Lecture 15: Metabolism Of Nitrogenous Compounds PDF
Document Details
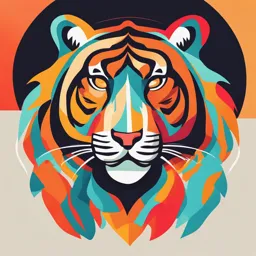
Uploaded by ProfoundBrown
Tags
Summary
This document is a lecture on biochemistry, specifically the metabolism of nitrogenous compounds. It discusses the utilization of inorganic nitrogen and ammonia. The content focuses on reaction pathways and the role of various enzymes in nitrogen assimilation.
Full Transcript
1 Lecture 15 Metabolism of Nitrogenous Compounds Utilization of Inorganic Nitrogen: 2 The Nitrogen Cycle Pathways of nitrogen metabolism (purple) in the general pattern of intermediary metabolism: Utiliz...
1 Lecture 15 Metabolism of Nitrogenous Compounds Utilization of Inorganic Nitrogen: 2 The Nitrogen Cycle Pathways of nitrogen metabolism (purple) in the general pattern of intermediary metabolism: Utilization of Inorganic Nitrogen: 3 The Nitrogen Cycle Utilization of Ammonia: 4 Biogenesis of Organic Nitrogen Reactions in assimilation of ammonia and major fates of the fixed nitrogen: 1.Glutamate dehydrogenase 2.Glutamine synthetase 3.Asparagine synthetase 4.Carbamoyl phosphate synthetase 5.Glutamate synthase. In animals, glutamine, rather than NH3, is the chief nitrogen source for pyrimidines. Utilization of Ammonia: 5 Biogenesis of Organic Nitrogen Several ubiquitous enzymes use ammonia as a substrate for synthesis of glutamate, glutamine, asparagine, or carbamoyl phosphate. Utilization of Ammonia: 6 Biogenesis of Organic Nitrogen Glutamate dehydrogenase catalyzes the reductive amination of a-ketoglutarate: Utilization of Ammonia: 7 Biogenesis of Organic Nitrogen A related enzyme that occurs only in microorganisms, plants, and lower eukaryotes, glutamate synthase, catalyzes a reaction comparable to that catalyzed by glutamate dehydrogenase but functions primarily in glutamate biosynthesis: Utilization of Ammonia: 8 Biogenesis of Organic Nitrogen Whether formed by action of glutamate dehydrogenase or glutamate synthase, or by transamination, glutamate can accept a second ammonia moiety to form glutamine in the reaction catalyzed by glutamine synthetase. Mn2+ is required. Utilization of Ammonia: 9 Biogenesis of Organic Nitrogen The glutamine synthetase reaction occurs via an acyl phosphate intermediate. ATP phosphorylates the d-carboxylate of glutamate to give a carboxylic- phosphoric acid anhydride (g-glutamyl phosphate), which undergoes nucleophilic attack by the nitrogen of ammonia to give the amide product, glutamine. Utilization of Ammonia: 10 Biogenesis of Organic Nitrogen Prokaryotic glutamine synthetase is controlled by: o Cumulative feedback inhibition § Involves the action of eight specific feedback inhibitors. § Metabolic end products of glutamine (trp, his, glucosamine- 6-phosphate, carbamoyl phosphate, CTP, and AMP) § Indicators of the general status of amino acid metabolism (ala, gly) o Covalent modification § Adenylylation of a specific tyrosine residue Utilization of Ammonia: 11 Biogenesis of Organic Nitrogen Regulation of the activity of bacterial glutamine synthetase: The complex of AT (adenylyltransferase) and a regulatory protein catalyzes both the adenylation and deadenylation of glutamine synthetase (GS), depending on whether is deuridylated (left) or uridylated (right). Uridylation of is catalyzed by uridyltransferase (UT), and deuridylation is catalyzed by uridyl-removing enzyme (UR), both activities part of a bifunctional enzyme. Utilization of Ammonia: 12 Biogenesis of Organic Nitrogen If NH4+ levels are high, the glutamate dehydrogenase/glutamine synthetase pathway operates: Utilization of Ammonia: 13 Biogenesis of Organic Nitrogen When NH4+ levels are low, the glutamate synthase/glutamine synthetase pathway predominates: Utilization of Ammonia: 14 Biogenesis of Organic Nitrogen Asparagine Synthetase: Catalyzes a reaction comparable to that of glutamine synthetase. Although widespread, asparagine synthetase accounts for much less ammonia assimilation. This enzyme uses ammonia or glutamine in catalyzing