Lung and Chest Wall 4.Airways Resistance 5.Work of Breathing 1 Mechanics of Breathing Objectives (Levitzky p 12) 1.Describe the generation of a pres...
Mechanics of Breathing Lecture Outline 1.Generation of a Pressure Difference 2.Pressure-Volume Relationships in the Respiratory System 3.Interaction of Lung and Chest Wall 4.Airways Resistance 5.Work of Breathing 1 Mechanics of Breathing Objectives (Levitzky p 12) 1.Describe the generation of a pressure gradient between atmosphere and alveoli 2.Describe the passive expansion and recoil of the alveoli 3.Define the mechanical interaction of the lung and chest wall and relate to the concept of negative intrapleural pressure 4.Describe the pressure-volume characteristics of the lung and chest wall and predict changes in the compliance of the lung and chest wall in different physiologic/pathologic conditions 5.State the roles of pulmonary surfactant and alveolar interdependence in the recoil and expansion of the lung 6.Define the functional residual capacity (FRC) and describe changes in FRC in different physiologic and pathologic conditions 7.Define airways resistance and list factors that contribute or alter the resistance to airflow 8.Describe the dynamic compression of airways during a forced expiration 9.Relate changes in the dynamic compliance of the lung to alterations in airways resistance 10.List the factors that contribute to the work of breathing 11.Predict alterations in the work of breathing in different physiologic and pathologic states 2 References Assigned reading from your text: Levitzky Chapter 2 3 I. Generation of a Pressure Difference 4 Boyle’s Law Boyle’s Law states pressure is inversely proportional to volume at a constant temperature – Pressure of a gas is the force exerted per unit area on the walls of its container – Volume of gas is the enclosed space of its container When volume increases, pressure decreases When volume decreases, pressure increases The product of pressure and volume remains constant The product of volume and pressure remains constant Mathematical expression: P1V1 = P2V2 To illustrate (assume isothermal conditions) - Pre-inspiration: V = 3L P = 4 mmHg = 12 Inspiration: V = 4L P = 3 mmHg = 12 Active Expiration: V = 2L P = 6 mmHg = 12 5 Inspiration is an Active Process Lungs passively expand Incapable of self-inflation Air will move from a high pressure area to a low pressure area (it follows a pressure gradient) No pressure difference = no movement Pressure difference must be sufficient to overcome the resistance to airflow offered by the conducting airways If ambient pressure is greater than airway pressure, air flow follows its gradient and enters the lung 2 Pressure Gradient and Air Movement Airway pressure equals ambient air pressure (barometric pressure) down to alveoli at end of expiration (end-expiration) – Alveolar pressure considered 0 cm H2O pressure at end-expiration – Atmospheric pressure (760 mmHg) is referred to as 0 cm H20 Negative pressure breathing – Normal physiologic breathing – Air moves into lungs as a result of airway pressure decreasing below atmospheric pressure In past- Iron lung – negative pressure mechanical ventilator Positive pressure breathing – Mechanical ventilation – Air moves into the lungs as a result of atmospheric pressure exceeding airway pressure 2 Passive Expansion of Alveoli A pressure difference between the atmosphere and alveoli must be established to move air in/out of alveoli During inspiration, alveoli expand passively in response to an increased transmural pressure difference Increased volume Decreased pressure During normal quiet expiration, the elastic recoil of the alveoli returns them to original volume Decreased volume Increased pressure Find FRC on the graph 3 Air Movement Into and Out of Lungs Air moves into lungs when alveolar pressure is lower than atmospheric pressure Increasing lung volume lowers airway pressure below atmospheric pressure Pressure gradient is created that allows airflow into the lungs from the external environment Air moves out of lungs when alveolar pressure is sufficiently higher than atmospheric pressure Decreasing lung volume increases the airway pressure above atmospheric Pressure gradient is created that allows airflow from the lungs to the external environment 2 Functional Residual Capacity Functional Residual Capacity (FRC) Is the volume of gas in the lungs at the end of a normal tidal expiration, when no respiratory muscles are actively contracting – Occurs at the end of passive expiration It is determined by the balance of two forces: – Inward recoil of the lungs and – Outward recoil of the chest wall 2 Intrapleural Pressure is Normally Negative Intrapleural pressure is the pressure within the pleural cavity Intrapleural pressure is negative (subatmospheric) at FRC (relaxation volume) Lungs have a tendency to collapse due to inward elastic recoil of distended alveoli Chest wall has a tendency to expand outward due to elastic recoil of flexible thorax Negative IPP due to ~20 ml pleural liquid adheres opposing forces Inward recoil of the lungs and Outward recoil of the chest wall IPP is not responsible for lung expansion but keeps lungs expanded -3 to -5 cm H2O caused by the mechanical interaction between lung and chest wall At end expiration- lung and chest at an equilibrium of rest At end inspiration of a normal tidal volume IPP becomes more negative = -8 cm H2O e.g. Contrast positive IPP during pneumothorax 3 Changes in IPP and the “Thoracic Pump” Increased respiratory activity of the “thoracic pump” promotes venous return and cardiac output During inspiration, contraction of the inspiratory muscles increases intrathoracic volume IPP becomes more negative and expands lungs Decreased external pressure dilates SVC & IVC and heart chambers Increased pressure gradient for venous return favors increased blood flow to right heart Produces a transient decrease in LV filling Additionally, the diaphragm descends causing an increased intra-abdominal pressure exerted on the IVC below the diaphragm forces blood flow toward the heart During expiration, an increasing IPP decreases venous return to the right atrium – IPP increases compresses blood vessels increases resistance to blood flow Decreases venous return to right heart Increases LV filling – Venous valves in lower regions of body (legs) prevent backward flow of blood 12 Clinical Application: Cardiopulmonary Resuscitation CPR and intrathoracic pressure- From Moore p. 380 Applying firm pressure to the thorax over the sternum moves sternum 4-5 cm posteriorly Increased intrathoracic pressure forces blood out of the heart & into the great arteries When external pressure is released, intrathoracic pressure falls,& the heart fills with blood 13 Expiratory Tension Pneumothorax Air enters during inspiration that cannot leave with expiration – Flap of damaged tissue acts as a one-way valve – During inspiration: flap is open and air enters pleural cavity – During expiration: flap is closed and air is prevented from leaving Result: IPP is increased decreases venous return decreases cardiac output – Compresses the mediastinum and the heart 14 Pressures Involved in Respiration Alveolar pressure – Alveolar pressure = intrapulmonary pressure = airway pressure – Alveolar pressure = intrapleural pressure + alveolar elastic recoil pressure – Pressure inside the airways Intrapleural pressure (IPP) = IPP – Pressure inside the pleural cavity; it is normally negative – Reflected throughout the entire lung – P surrounding alveoli and intrapulmonary airways – Can become positive during forced exhalation or positive pressure ventilation – Measured by balloon in the intrathoracic portion of esophagus Transpulmonary pressure is the transmural pressure – Transmural pressure difference = Inside pressure – outside pressure – Transpulmonary pressure = Alveolar pressure – intrapleural pressure – Distending pressure across the alveolar wall or whole lung 4 Pressure Changes During Inspiration due to the Interaction of the Lung and Chest Wall Note the pressures between end expiration and pressure changes during inspiration As inspiratory muscles contract: – The IPP becomes more negative and – The transmural (transpulmonary) pressure increases – Alveoli distend and decrease alveolar pressure 4 17 Muscles of Respiration Muscles of respiration include Diaphragm External intercostals Scalenes Abdominal muscles Internal Intercostal muscles 18 Muscles of Inspiration Diaphragm is the primary muscle of inspiration – Accounts for ½ tidal inhalation when upright and up to 2/3 when supine – Dome moves downward 1-2 cm into the abdominal cavity Abdominal wall is compliant – Diaphragm is not essential for breathing at rest – Diaphragmatic or abdominal breathing uses only the diaphragm: Diaphragmatic breathing can occur during spinal anesthesia During deep inspiration– Dome moves down as much as 10 cm into the abdominal cavity – The limit of abdominal wall compliance is