Acetabular Revision Surgery in Major Bone Defects PDF
Document Details
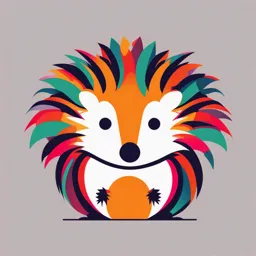
Uploaded by ConvincingHeliotrope112
2019
Eduardo García-Rey, Eduardo García-Cimbrelo
Tags
Summary
This book focuses on acetabular revision surgery in patients with major bone defects, providing a comprehensive overview of the topic. It covers the basic science of osteolysis, bone defect classifications, surgical techniques, and bone graft biology. Expert opinions and current research are integrated. This book is intended for orthopedic surgeons and related specialists.
Full Transcript
Acetabular Revision Surgery in Major Bone Defects Eduardo García-Rey Eduardo García-Cimbrelo Editors 123 Acetabular Revision Surgery in Major Bone Defects Eduardo García-Rey Eduardo García-Cimbrelo Editors Acetabular Revision Surgery in Major Bone Defects Editors Eduardo Gar...
Acetabular Revision Surgery in Major Bone Defects Eduardo García-Rey Eduardo García-Cimbrelo Editors 123 Acetabular Revision Surgery in Major Bone Defects Eduardo García-Rey Eduardo García-Cimbrelo Editors Acetabular Revision Surgery in Major Bone Defects Editors Eduardo García-Rey Eduardo García-Cimbrelo Orthopaedic Surgery Department Orthopaedic Surgery Department Hospital Universitario La Paz-IdiPaz Hospital Universitario La Paz-IdiPaz Madrid Madrid Spain Spain ISBN 978-3-319-98595-4 ISBN 978-3-319-98596-1 (eBook) https://doi.org/10.1007/978-3-319-98596-1 Library of Congress Control Number: 2018957994 © Springer Nature Switzerland AG 2019 This work is subject to copyright. All rights are reserved by the Publisher, whether the whole or part of the material is concerned, specifically the rights of translation, reprinting, reuse of illustrations, recitation, broadcasting, reproduction on microfilms or in any other physical way, and transmission or information storage and retrieval, electronic adaptation, computer software, or by similar or dissimilar methodology now known or hereafter developed. The use of general descriptive names, registered names, trademarks, service marks, etc. in this publication does not imply, even in the absence of a specific statement, that such names are exempt from the relevant protective laws and regulations and therefore free for general use. The publisher, the authors, and the editors are safe to assume that the advice and information in this book are believed to be true and accurate at the date of publication. Neither the publisher nor the authors or the editors give a warranty, express or implied, with respect to the material contained herein or for any errors or omissions that may have been made. The publisher remains neutral with regard to jurisdictional claims in published maps and institutional affiliations. This Springer imprint is published by the registered company Springer Nature Switzerland AG The registered company address is: Gewerbestrasse 11, 6330 Cham, Switzerland Preface Total hip arthroplasty (THA) has been recognised as one of the most important surgical advances during the last five decades. End-hip arthritis is probably the most disabling condition to affect daily life activities not only in elderly but also in young patients. The older population is increasing in most countries worldwide, and they are more active than ever during the last decades so cartilage aging plus more physi- cal and social demands in the more developed societies are strongly increasing THA indications. Thus, in young patients diagnoses other than primary arthritis, such as avascular necrosis, post-traumatic conditions, rheumatologic diseases and congeni- tal hip diseases or other developmental sequelae, influence THA outcome together with the possibility of loosening and wear at long term. All these issues contribute to a greater number of THA revision procedures. Nowadays, both cemented and cementless bone fixation can provide excellent long-term survival when using most contemporary implants. Most current femoral components have a survivorship higher than 95% more than 10 and 15 years after surgery. In fact, the THA revision procedure that is increasing is acetabular revision, and, as is reflected in most National Registries and clinical studies, the most fre- quent indication for THA revision surgery is related to the acetabular side. Indications for acetabular revision surgery at short term include dislocation, infec- tion or, more rarely, fractures. To date, recent problems related to newer, not-very well studied, implants have also increased the number of revision procedures due to newer complications. At long term, the most frequent reasons for hip revision sur- gery are wear and loosening with the appearance of osteolysis. During last years, the appearance of late dislocation in older patients has become of interest. Nevertheless, in an indication for acetabular revision surgery, the most challenging issue is the existence of bone defect. Proper hip reconstruction during surgery to allow the closest distance to the hip rotation centre and the longest duration of the implant is the main purpose of the treatment. First, a hip surgeon must be familiar with the basic science that can affect bone biology and changes associated to implants. A better understanding of all these changes related to bearing surfaces and bone interfaces facilitates clinical manage- ment when facing a patient