the conversion of aspartate to asparagine. Utilization of Ammonia: 15 Biogenesis of Organic Nitrogen The final route for assimilating ammonia first forms carbamoyl phosphate. The enzyme responsible is carbamoyl phosphate synthetase. Either ammonia or glutamine can serve as the nitrogen donor: The Nitrogen Economy: 16 Aspects of Amino Acid Synthesis and Degradation The Nitrogen Economy: 17 Aspects of Amino Acid Synthesis and Degradation Transamination is the reversible transfer of an amino group from an a-amino acid to an a-keto acid, with pyridoxal phosphate as a coenzyme. The Nitrogen Economy: 18 Aspects of Amino Acid Synthesis and Degradation Aminotransferases utilize a coenzyme, pyridoxal phosphate, that is derived from vitamin B6. The functional part of the cofactor is an aldehyde functional group, CHO, attached to a pyridine ring. The catalytic cycle begins with condensation of this aldehyde with the a-amino group of an amino acid, to give a Schiff base, or aldimine, intermediate. The Nitrogen Economy: 19 Aspects of Amino Acid Synthesis and Degradation Transamination can be used for amino acid synthesis and also for degradation of amino acids that accumulate in excess of need. In degradation the transaminase works with glutamate dehydrogenase, as exemplified by the degradation of alanine: The Nitrogen Economy: 20 Aspects of Amino Acid Synthesis and Degradation Most aminotransferases use glutamate/a-ketoglutarate as one of the two a-amino/a-keto acid pairs involved. Two such enzymes are important in the clinical diagnosis of human disease serum glutamate-oxaloacetate transaminase (SGOT) and serum glutamate-pyruvate transaminase (SGPT): These enzymes, abundant in heart and in liver, are released from cells as part of the cell injury that occurs in myocardial infarction, infectious hepatitis, or other damage to either organ. Assays of these enzyme activities in blood serum can be used both in diagnosis and in monitoring the progress of a patient. 21 The Nitrogen Economy: Aspects of Amino Acid Synthesis and Degradation 22 Protein Turnover Proteins are subject to continuous biosynthesis and degradation, a process called protein turnover. For an intracellular protein whose total concentration does not change with time, the steady state level is maintained by synthesis of the protein at a rate just sufficient to replenish protein lost by degradation. Many of the amino acids released during protein turnover are reutilized in the synthesis of new proteins. 23 Protein Turnover 24 Protein Turnover Chemical Signals for Turnover: The turnover rates for different proteins vary by as much as 1000-fold, whereas differences in protein stability, as measured by denaturation in vitro, may be much less. Four structural features are currently thought to be interrelated determinants of turnover rate: o Ubiquitination, o Metal-catalyzed oxidation of particular residues o PEST sequences o particular N-terminal residues Protein Turnover 25 Ubiquitin is a small (76-residue) protein expressed in all eukaryotic cells—it derives its name from its widespread (ubiquitous) distribution. Ubiquitin is covalently conjugated to specific cellular proteins in an ATP-dependent reaction, which condenses the terminal carboxyl group of ubiquitin with lysine amino groups on target proteins. In the first reaction, catalyzed by the ubiquitin-activating enzyme E1, a thioester is formed between the C-terminal Gly residue of ubiquitin and an internal Cys residue of E1 in a two-step process. Formation of this high-energy covalent linkage is driven by ATP hydrolysis, via a ubiquitin-adenylate intermediate. Protein Turnover 26 Structure of the proteasome: The proteasome contains two major assemblies, a 28-subunit core particle (aka the 20S particle) and a 19-subunit regulatory particle (aka the 19S particle), which forms the base and lid assemblies. The proteolytic active sites are located within the large internal space (~100 x 60Å) of the 20S core particle. The lid and base assemblies of the 19S