reached Contraction of external intercostals is sufficient for ventilation at rest in the event of diaphragmatic paralysis – Loss of external intercostal muscle contraction does not have major impact on ventilation during exercise 19 Accessory Muscles of Inspiration “Tripod” Position- Post-exercise, COPD, epiglottitis – Leaning on a table or putting their hands on their knees fixes their scapulae and clavicles so these muscles can act on their rib attachments and expand the thorax. Sternocleidomastoid – Unilateral contraction flexes head laterally- use this to find landmark for PNBs – Bilateral contraction pulls head forward – Assists pump-handle action of deep respiration With cervical vertebrae fixed, elevates the manubrium and medial ends of clavicle Pectoralis major, pectoralis minor, and serratus anterior – – – – Not true thoracic wall muscles- but extend from thoracic cage (axial skeleton) Help elevate the ribs to expand the thoracic cavity When respiration is deep and forceful (exercise) Pulmonary rehab builds accessory muscles to assist breathing (lung disease) 20 Accessory Muscles of Inspiration- Scalenes Anterior, middle, and posterior scalenes contract to lift the upper thorax – Action: Anterior flexes head Middle flexes neck laterally & elevates first rib Posterior flexes neck laterally & elevates second rib – Light to moderate contraction of scalenes fixes/immobilizes upper thorax allows the external intercostals to raise the lower ribs to a greater degree 21 Expiration Decreased lung volume increases pressure in the airways air flows out of lungs – Expiration is approximately twice as long as inspiration – Normal I:E ratio is 1:2-1:4 Two lung factors decrease lung volume: – Inward recoil of elastic tissue of lung to resting state – Surface tension recoil in alveoli: more important than elastic tissue recoil Passive expiration requires no muscular activity Active expiration requires muscular activity – Utilized during: exercise, singing, coughing, Valsalva or pathophysiologic states (eg COPD) – Muscular contractions decreased volume of thoracic cavity decrease lung volume – Functional aspects of active expiration: Accelerates exhalation Allows for greater exhalation volumes of air from ERV Increases CO 22 Muscles of Expiration- Abdominal Muscles Abdominal Muscles are major muscles of expiration Attached to lower rib cage and upper portion of pelvic girdle including pubic symphysis – Immobilizes the lower thorax and pulls down on the rib cage during contraction Contraction of the abdominal muscles decreases all three dimensions of thorax: – Transverse – Anterior-posterior – Vertical Increases intra-abdominal pressure which pushes up on the diaphragm, decreasing the vertical dimension of thorax 23 Summary of Events During a Eupneic Breath Summary of events Muscular contraction / relaxation changes thoracic volume Change in IPP Change in alveolar pressure Air flow Change in lung volume 24 Summary of Volume, Pressure, and Airflow During an Idealized Breath Volume (measured by spirometer) Thoracic volume increases as the chest wall expands Thoracic volume decreases IPP (estimated by intraesophageal balloon) Solid line- actual, dashed line- predicted changes in IPP IPP becomes more negative + work of breathing IPP becomes less negative Airflow (measured by pneumotachograph) Air flows in until alveolar equals atmospheric pressure Air flows out until atmospheric P equals alveolar Alveolar pressure (calculated) Initially alveolar pressure = atmospheric pressure Changes in alveolar pressure establishes a gradient 25 II. Pressure-Volume Relationships in the Respiratory System 26 Compliance C= V/ P A measure of the lung’s distensibility – The ease with which lungs can be inflated – Compliance is an elastic property High compliance is due to a loss of elastance Low compliance is due to high elastance Compliance of the lung is reflected in both – Elastic tissue recoil Lungs tend to collapse Chest wall tends to expand – Surface tension recoil Lungs tend to collapse Squeeze the bag to check compliance27 Non-Linear Aspects of Compliance Compliance is volume dependent Alveoli are more compliant at low volumes (and have less elastic recoil) – Lungs distend easily Alveoli are less compliant ( and have more elastic recoil) at high volumes – – At high volumes- large increases in pressure yield small increases in volume At high lung volumes, lungs are already highly stretched so much that an increased IPP only yields a small increase