with THA. Biological processes secondary to wear v vi Preface p articles and different reactions to all kinds of polyethylenes, metallic particles, ceramics and cement contribute to silent osteolysis until there is significant bone destruction or implant loosening. The hip surgeon must also be familiar with advances in research that may improve clinical management. Second, it is necessary to classify the bone defects in every patient. Different imaging techniques can now- adays improve preoperative diagnosis, while newer surgical tools and tricks can diminish bone loss during explantation of the failed acetabular component. Appropriate surgical planning is critical before starting an acetabular revision procedure. Recommendations in young patients presenting severe polyethylene wear with osteolysis and fixed implants are different than in other patients with loosened cups. The surgical team needs to be familiar with revision implants, tech- niques and bone graft use. Understanding the biology of the latter, particularly of allografts, improves the clinical and radiological outcome. Despite the surgical choice, the surgeon must keep in mind that bone defect determines surgical tech- nique. From conventional cementless cups to cages, bone impaction grafting or reinforcement rings, adequate hip rotation centre reconstruction in stable construc- tion will affect the clinical and radiological outcome of these patients. Independent industry-unrelated high-quality studies are the most reliable way to review all the different clinical choices. Finally, all issues associated to different complications, such as dislocation or infection, must be addressed for proper management. Acetabular revision surgery in the presence of bone defect continues to be of concern for the patient and the surgeon. In this book all the most critical topics are covered beginning with the basic science, trying to clarify some newer research findings, continuing with established reconstruction techniques with or without the use of bone graft are reviewed. Continuing high-quality clinical studies to evaluate this complex problem and improve our understanding of the concepts will allow us to reliably improve outcomes for our patients. Madrid, Spain Eduardo García-Rey Madrid, Spain Eduardo García-Cimbrelo Contents 1 Osteolysis After Total Hip Arthroplasty: Basic Science 1 G. Vallés and N. Vilaboa 2 Bone Defects in Acetabular Revision Surgery. Imaging and Classifications 33 Eduardo García-Rey 3 Prosthetic Component Fixation and Bone Defect Determine Acetabular Revision Surgery 49 Eduardo García-Cimbrelo and Eduardo García-Rey 4 Biology of Bone Grafting 79 Eduardo García-Rey and Enrique Gómez-Barrena 5 Acetabular Revision with Impaction Bone Grafting 93 Berend Willem Schreurs and Wim Rijnen 6 Biological Repair of Acetabular Bone Defects and Cup Migration After Impaction Bone Grafting in Total Hip Arthroplasty 103 Eduardo García-Rey and Eduardo García-Cimbrelo 7 Revision Surgery After Fractures of Ceramic Components 115 Luigi Zagra and Enrico Gallazzi 8 Antiprotrusio Cages for Acetabular Revision 125 Antonio Coscujuela, Jose Luis Agullo, and Daniel Rodriguez-Perez 9 Revision Arthroplasty of the Acetabulum Using Structural Allograft and a Cage: State-of-the-Art 137 E. Gibon, L. Kerboull, and M. Hamadouche 10 Total Hip Replacement Revision Using a Dual Mobility Cup Cemented into a Metallic Ring 159 Pascal Bizot vii viii Contents 11 Trabecular Metal in Acetabular Revision Surgery for Severe Bone Defects and Pelvic Discontinuity 173 José Sueiro-Fernandez 12 Acetabular Bone Defect in Infected Total Hip Arthroplasty 199 Jose Cordero-Ampuero and Eduardo García-Rey Index 217 Chapter 1 Osteolysis After Total Hip Arthroplasty: Basic Science G. Vallés and N. Vilaboa Total Hip Replacement: Clinical Need and Demand Total hip arthroplasty (THA) represents the most successful and revolutionary intervention achieved in orthopedic surgery in the last century [1–3]. This surgery is performed to restore the injured or degenerated joint function when conservative treatment options have failed and pain, stiffness and other limitations drastically reduce the patient’s quality of life. The clinical settings in which hip arthroplasty is indicated involve acute and chronic underlying joint-related diseases, mostly degen- erative osteoarthritis and rheumatoid arthritis but also other arthropathies including avascular necrosis, developmental and congenital disorders, neoplasias, fractures and post-traumatic degenerative arthritis. In 2010, the number of individuals bearing hip implants in USA was estimated in more than 2.5 millions. In Europe, countries with high incidence are Germany, Switzerland and Belgium with ratios, of 296, 287 and 240 THA procedures per 100,000 inhabitants, respectively, while in US and UK the frequencies are 184 and 194. Early hip failure (within 5 years of implantation) is mainly associated to instabil- ity, aseptic loosening (AL), infections, wear and fracture and has a decreasing trend due to improvements in surgery techniques and advances in the biomaterials field. However, an alarming prevalence has been detected in some cohorts, especially in patients with metal-on metal (MoM) bearings [6, 7]. In the long-term, the survival rate (endpoint at revision) of prosthesis after 10–15 years of implantation has been estimated