regulatory particle control substrate entry into the 20S core particle. Proteins tagged with the protein ubiquitin pass through the tube in an ATP-dependent fashion. Protein Turnover 27 Enzymatic pathway for attachment of ubiquitin to target proteins: E1, E2, and E3 are proteins involved in the ubiquitin transfer to lysine residues on target proteins. Amino Acid Degradation and Metabolism of 28 Nitrogenous End Products Amino acid degradation usually begins with conversion to the corresponding a-keto acid by transamination or oxidative deamination. Amino Acid Degradation and Metabolism of 29 Nitrogenous End Products Amino Acid Degradation and Metabolism of 30 Nitrogenous End Products Animals have evolved pathways, adapted to their lifestyles, for excretion of ammonia, uric acid, or urea as the major nitrogenous end product. Amino Acid Degradation and Metabolism of 31 Nitrogenous End Products Urea is synthesized almost exclusively in the liver and then transported to the kidneys for excretion. The synthetic pathway, which is cyclic, was discovered by Hans Krebs and Kurt Henseleit in 1932. Amino Acid Degradation and Metabolism of 32 Nitrogenous End Products Urea is synthesized by an energy-requiring cyclic pathway that begins and ends with ornithine. The net reaction for one turn of the urea cycle is as follows: Amino Acid Degradation and Metabolism of 33 Nitrogenous End Products The Krebs– Henseleit urea cycle: Amino Acid Degradation and Metabolism of 34 Nitrogenous End Products Amino Acid Degradation and Metabolism of 35 Nitrogenous End Products Transport of ammonia to the liver for urea synthesis. The carrier is glutamine in most tissues, except for muscles, where this role is played by alanine. Amino Acid Degradation and Metabolism of 36 Nitrogenous End Products The glucose–alanine cycle removes toxic ammonia from muscle. Glutamine synthetase and glutaminase do the same for most other tissues. Coenzymes Involved in Nitrogen Metabolism 37 Vitamin B6 is also called pyridoxine. The active coenzyme has been oxidized to an aldehyde and the hydroxymethyl group at position 5 is phosphorylated. Pyridoxal phosphate (PLP) is the predominant coenzyme form Pyridoxamine phosphate (PMP) is an intermediate form in transamination reactions. 38 Coenzymes Involved in Nitrogen Metabolism All pyridoxal phosphate reactions involve initial Schiff base formation, followed by bond labilization caused by electron withdrawal to the coenzyme’s pyridine ring. 39 Coenzymes Involved in Nitrogen Metabolism Folate coenzymes contain multiple glutamate residues, which help them be retained within cells and bind more tightly to enzymes. 40 Coenzymes Involved in Nitrogen Metabolism Dihydrofolate reductase is the target for a number of useful anticancer, antibacterial, and antiparasitic drugs. Coenzymes Involved in Nitrogen Metabolism 41 Human dihydrofolate reductase complexed with ligands: This figure shows the X-ray crystal structure of the human enzyme with bound NADPH (yellow) and methotrexate (red). The additional amino group on methotrexate allows formation of an additional hydrogen bond to the enzyme, which increases its binding affinity to the folate binding site. These two ligands bind such that the pyridine ring of NADPH is in close proximity to the pteridine ring of the folate ligand, as required for the hydride transfer catalyzed by this enzyme. Notice that the folate binds in a bent, rather than linear, form. Coenzymes Involved in Nitrogen Metabolism 42 Before World War II, one of the few effective antibacterial drugs available was sulfanilamide, one of the class of sulfonamide drugs (“sulfa drugs”). D. D. Woods, noted a structural similarity between sulfanilamide and p-aminobenzoate (PABA), which was known to be essential for bacterial growth. Woods proposed that sulfanilamide acts by blocking the normal utilization of PABA, and he coined the term antimetabolite. PABA is not required for growth of animal cells, so the drug is not toxic to human cells. The enzyme