in V Lungs are not compliant at very low and very high lung volumes At approximately FRC ~ 3000 ml – the compliance curve becomes linear 28 Two Types of Compliance: Static and Dynamic Static compliance is measured – While no airflow is occurring – Exhalation in stages with measurements Dynamic compliance is measured with airflow- during inspiration and expiration – The dynamic compliance curve: – Has both inspiration and expiration limbs – The difference between the curves is hysteresis 29 Dynamic Compliance in Obstructive Disease Dynamic compliance Dynamic compliance changes with increased breathing frequencies in obstructive disease 30 Variations in Compliance The ability to inflate lungs is affected by variations in compliance: Increased compliance indicates increased distensibility – – Decreased compliance indicates decreased distensibility – – Lungs are more easily stretched on inspiration High compliance has a steep slope- Obstructive disease Lungs are more difficult to stretch on inspiration Low compliance has a flatter slope- Restrictive disease C= V/ P Units are C= L/cm H2O or ml/cm H2O Normal value C =.2 L/ cmH2O 31 Histology of Abnormal Compliance Conditions that produce abnormal compliance: Fibrosis- Soft tissues become less distensible Decreases compliance due to excess fibrous tissue Results in decreased V with same IPP Emphysema- Soft tissues expands more readily Increases compliance due to destruction of elastic septa results in increased V with same IPP The patient with emphysema has: A large lung volume due to air trapping A non-compliant chest wall Difficulty expiring due to loss of elasticity 32 Pressures Related to Compliance Static compliance is measured in the absence of gas flow; based on plateau pressure: Cstat = Vt/(Pplat – PEEP) Dynamic compliance is measure in the presence of gas flow; based on peak pressure: Cdyn = Vt/(Ppeak – PEEP) 33 34 Clinical Evaluation of Lung Compliance- Specific Compliance A measure of distensibility of lung as it relates to lung volume – Compliance/ FRC – Lung compliance/unit of lung volume – L/cm H2O/ L lung volume – Measurement begins at FRC since the compliance relationship is linear at this point Significance of specific compliance: – Used denote compliance with respect to original lung volume – Compliance decreases with decreased lung volume; specific compliance does not – Standardizes for overall lung size/volume – Analogous to comparing CO and CI 35 Example of Specific Compliance = C / FRC Example of compliance for two lungs – – – – IPP = 5 cm H2O; reflects the change of IPP from end-expiration to end-inspiration Equal volumes of air go to both lungs (1/2 L to each lung) Assume an FRC = 2L Compliance for two lungs = V/ IPP i.e., 1L/5 cm H2O = 0.2L/cm H2O Specific compliance for two lungs – C/FRC = 0.2L/cm H2O/ 2.0l = 0.1L/cm H2O/L Example of compliance for person with only one lung inhales.5 L Air – Change in IPP = 5 cm H2O – – Compliance = V/ IPP i.e., 0.5L/5 cm H2O = 0.1L/cm H2O Compliance for one lung appears half as compliant as two without factoring FRC Specific compliance for one lung – C/FRC = 0.1L/cm H2O/1.0l = 0.1L/cm H2O/L 36 Elastance Elastance – Tendency to oppose stretch – Elastic recoil of alveolar walls increases at higher lung volumes – Increased elastance compresses alveolar gas – Raises alveolar above atmospheric pressure (exhalation) 37 Elastic Recoil of Lungs - Inward Recoil due to elastic tissue – Elasticity: property of matter that causes it to return to it original position or configuration after having been displaced (stretched) – Elasticity is the inverse of compliance Hooke’s law applies to elastance F = k * x – (F=Force, k=constant, x=distance of deformation) – The stretch placed on an elastic body is proportional to the force applied to it Elastic tissue of lungs follows Hooke’s law using the following: Force is replaced with IPP Distance of deformation is replaced with lung volume 38 Surface Tension Accounts for 2/3 of total elastic recoil forces in the normal lung Air-liquid interface within the lungs that attempts to collapse lungs due to surface tension forces – Surface tension forces occur at any gas-liquid interface – Generated by cohesive forces between water molecules and hydrogen bonding when unopposed at the surface of a liquid – Surface tension is what causes water to bead Causes water molecules to from the smallest surface area possible In alveoli, the inner alveolar surface lined by a fluid in contact with air – Surface