about a 90–95% [8–10] although percentages of only 58–62% or even less have been also reported after longer service periods [10–12]. G. Vallés · N. Vilaboa (*) Hospital Universitario La Paz-IdiPaz, Madrid, Spain Centro de Investigación Biomédica en Red de Bioingeniería, Biomateriales y Nanomedicina, CIBER-BBN, Madrid, Spain e-mail: [email protected] © Springer Nature Switzerland AG 2019 1 E. García-Rey, E. García-Cimbrelo (eds.), Acetabular Revision Surgery in Major Bone Defects, https://doi.org/10.1007/978-3-319-98596-1_1 2 G. Vallés and N. Vilaboa Demographic changes and lifestyle habits, with a younger and more active pop- ulation suffering from disabling joint diseases, have led to a significant raise in the number of THA performed [4, 5, 13]. While THA was initially intended for elderly patients with limited activity, current candidates include young and middle-age patients as well as physically active elderly individuals [3, 13]. As a consequence, the incidence of total hip replacements (THR) increased by a 25% during the period 2000–2009 and is expected to increase annually at a rate of approximately a 5% per year in the coming years, to such an extent that in the period 2005–2030 THA procedures will increase a 174% [4, 14]. Approximately half of the joint replacements currently performed in USA are made in patients younger than 65 years. In Europe, 42% of men and 31% of women who underwent THA in England and Wales were in this age group. By 2030, 52% of THR might be performed in these patients [16, 17]. Given the limited expected time of service of prosthesis in patients younger than 50 years, the revision rates will dramatically increase in coming years [17, 18]. Implant Failure: Aseptic Loosening Implant failure is still a major complication of joint arthroplasty with severe conse- quences for the patient that impact the health-care system [8, 16, 19–21]. Failure, origined by mechanical and/or biological factors, can arise from multifactorial causes such as implant design, surgical technique, method of fixation or infection but the main factor limiting the longevity of THAs is AL secondary to peripros- thetic osteolysis [3, 13, 15, 17]. Specifically, implant loosening due to aseptic oste- olysis accounts for over 70% of total hip revisions while infections, recurrent dislocations, periprosthetic fractures and surgical errors contribute to about a 20% of the reported failures [21–23]. Instability and infection are complications fre- quently diagnosed in the early postoperative period, while osteolysis and AL usu- ally appear in the medium to long-term. Revision surgery is a procedure technically more challenging than primary arthroplasty and often associated with poorer prognosis and higher risk of failure [24, 25]. The late development and diag- nosis of AL, usually asymptomatic in early stages, and its association to severe periprosthetic bone defects are factors that compromise the revision surgery proce- dure [18, 26]. Patients for revision surgery are typically in their 70s and 80s, and age-associated issues including morbidity and mortality risks undermine the inter- vention. The exponential growth in the number of primary total joint replacement procedures is associated to a concomitant increase in the number of revision opera- tions. In this regard it has been estimated that the revision burden (ratio of revision/ primary THA) will be about 17% in 2030 [27, 28]. Statistical data have shown that 10 years after of the primary hip arthroplasty, 24% of cases develop osteolysis and about a 15% of recipients require further surgical intervention due to AL [17, 29]. In young patients, under 30 years, the percentage of cases which requires revision 1 Osteolysis After Total Hip Arthroplasty: Basic Science 3 surgery can reach rates up to 33% while in older patients range from 7% to 15%. Regarding components, the acetabular cup is revised more frequently than the femoral stem [30, 31]. Currently, 40,000 hip revision procedures due to loosening are performed annually in USA and it is expected to increase about a 137% for 2030 [14, 32]. AL is characterized by areas of osteolysis at the bone-implant interface, identi- fied radiographically as radiolucent zones, which can result in displacement of the prosthetic component [3, 33]. Progression of peri-prosthetic bone loss as assessed by radiology is very slow. Osteolytic lesions often appear many years after the pri- mary surgery and can be associated to mechanically stable implants. Clinical symptoms associated to bone tissue destruction may not be clearly apparent or even remain silenced. Several factors determine the longevity of hip implants. Apart from the impor- tance of a correct surgical technique (orientation and alignment of prosthetic com- ponents, prosthesis stability, anchorage and osteointegration of the implant …) there are significant differences regarding prosthesis- (e.g. type of bearing material, prosthesis design, shape of prosthesis, surface technology, type of fixation …) and patient- related factors (e.g. age, co-morbidities, level of activity and differences in mechanical loading,…) wich influence the host response to the implant and there- fore its success or failure [17, 35]. In this regard, Engh et al. found that implant wear and patient-specific propensity equally contribute to the degree of osteolysis