incorporating PABA into folic acid is inhibited by sulfonamides. Because animal cells do not carry out the synthetic pathway but instead take up fully formed folate from the diet, they are not harmed by the drug Coenzymes Involved in Nitrogen Metabolism 43 Metabolic reactions involving synthesis, interconversion, and utilization of one- carbon adducts of tetrahydrofolate: 44 Coenzymes Involved in Nitrogen Metabolism Glycine can yield an additional molecule of 5,10- methylenetetrahydrofolate through action of the glycine cleavage system, a multienzyme complex located in mitochondria: 45 Coenzymes Involved in Nitrogen Metabolism Structure of vitamin B12: The molecule shown here is the cyanide- containing form originally isolated (cyanocobalamin). In cells, a water molecule or hydroxyl group takes the place of CN, forming the precursor to the coenzyme forms of B12. The corrin ring is shown in red. 5,6-Dimethylbenzimidazole (DMB), which is linked to the cobalt, is shown in blue. 46 Coenzymes Involved in Nitrogen Metabolism The role of B12 as a methyl transferase in Met biosynthesis: B12 coenzymes have either a methyl group or a 5’- deoxyadenosyl moiety linked to cobalt, making them the first known organometallics in metabolism. 47 Coenzymes Involved in Nitrogen Metabolism Coenzymes derived from vitamin B12: The corrin ring, identical in all known forms of B12, is indicated here schematically. The Co bears a positive charge (n = 1, 2, or 3), while each molecule is uncharged overall. 48 Coenzymes Involved in Nitrogen Metabolism Pernicious anemia is caused by deficiency of a glycoprotein needed for intestinal absorption of vitamin B12, leading to intracellular deficiencies of B12 coenzymes. B12 deficiency causes 5-methyltetrahydrofolate to accumulate, with concomitant depletion of other folate coenzymes. Folic acid deficiency increases the risk of cardiovascular disease and birth defects in humans. Coenzymes Involved in Nitrogen Metabolism 49 A relationship between folate and B12 metabolism: This scheme is based on the apparent folate deficiency seen in early stages of B12 deficiency as a result of decreased flux through the methionine synthase reaction. 50 Pathways of Amino Acid Degradation Glucogenic amino acids are amino acids whose carbon skeletons are degraded to one of these five intermediates: o Pyruvate o a-Ketoglutarate o Succinyl-CoA o Fumarate o Oxaloacetate Ketogenic amino acids are amino acids whose carbon skeletons are degraded to: o Acetyl-CoA o Acetoacetate Some amino acids are both glucogenic and ketogenic. Pathways of Amino Acid Degradation 51 52 Pathways of Amino Acid Degradation Hydrogen sulfide (H2S), derived from cysteine, is a powerful gaseous signaling molecule involved in the regulation of vascular blood flow and blood pressure. Pathways of Amino Acid Degradation 53 Asparaginase catalyzes hydrolytic cleavage of the asparagine amide to aspartate and ammonium. Aspartate is then transaminated directly to oxaloacetate by aspartate transaminase. Pathways of Amino Acid Degradation 54 Histidine also undergoes decarboxylation to generate histamine, a substance with multiple biological actions. When secreted in the stomach, histamine promotes the secretion of HCl and pepsin. It is a potent vasodilator, released locally in sites of trauma, inflammation, or allergic reaction. Antihistamines, such as the two shown in the margin, are in use to treat allergies and other inflammations. Typically, these drugs prevent the binding of histamine to its receptors. Pathways of Amino Acid Degradation 55 Branched-chain amino acid oxidation, fatty acid b-oxidation, and the citric acid cycle share a common chemical strategy: A deficiency of branched-chain a-keto acid dehydrogenase complex, which metabolizes valine, leucine, and isoleucine in humans, leads a severe mental developmental defect called maple syrup urine disease. 