tension of the fluid is attempting to contract and force air out of lungs – Net effect results in the overall surface tension recoil force of the alveoli or lungs 39 Law of La Place States the pressure within a spherical bubble is directly proportional to the surface tension and inversely proportional to the radius of the bubble at the liquid-air interface P = 4T/r P=pressure T = tension and r= radius La Place worked with bubbles have two air-liquid interfaces P = 2T/r Alveoli have only one air-liquid Interface and are assumed spherical Rearranging La Place’s law shows the relationship between the pressure inside a distensible sphere and the tension of its walls T = P x r/2 Surface tension has a resolved Inward direction of force-Whereas the air pressure inside the sphere is directed outward 40 Surface Tension and the Law of Laplace If the lung were interconnected alveoli with a constant surface tension at the alveolar-air interface, there would be a tendency for smaller alveoli to collapse Yet- Two factors for alveolar stability: – Pulmonary surfactant – Structural interdependence of alveoli 41 The Inherent Instability of Alveoli Smaller sphere is expected to collapse Example: two nearby alveoli are connected to each other have the same common airway (alveolar duct), and the same external pressure If each of the alveoli has a different radius and both have the same surface tension According to La Place’s law the alveolus with the smaller radius (r=1 unit) should generate a higher internal pressure forcing air into the larger alveolus (with r= 5 units) Surface tension is 50 dynes/ unit P1 = 2(50)/1 = 100; P=100 to keep smaller alveolus open P2 = 2(50)/5 = 20; P=20 to keep the larger alveolus 42 Surfactant Surface active agents (surfactants) are substances that decrease surface tension – Surfactants have little attraction for other surfactant molecules or water molecules – A surfactant placed in a volume of water in a beaker uniformly disperses itself throughout the water, and displaces water molecules - reducing their concentration – Reduced concentration of water molecules at the surface reduces the surface tension Pulmonary surfactant: surface active agent at the fluid surface of the inner lining of the alveoli – Major role is stabilizing alveoli by equalizing pressure inside the smaller alveoli – Derived from type II alveolar epithelial cells (type II pneumocytes) Phospholipids (80%), Cholesterol (10%) & Surfactant proteins (10%) – Polar molecule due to phospholipid component Hydrophilic and hydrophobic regions at opposite ends of the molecule – Hydrophilic end faces inward toward the alveolar lining – Hydrophobic end points outward toward the fluid-air interface – Has an immune function 43 Surfactant Decreases Surface Tension of the Smaller Alveolus As the radius of the smaller alveolus decreases, surfactant molecules increase in concentration at the air-liquid interface The smaller alveolus has less surface tension – This decreases alveolar surface tension proportional to the size of the alveolus – The end result is that the force of air pressure needed to inflate both sizes of alveoli remains the same so from the standpoint of surface tension all alveoli are equal 44 Surfactant and Alveolar Volume Changes Following inspiration Surface tension will increase due to the higher water concentration at the surface in conjunction with the decreased concentration of surfactant The greater the inspiration the greater the recoil due to increased surface tension Following expiration Surface tension decreases when alveoli deflate and surfactant concentration is increased 45 Pulmonary Surfactant Increases Alveolar Compliance Pulmonary surfactant increases alveolar compliance and helps prevent atelectasis by reducing surface tension in smaller alveoli Hysteresis is the difference between inspiration/expiration curves Due to Surfactant has less effect on decreasing surface tension during inspiration than expiration – Due to movement of surfactant molecules 46 Importance of Pulmonary Surfactant Reduces surface tension recoil forces and the muscular efforts needed to ventilate – Surfactant stabilizes the alveoli and prevents them from collapsing Aids in keeping the alveoli dry – A deficiency would increase surface tension and pull fluid out of the pulmonary capillaries and into the alveoli 47 Surfactant Deficiency Conditions associated with a deficiency of surfactant – – – – Atelectasis Failure of normal lung expansion in premature