and might account for the extent of the area affected by periprosthetic bone loss. Research efforts have attempted to identify clinical risk factors and individual sus- ceptibility to periprosthetic osteolysis in order to predict the outcome and prevent, or at least attenuate, the ensuing complications of THA. Over the last years, the influence of parameters such as gender, body mass index, and age [21, 37, 38], as well as the contribution of genetic factors to the development and progression of AL [15, 37, 38] have been considered. Regarding age and gender, young male patients show high risk of developing osteolysis. Considering other factors, genetic variations affecting molecules involved in inflammation and bone turnover may play a role in the predisposition to AL of patients with THA. Particularly, polimor- phisms in genes encoding proteins such as tumor necrosis factor-α (TNF-α), inter- leukin-6 (IL-6), transforming growth factor-β (TGF-β), interleukin-1 receptor antagonist (IL-1 Ra), matrix metalloproteinase-1 (MMP-1), osteoprotegerin (OPG) or receptor activator for nuclear factor kappa-B ligand (RANKL), have been associ- ated with susceptibility to osteolysis and/or short prosthesis longevity [15, 20, 39– 41]. These proteins are powerful mediators in the biological response to particulate wear debris and the periprosthetic bone loss around THR. Other risk factor associated to predisposition to osteolysis is the preoperative diagnosis, which might influence the host response to the biomaterial. While some authors have observed higher rates of loosening in patients who underwent THA due to inflammatory arthritis, post-traumatic arthritis, developmental dysplasia or osteonecrosis as compared to primary osteoarthritis, others have not found signifi- cant differences between these groups [15, 42]. 4 G. Vallés and N. Vilaboa The Enemy: Wear Debris Particles Osteolysis is the long-term consequence of the biological response to wear debris and products derived from corrosion of implants [3, 33, 43]. Particulate wear debris, mainly generated by articulating motion at the bearing surfaces but also by non- articular surfaces, are the primary causes of periprosthetic bone loss and implant loosening [44, 45]. Locally, continous exposure to prosthetic debris combined to repetitive mechanical stresses triggers an inflammatory chronic response which is highly influenced by the intrinsic properties of wear debris particles. The type of prosthesis determines the characteristics of the resultant wear debris particles and therefore the magnitude of the host response. Articulating bearings in THA are hard-on-hard material such as MoM or ceramic-on-ceramic (CoC) and hard-on-soft material (metal-on-polymer) couples, being the most satisfactory the combination of a cobalt chrome femoral head articulating on an ultra high molecular weight polyethylene (UHMWPE) acetabular component [33, 46]. Wear occurs through five major mechanisms: adhesion, abrasion, third body, fatigue, and corrosion. Third body wear damage is considered a relevant source of particles in which metal, poly- mer, cement or even cortical bone debris are entrapped between the UHMWPE acetabular component and the hard bearing surface contributing to accelerated dete- rioration of the implant [3, 47]. Research in materials manufacturing and tribology has focused on identifying alternative bearing surfaces that reduce the production of wear particles debris [3, 46]. These new materials might give rise to wear particles with unknown characteristics. In general, the standing paradigm of AL states that implant-derived-particles stimulate periprosthetic cells to release factors which affect cell functions through autocrine and/or paracrine mechanisms [13, 18, 33, 48]. Potentially, all cell types at the periprosthetic tissue are targets of wear debris but macrophages are critically involved [17, 29, 33, 45]. Macrophages activated by particles produce an array of inflammatory, chemotactic and bone-resorbing factors such as chemokines, growth factors, cytokines, degradative enzymes and reactive oxygen radicals, among oth- ers, triggering and perpetuating the periprosthetic osteolytic cascade [3, 24, 43, 48]. As result of the inflammatory response, a granulomatous pseudomembrane gener- ates at the bone-implant interface, which further compromises the interface stability and osseointegration of the device. Altered-load bearing effects contribute to abnor- mal wear processes and production of particles in the implant bed, leading in advanced stages of periprosthetic osteolysis to further anomalous mechanical load- ing. Although osteolysis due to wear debris initiates as a localized phenome- non, joint fluid can transport wear debris, cells and molecules to adjacent bone sites extending the affected bone-implant interface. Morever, high fluid production and altered loading increase periprosthetic pressure in the peri-implant region [34, 49]. Moreover, the extent of the inflammatory reaction to wear particles is not confined to joint and adjacent tissues since wear debris, mainly metal particles, have been detected in remote organs including the spleen, liver, kidney and lung [3, 45]. Whether systemic dissemination of wear particles may cause side effects, e.g. toxic- ity and carcinogenicity, is a matter of debate. 