56 Pathways of Amino Acid Degradation Although lysine is degraded by several pathways, the major route in mammals, the saccharopine pathway, does indeed follow this predicted strategy. Saccharopine is the intermediate in the two-step process that removes the e-amino group from lysine: Pathways of Amino Acid Degradation 57 These two reactions are catalyzed by a bifunctional enzyme named a-aminoadipic semialdehyde synthase. The mammalian enzyme is a single protein composed of separate domains for the two catalytic activities. Although this process uses a-ketoglutarate as the amino acceptor, and produces glutamate, it is more similar to the argininosuccinate synthetase–argininosuccinase reactions of the urea cycle than to a transamination: Pathways of Amino Acid Degradation 58 Metabolic fates of tryptophan: The major catabolic pathway degrades most of the tryptophan molecule to acetoacetate and alanine. The synthetic pathway to the nicotinamide nucleotides. Pathways of Amino Acid Degradation 59 A hereditary deficiency of phenylalanine hydroxylase is responsible for phenylketonuria (PKU) In PKU, phenylalanine accumulates to very high levels (hyperphenylalaninemia) because of the block in conversion to tyrosine, and much of this phenylalanine is metabolized via pathways that are normally little used—particularly transamination to phenylpyruvate (a phenylketone), and also subsequent conversion of phenylpyruvate to phenyllactate. These compounds are excreted in urine in enormous quantities (1 to 2 grams per day). 60 Pathways of Amino Acid Degradation Tyrosine is hydroxylated to p-hydroxyphenylpyruvate catalyzed by p-hydroxyphenylpyruvate dioxygenase, an unusual iron- containing enzyme, which catalyzes a ring hydroxylation, decarboxylation, and side chain migration, using ascorbate as a cofactor. Procollagen prolyl hydroxylase catalyzes the same chemistry. This reaction involves a mechanism called the NIH shift, after scientists at the NIH, who described a ring hydroxylation that proceeds via formation of an epoxide intermediate and migration of the alkyl side chain. 61 Amino Acids as Biosynthetic Precursors There are indications that protein methylation protects proteins, in at least two ways: 1. By blocking sites of ubiquitination, methylation evidently helps protect proteins from turnover. 2. Spontaneous damage of protein molecules during aging causes deamidation, isomerization, and racemization of their asparagine and aspartate residues. A methylation reaction can initiate the repair of these damaged residues. Amino Acids as Biosynthetic Precursors 62 Methyl group metabolism and homocystinuria: Serine hydroxymethyltransferase catalyzes the entry of one- carbon units into the tetrahydrofolate (THF) pool. A deficiency of methylenetetrahydrofolate reductase (MTHFR) or methionine synthase blocks the conversion of homocysteine to methionine. Amino Acids as Biosynthetic Precursors 63 Whereas the one-carbon and methyl cycles are found in virtually all cells, liver and kidney possess an additional route for methionine synthesis that involves a different kind of transmethylation. Homocysteine can be remethylated using betaine as the methyl donor in a reaction catalyzed by the cytosolic betaine- homocysteine methyltransferase. Betaine, also known as trimethylglycine, is formed from the mitochondrial oxidation of choline. 64 Amino Acids as Biosynthetic Precursors Homocysteinemia can result from: Genetic defects in: o Cystathionine b-synthase o Methionine synthase o 5,10-Methylenetetrahydrofolate reductase Dietary deficiency of: o Folic acid o Vitamin B6 o Vitamin B12 Amino Acids as Biosynthetic Precursors 65 Polyamines are required for cell proliferation because of their roles in stabilizing duplex DNA structures: 66 Amino Acids as Biosynthetic Precursors In addition to serving as a precursor for ornithine and the polyamines, glutamate is one of several amino acids serving as precursors to compounds that function in transmission of nerve impulses. Decarboxylation of glutamate yields – aminobutyric acid, or GABA. In addition, glutamate itself is a neurotransmitter. 