neonates Pulmonary edema - caused by fluid transfer from pulmonary capillaries alveoli Respiratory Distress Syndrome Possible causes of surfactant deficiency – – – – – – – Immature lung Surfactant is not normally secreted until between the 6 th and 7th month gestation Premature neonates can be given synthetic aerosol surfactant May give the mom steroids which may increase the maturation of Type II cells Hypoxia- lack of O2 reduces cellular metabolic activities Interrupted blood supply – loss of pulmonary blood- embolism- can reduce O 2 Locally with the Insufficient production of surfactant 48 Alveolar Interdependence Mechanical interdependence stabilizes alveoli and opposes collapse Collapsing alveoli would increase the stress on the walls of adjacent alveoli and tend to hold it open Elastic septa – capillaries 49 Atelectasis Caused by respiratory changes during anesthesia FRC decreases (~1.5 L) Compliance decreases Resistance increases Atelectasis develops in 90% anesthetized lungs Includes bases- localized or global A cause of hypoxemia The usual suspect in postoperative fever Obesity contributes to development Prevention of atelectasis during anesthesia Positive end-expiratory pressure Recruitment maneuvers Minimizing gas resorption Maintenance of muscle tone 50 III. Interaction of Lung and Chest Wall 51 Interaction of Lung and Chest Wall Inward elastic recoil of lung normally opposes the outward recoil of the chest wall Chest wall recoil becomes inward at 70% TLC – – Below 70%, chest wall elastic recoil is outward Above 70%, chest wall elastic recoil directed inward If integrity is disturbed (pneumothorax)- Interdependence ceases and alveoli tend to collapse Air moves in until IPP equalizes with atmospheric pressure and abolishes the transpulmonary pr gradient – Chest wall expands – Lung recoils 52 Static Pressure-Volume Curves of the Lung, Chest Wall, and Both Functional residual capacity At FRC, the volume of gas in lungs at end normal tidal expiration- Your patient’s “reserve” FRC= (0 cm H2O) – – – the volume where outward recoil of chest wall is equal to the inward recoil of the lungs At high lung volumes- (>70%) mouth pressure positive because both lung and chest wall elastic recoil are inward At low lung volumes- (2000 = turbulent flow Transitional Distribution of Airway Resistance 35-50% in upper airways Resistance greater when breathing through nose than mouth Although a single small airway has the highest resistance- numerous small airways arranged in parallel have low resistance laminar flow Negative pressure breathing pulls upper airway inward- can obstruct airflow; Reflex contraction of the pharyngeal dilators keeps airway open Recall areas of obstruction in each portion of the pharynx 56 Active Control of the Airways Parasympathetic control predominates in normal lung 57 Relationship Between Lung Volumes and Airway Resistance Airways resistance decreases with increasing lung volume Small airways are compressible and traction on small airways increases Airway resistance is greater in emphysema due to secretions/ inflammation Small airways: Compressible At higher lung volumes- traction on small airways increases 58 Dynamic Compression of Small Airways Dynamic compression causes small airway collapse after the equal pressure point During forced expiration, with an open glottis- when IPP is positive- small airways are compressed (dynamic compression) Equal pressure point - a functional result that produces obstruction and air trapping The point during a forced expiration where pressure inside the airway is equal to the pressure outside the airway Frictional resistance causes a fall in the driving pressure along the length of the conducting airway At the point that driving pressure equals surrounding peribronchial pressure- the net transmural pressure is zero= the equal pressure point 59 Alveolar Elastic Recoil and Forced Expiration When dynamic compression occurs- Alveolar elastic recoil pressure becomes the effective driving pressure for airflow from the lung Driving pressure is the difference between alveolar pressure (sum of pleural pressure and lung elastic recoil pressure) and atmospheric pressure (assumed 0) Alveolar elastic recoil pressure opposes dynamic compression of the airways In emphysema- great difficulty achieving high airflow rates due to less alveolar elastic recoil to generate a driving pressure and less traction to oppose dynamic compression More forceful exhalation produces greater IPP and more collapse and flow becomes effort independent 60 Lung Volumes and Capacities Ganong FIGURE 34–8 Lung volumes and capacity measurements. Lung volumes recorded by a spirometer. Lung capacities are determined from volume recordings. See text for definitions. (Reproduced with permission from Fishman AP: Fishman’s Pulmonary Diseases and Disorders, 4th ed. McGraw Hill Medical, 2008). 