1 Osteolysis After Total Hip Arthroplasty: Basic Science 5 a b Fig. 1.1 Periprosthetic membrane retrieved from a patient undergoing revision surgery due to hip aseptic loosening. (a) Optical microscopy imaging. Hematoxylin-eosin staining showing charac- teristic features of the foreign body reaction induced by wear debris particles, with infiltration of inflammatory cells. High amounts of metal particles are found, indicated by white arrows. (b) Transmitted polarized light and confocal microscopy imaging. Nuclei of cells in the tissue were counterstained with DAPI (blue). UHMWPE particles were detected by polarized light as bright areas (indicated by a red arrow) and metal particles were detected by transmitted light (black, white arrows) and by reflection (green false colour labelled). Scale bar: 100 μm Histological examination of periprosthetic tissues retrieved during revision sur- gery has revealed significant amounts of prosthesis-derived particles (Fig. 1.1) and a multi-cellular composition characterised by the presence, among other cells, of macrophages, multinucleated foreign body giant cells containing engulfed particles, lymphocytes, fibroblasts and osteoclasts in association to elevated levels of pro- inflammatory cytokines, such as TNF-α, interleukin-1β (IL-1β), prostaglandin-E2 (PGE2) and RANKL [33, 50]. The phagocytosable range size of particles able to induce an in vitro inflamma- tory response has been established in less than 10 μm. In general, nanometer- sized particles with diameters lower than 150 nm can be internalized through endocytosis or pinocytosis, while particles sized between 150 nm and 10 μm utilize a phagocytosis-mediated process and particles higher than 20 μm induce multinu- cleated giant cell formation [33, 51]. The greater the particle load of phagocytosable particles, the higher is the local inflammatory response. Regarding shape, elongated particles are more reactive than round particles. Originally coined as “cement disease”, as osteolysis due to AL was thought to be a response to particles of polymethylmethacrylate (PMMA), later it was observed that osteolysis also occurs with cementless implants and potentially all kind of par- ticles (metals, PE, ceramics, and bone cement) can elicit a biological response in joint tissues [3, 33, 43]. Particle chemistry plays an important role [3, 13, 33]. In general, metal particles are more pro-inflammatory and/or toxic than polymers or ceramics [3, 52–54]. An overview of the most relevant and recent findings about the cellular events and molecular pathways modulated by the different types of wear debris is presented in the following sections. 6 G. Vallés and N. Vilaboa Polyethylene Particles Analyses of periprosthetic tissues retrieved during revision surgery have shown that UHMWPE wear debris originated from the acetabular liner are the most frequent types of debris, standing for 70–90% of the debris load independently if implants are cemented or not [3, 17]. Probably due to their abundance, UHMWPE particles are considered as key players in the stimulation of periprosthetic bone loss and implant loosening. Traditionally, the most used bearing couple was UHMWPE articulating against a metallic ball. With hundreds of thousands of UHMWPE particles generated in a single step in the periprosthetic space, about 500 billion of particles produced per year, and a total amount of trillions of particles during the lifetime of a prosthesis, osteolysis was associated to a threshold of 2.16 × 109 and polyethylene (PE) particles per gram of interfacial tissue and a wear rate greater than 0.1 mm/year of the UHMWPE acetabular liner [3, 17, 23, 55]. Histological examination of periprosthetic tissues have revealed that UHMWPE debris appears as small particles or large shards, and exhibit birefringence under polarized light. Once isolated from membranes, UHMWPE particles display an irregular surface with a predominant globular shape, although other irregular shapes are also observed especially in those of larger size, being 90% smaller than 1 μm [3, 17]. The different sizes and shapes observed have been related to the specific wear mode as well as to the implantation period [3, 45]. Small particles can be detected within macrophages while large particles are usually included within foreign body multinucleated giant cells. Bacterial lipopolysaccharide (LPS), possibly derived from subclinical infections or systemic sources, may bind to particles and contribute further to the inflamma- tory reaction [56, 57]. Some evidence suggests that macrophages might not be able to induce an inflammatory response if adsorbed endotoxins are not present on UHMWPE particles. Proteins present in the physiological fluids, including type I collagen, aggrecan proteoglycans, immunoglobulin, fibronectin and albumin, can also be adsorbed on UHMWPE surfaces. The interaction of adsorbed pro- teins and cell surface receptors, such as integrins, has a remarkable role in the mac- rophage interactions with biomaterials. Specifically, integrins participate in receptor-mediated phagocytosis of wear particles of different composition, includ- ing UHMWPE. The downstream effects of integrin-mediated interactions result in the production of pro-inflammatory cytokines and osteoclast activation, as shown by Zaveri et al. who have recently reported that Mac-1 integrin and RGD-binding