67 Amino Acids as Biosynthetic Precursors Biosynthesis of nitric oxide and creatine phosphate from arginine: In NO synthesis, the Nw-hydroxy-L-arginine intermediate remains tightly bound to NO synthase (NOS). 68 Amino Acids as Biosynthetic Precursors Biosynthesis of thyroid hormones as residues in the protein thyroglobulin: The iodinated forms of tyrosine— triiodothyronine (T3) and thyroxine (T4) — are released from these proteins by proteolytic degradation. One result of iodine deficiency is goiter, a condition in which the thyroid gland grows abnormally large as it attempts to scavenge all available iodine. Amino Acids as Biosynthetic Precursors 69 Phenylalanine and tyrosine serve as precursors to an enormous number of plant substances, ranging from the polymeric lignin to tannins, pigments, and many of the flavor components of spices. In fact, the role of these amino acids as precursors to such substances in cinnamon oil, wintergreen oil, bitter almond, nutmeg, cayenne pepper, vanilla bean, clove, and ginger is related to their designation as aromatic amino acids. These are derived from coniferyl alcohol, which is also the central intermediate in lignin synthesis. L-Tyrosine is the starting point for the synthesis of opiates such as codeine and morphine in the poppy plant. 70 Amino Acids as Biosynthetic Precursors Phenylalanine serves as the precursor to a large number of plant pigments and related polyphenolic compounds called flavonoids. These include many flower colorants, which serve in part as ultraviolet protectants, and also the respiratory inhibitor rotenone. Amino Acids as Biosynthetic Precursors 71 The same biosynthetic scheme also leads to a class of flavonoids called anthocyanins, which are common flower pigments. Substituents on the rings determine specific color, as shown. An offshoot from this pathway leads to synthesis of cocaine. 72 Amino Acids as Biosynthetic Precursors Tryptophan is utilized for synthesis of a plant growth hormone. As shown here, the transamination product of tryptophan is decarboxylated to yield indole-3-acetic acid, or auxin. 73 Porphyrin and Heme Metabolism Porphyrin biosynthesis involves: 1. Formation of a pyrrole ring. 2. Condensation of four pyrrole moieties, giving a cyclic tetrapyrrole. 3. Side chain modifications and ring oxidations. Porphyrias involve abnormal accumulations of heme precursors, either from overproduction of the unnatural type I porphyrins or from abnormally high flux through d-ALA synthetase. Porphyrin and Heme Metabolism 74 Catabolism of heme: Most of the heme comes from breakdown of aged erythrocytes, but some comes from cytochromes and other heme proteins. Heme protein degradation in animals releases amino acids and iron, which are reused, and bilirubin, which must be solubilized for excretion. Amino Acids and Their Metabolites as 75 Neurotransmitters and Biological Regulators Glutamate, tyrosine, glycine, and tryptophan serve as neurotransmitters or precursors to neurotransmitters. The pathway to serotonin begins with hydroxylation of tryptophan by a tetrahydrobiopterin-dependent aromatic amino acid hydroxylase, similar to phenylalanine hydroxylase. This reaction is followed by a PLP-dependent decarboxylation to yield serotonin. Amino Acids and Their Metabolites as 76 Neurotransmitters and Biological Regulators Tryptophan hydroxylase and tyrosine hydroxylase are both tetrahydrobiopterin-dependent monooxygenases and are mechanistically and structurally related to phenylalanine hydroxylase. The mechanism of all three members of the aromatic amino acid hydroxylase family involves an NIH shift, with migration of a hydride accompanying the hydroxylation. Amino Acids and Their Metabolites as 77 Neurotransmitters and Biological Regulators Biosynthesis of the catecholamines—dopamine, norepinephrine, and epinephrine: Tyrosine hydroxylase catalyzes the rate-limiting step of catecholamine synthesis, and it is feedback inhibited by the end products of the pathway— dopamine, norepinephrine, and epinephrine. Amino Acid Biosynthesis 78