61 Lung Volumes and Capacities VC- Vital capacity (IRV + TV + ERV) – Volume of air expelled from fully inflated lungs TLC- Total lung capacity (IRV + TV+ ERV+ RV) – The volume of air in the lungs after a maximal effort RV- Residual volume – Volume of gas left in the lungs after a maximal forced expiration FVC- Forced vital capacity – Forced exhalation from TLC – Largest amount of air that can be expired after maximal inspiration FEV1- Forced expiratory volume in the 1 st second – Volume of air that can be expired in 1 second after maximal inhalation – Fraction of the VC that can be expired in 1st second 62 Airways Resistance and FVC Measurements of forced vital capacity are measures of expiratory airway resistance FEV1/FVC – represents larger airways FEF 25%-75% – represents medium-sized airways In obstructive disease, increased airway resistance takes time to overcome 63 Isometric Pressure-Flow Curves at Three Lung Volumes Airflow becomes independent of effort due to greater dynamic compression with more positive IPP Middle curve at 50% VC – – Demonstrates dynamic compression and the equal pressure point hypothesis Increasing expiratory effort increases up to a pointbeyond that a more positive IPP does not increase airflow. It becomes effort independent. 64 Flow-volume Curves of Varying Intensities Two interesting points: Effort dependence and independence At high lung volumes, airflow rate is effort-dependent At low lung volumes, expiratory efforts all merge into same effort-independent curve – IPPs high enough to produce low lung volumes produce dynamic compression – At low lung volumes- there is less alveolar elastic recoil pressure Peak expiratory flow is the maximal flow rate the patient can attain 65 Flow-Volume Curves Maximal expiratory flow-volume curves representing obstructive and restrictive diseases Peak expiratory flow is decreased with obstructive and restrictive diseases 66 Inspiratory and Expiratory Flow-Volume Curves in Obstruction A- Fixed intra-or extra thoracic obstruction Caused by foreign bodies, tumors, scarring – eg previous intubation or tracheostomy B- Variable extra thoracic obstruction Caused by tumors, fat deposits, weakened pharyngeal muscles (OSA), paralyzed cords C- Variable intrathoracic obstruction of the trachea are most commonly caused by tumors 67 V. Work of Breathing 68 Movement of Lungs is Passive and Determined by Two Types Resistance 2. Non-elastic tissue resistance Occurs mostly in disease states Occurs only during motion Pulmonary tissue resistance caused by friction of moving tissues 69 Factors to Overcome During the Work of Breathing In restrictive disease The work required to overcome elastic resistance increases as tidal volume increases In obstructive disease The work required to overcome nonelastic (airway) resistance increases as respiratory rate and expiratory flow increase 70 1. The forced vital capacity is: A. the amount of air that normally moves into (or out of) the lungs with each respiration B. the amount of air that enters the lungs but does not participate in gas exchange C. the amount of air expired after maximal inspiratory effort D. the largest amount of gas that can be moved into and out of the lungs in 1 minute 2. Airway resistance E. does not affect the work of breathing F. is increased in paraplegic patients G. is increased following bronchial smooth muscle contraction H. makes up 80% of the work of breathing I. J. K. L. 3. Surfactant lining the alveoli helps prevent alveolar collapse is produced in alveolar type I cells and secreted into the alveolus is increased in the lungs of heavy smokers is a glycolipid complex 4. Downstream from the equal pressure point, pressure outside the airway is: M. Less than the driving pressure inside the airway N. Greater than the driving pressure inside the airway O. Equal to the driving pressure inside the airway P. Unpredictable compared to the driving pressure inside the airway 5. Q. R. S. T. Work of breathing increases in restrictive disease as: FRC increases Respiratory rate increases Expiratory flow increases Tidal volume increases 71