integrins are involved in osteolysis induced by UHMWPE particles. Macrophage activation can occur not only by phagocytosis of UHMWPE particles but also by cell contact through receptor-mediated mechanisms, including Toll-like receptors (TLRs), and the cluster of differentiation molecules CD11b and CD14. Thus, Maitra et al. reported that UHMWPE particles stimulate in vitro TLR1/2 and acti- vate phagocytosis. In a PE particle-induced osteolysis murine calvarial model, TLR2 and TLR4 were found highly expressed in macrophages. TLRs act pri- marily through the adapter protein myeloid differentiation primary response protein 1 Osteolysis After Total Hip Arthroplasty: Basic Science 7 (MyD88) and induce activation of nuclear factor kappa-B (NF-кB), mitogen- activated protein kinases (MAPK) and interferon-regulatory factors (IRFs), leading to the release of pro-inflammatory cytokines, growth factors and chemokines [22, 24]. TLRs are activated by pathogen- or damage-associated molecular patterns in response to infection or tissue damage. Oxidative stress, e.g. oxygen intermediates and free radicals, may provoke degradation of UHMWPE resulting in products as alkane polymers. These degradation products could influence the “original” inmu- nogenecity of particles, altering their binding affinity to TLRs or other molecules in the cells surfaces and thereby activating endogenous signaling systems. Moreover, particulate debris affects the host tissue integrity by increasing cell death, which generates danger signals such as heat shock proteins that further increase TLR acti- vation. The inflammasome has been recently involved in the cellular activation by wear particles [17, 61]. Inflammasome activation depends on reactive oxygen species (ROS), enzymes release and other danger signals. Upon phagocytosis of UHMWPE particles, endosomal and lysosomal damage results in release of cathepsins which trigger the inflammasome activation. If particles are larger that 20 μm, multinucle- ated giant cells may activate also NADPH oxidases and generate ROS, also contrib- uting to its activation. After the initial interaction with macrophages, particles induce an active infiltra- tion of inflammatory cells into the periprosthetic area. Migration is mediated through chemotactic factors such as interleukin-8 (IL-8), macrophage chemotactic protein-1 (MCP-1), and macrophage inflammatory protein-1 (MIP-1) released by inflammatory and mesenchymal cells, among others, upon challenging with parti- cles. Thus, the pro-resorptive environment is created not only by locally acti- vated cells but also by migration of macrophages and osteoclast precursors, i.e. monocytes. In this regard, in vivo studies employing UHMWPE particles have shown the contribution of chemotactic factors released by macrophages and mesen- chymal stem cells (MSCs) to cell recruitment. Specifically, the CCR2/MCP-1 and the CCR1/MIP-1α ligand/receptor axes are involved in the systemic recruit- ment of macrophages and MSCs, respectively, in the presence of UHMWPE parti- cles [60, 63]. The most active field of research during the last decade has been focused in the identification of factors associated to the destruction of the bone tissue when UHMWPE particles are present. For instance, in vitro stimulation of macrophages with these particles increases the expression of genes involved in inflammation and osteoclastogenesis such as MMP9 (coding for matrix metalloproteinase-9), CTSK (coding for cathepsin K protein), CALCR (coding for calcitonin receptor) and TNFRSF11A (coding for receptor activator of nuclear factor kappa-B protein (RANK)). In vivo, calvarial models of PE particle-induced osteolysis have corrobo- rated these findings. Production of factors involved in bone destruction depends on the size and con- centration of wear particles. Green et al. showed that, for a given tested concentra- tion, PE particles of 0.24 μm in length induce higher production of TNF-α, IL-1β, 8 G. Vallés and N. Vilaboa IL-6, and PGE2 in macrophages than larger particles. Dose- and size-dependent effects were also reported, as sizes range between 0.45 and 1.71 μm were the most reactive when higher concentrations were tested. A recent study has shown that treatment of peripheral blood mononuclear cells with UHMWPE particles below 50 nm does not induce the release of TNF-α, IL-1β, IL-6 and IL-8. Regarding concentration, UHMWPE particles showed a dose-dependent induction in the production of TNF-α, IL-1β and IL-6 in macrophages, and in the production of RANKL in osteoblasts [16, 51, 65]. PE particles are able to regulate prolifera- tion and function of osteoblastic or bone-forming cells, through induced expres- sion of pro-osteoclastogenic factors RANKL, IL-6, IL-8, PGE2 and macrophage colony-stimulating factor (M-CSF) and also through the interference in the regula- tion of matrix synthesis proteins such as collagen or alkaline phosphatase (ALP) [16, 66, 67]. Differentiation of osteoblastic cells into a mature, osteocyte-like phe- notype is tighly regulated by several genes including runt-related ranscription factor-2 (Runx2), sclerostin (SOST) and osterix (Osx) whose expression is also altered in the presence of PE particles. In osteoprogenitor cells, UHMWPE parti- cles inhibit in a dose-dependent manner proliferation and osteogenic differentia- tion. Effects of PE particles on osteoblasts are further influenced by their maturation state. A recent study has detected increased expression of cata- bolic markers incuding matrix metalloproteinase-13 (MMP-13), carbonic anhy- drase 2 (CA2), cathepsin K, and tartrate resistant acid phosphatase (TRAP) in human primary osteocyte-like cultures exposed to PE particles. Moreover, in vitro exposure of macrophages to these particles induces spontaneous differen- tiation into mature and active osteoclasts. In other coexisting cells in the peri- prosthetic bone bed, studies are scarce. For instance, in fibroblasts, dominant cell type in the interfacial membrane, PE particles induce the expression of the pro- inflammatory cytokine IL-6. The role of these cells in wear-induced osteolysis has been mainly attributed to their interaction and cooperation with macrophages to amplify inflammation, fibrosis and osteoclast activation. Macrophages and fibroblasts in the interfacial tissues overexpress macrophage migration inhibitory factor (MIF), which can up-regulate the expression of pro-inflamatory factors and matrix metalloproteinases (MMPs) involved in the periprosthetic bone tissue destruction. New lines of evidence have addressed the role of dendritic cells (DCs) in UHMWPE particles-induced osteolysis, with a similar role than macro- phages. Research efforts have recently focused in how to fit the paradigm of polarization of macrophages when exposed to wear particles. Wear particles contribute to the creation of a periprosthetic environment in which macrophages can be polarized to M1 (pro-inflammatory) and M2 (anti-inflammatory) phenotypes. Both phenotypes have been observed and extensively characterized [17, 60]. Polarization of uncom- mitted M0 or M1 macrophages toward the M2 phenotype, that promotes bone heal- ing, has been proposed as therapeutic strategy to decrease the local inflammation. In a calvaria model employing UHMWPE particles, bone resorption was reduced after administration of the M2 phenotype inducer interleukin-4 (IL-4). 1 Osteolysis After Total Hip Arthroplasty: Basic Science 9 Broad-scale expression profiling of human macrophages challenged to UHMWPE particles revealed changes in the expression of genes related to inflammatory response, cell proliferation, cytokine-mediated signaling, response to stress, cell migration and death. Among others, particles modulated the transcript levels of genes encoding factors with remarkable roles in osteoclastogenesis and bone resorp- tion such as IL-8, MIP-1α, MIP-1β, macrophage inflammatory protein-3 alpha (MIP-3α), interleukin-23 (IL-23), M-CSF, IL-1α and vascular endothelial growth factor (VEGF); and cell surface receptors involved in the recognition of particles such as β-integrins, protein-coupled receptors, intercellular adhesion molecules, TNF receptor superfamily members and TLRs-signaling. Moreover significant up- regulation of the expression of genes encoding MMPs, including MMP-1 and MMP- 19, was also found. Overall, these findings show that inflammation and osteoclastogenesis-related mechanisms activated by UHMWPE particles are main processes involved in the pathogenesis of wear-induced osteolysis. The introduction of highly crosslinked polyethylene (HXLPE) to reduce wear and osteolysis in total joint arthroplasty has attracted great interest. Moreover, as compared to polymeric and metallic particles, HXLPE particles induce a moderate degree of peri-implant osteolysis. Metallic Particles Metal particles are massively generated from the bearing surfaces of MoM hip replacements, which were conceived as alternative to metal-on-polyethylene (MoP). However, adverse long-term effects are associated to MoM, including a higher rate of failure as compared to other bearing surfaces. Metals are susceptible to deg- radation upon exposure to extracellular tissue fluids, and therefore the clinical out- come and durability of implants are affiliated to particulate corrosion and wear products. Metal wear particles arise mainly from the bearing surfaces but also from the metal back and fixation screws of the acetabular cup [45, 73]. Under the optical microscope, they appear as black to brownish colored entities, with amorphous or irregular shapes (flakes or needles) and sharp edges, and sizes ranging from 0.1 to 5 μm. Ultrastructural methods showed that the majority of metal particles retrieved from periprosthetic tissues and joint simulators are in the nanometric range [3, 74]. These nanoparticles have a greater relative surface area than mic- roparticles and are potentially more chemically and biologically reactive. About 6–250 × 1012 metal particles are released per year from a MoM articulating surface. As particles with other compositions, metallic particles stimulate inflammation and bone resorption in detriment of bone formation. Resorption areas associate to metal particles and periprosthetic tissues are characterized by express inflammatory mediators including IL-8, IL-1β, macrophage inflammatory protein-2 alpha (MIP-2α), stromal cell-derived factor 1 (SDF-1) and its receptor CXCR4 [9, 76, 77]. 10 G. Vallés and N. Vilaboa In vitro, exposure to Ti particles induces macrophages to release TNF-α, IL-6, IL-1β and PGE2 in a process mediated by tyrosine phosphorylation and MAPK pathway. A recent factor associated to induced inflammation by Ti particles is the cannabinoid receptor type 2 (CB2), inductor of osteoclastogenesis. Similar to described in PE particles, dose- and size-dependent responses have been observed and the release of pro-inflammatory factors can occur independently of phagocytosis-dependent mechanisms. Concentration-dependent effects have been detected for TNF-α, IL-1β and IL-6. Apart from inflammatory factors, the expression of genes encoding osteoclastogenic markers can be induced by Ti par- ticles, including TRAP, nuclear factor of activated T-cells 1 (NFATC1, also termed NFAT2), cathepsin K and RANK, as well as nitric oxide synthase 2 (NOS2), NF-кB and MMP-9. By regulating the expression of genes involved in the superoxide dis- mutase pathways, Ti particles also modulate oxidative stress. Regarding che- motactic factors, in vitro exposure of primary human monocytes/macrophages to Ti particles increased the production of MIP-1α, resulting in increased monocyte migration. Other chemokines induced upon exposure to Ti particles in osteoblasts and osteoclasts are CCL17 and CCL22. Interestingly, the expression of the gene encoding CCR4, receptor of both chemokines, is up-regulated in osteoclast precur- sors exposed to Ti particles which accounts for macrophage recruitment and bone loss. In osteoblastic cells, metal particles modulate the expression of genes encoding pro-inflammatory and bone-resorbing factors. In vitro studies have shown that Ti particles decrease OPG and induce IL-6 production, effects associated to increased nuclear factor IL-6 (NF-IL-6) and NF-кB activation while production of MMP-2 increases through p38 signaling [9, 16, 80]. Ti particles severely impact on the via- bility and osteogenic potential of osteoblast precursors [16, 81]. Moreover, Ti par- ticles produce adverse effects in MSCs that include toxicity and increased IL-8 production. Induction of genes encoding pro-apoptotic proteins and down-regulation of those encoding anti-apoptotic and osteogenic factors has been detected in MSCs challenged with Ti particles. Altogether, these findings indicate that Ti particles impair the viability, proliferation and differentiation ability of MSCs. Exposure of fibroblasts to Ti-based particles induce the expression and/or production of RANKL, IL-6 and MCP-1 in a dose-dependent manner and stimulates the release of osteo- lytic enzymes as stromelysin and collagenase [3, 9, 16]. In human synovial cells, Ti particles increase MMP-2 activity. Bacterial endotoxins are detected frequently and in a significant amount adsorbed to metallic wear particles [57, 75]. In macrophages, exposure to Ti particles adsorbed to LPS results in the regulation of TLRs-mediated responses and the stimulation in the production of the proinflammatory cytokines TNF-α, IL-1β and IL-6. Nonetheless, endotoxin-free Ti particles activate TLRs-mediated pathways and induce the expression of same genes. However, comparative studies have shown that endotoxin-free Ti particles induce inflammation and osteolysis to a lower extent than those with adsorbed bacterial debris. As observed with UHMWPE particles, NALP3 inflammasome can be activated upon Ti particles internalization and subsequent cathepsin release. 1 Osteolysis After Total Hip Arthroplasty: Basic Science 11 In response to implant metal debris challenge, macrophages adopt a pro- inflammatory M1 phenotype [16, 79]. In a study to clarify whether macrophage phenotypes are equally sensitive to Ti particles, these cells were polarized towards phenotype M0, M1 and M2 and then incubated with Ti particles. While no signifi- cant effects were observed in M2-macrophages after Ti particle challenge, M1-macrophages experienced drastic changes both at the transcriptome and the proteome levels, with a notable increase in the production of inflammatory chemo- kines (e.g. MCP-1, MIP-1α, IL-8), cytokines (TNF-α, IL-1β) and growth factors (e.g. granulocyte-macrophage colony-stimulating factor (GM-CSF), granulocyte- colony stimulating factor (G-CSF) and epidermal growth factor (EGF)). This study supports again the notion that the local microenvironment, which determines the macrophage phenotype, notably influences the response to particles. Changes in mechanotransduction and adhesive properties of cells treated with metal particles have also been addressed in in vitro studies. For example, Preedy et al. observed that Ti particles increase osteoblast elasticity. In vivo studies employing calvaria or air pouch models have corroborated the in vitro findings regarding Ti particles induction of TNF-α, cyclooxygenase-2 (COX-2), IL-1β, MMP-9, MCP-1, RANK, RANKL, NFATC1, VEGF and CB2 expression and/or production [9, 16]. Similar results were observed when Ti-alloy particles were investigated. Concerns about wear debris derived from CoCr are mainly based on ions release. Co and Cr ions can travel through lymh and blood with harmful consequences for heart, brain and thyroid, spleen or liver. CoCr alloy particles have been histo- logically identified in necrotic areas with infiltrating macrophages and lymphocytes. In the local tissues, Co-Cr alloy particles and ions impair the expression of proteins related with osteoblastic differentiation (OPG, Osx and osteocalcin (OCN)) and increase those related with inflammation and osteoclastogenesis (IL-6, RANKL, MCP-1 and NFATC1). Co ions affect osteoblasts and neutrophils functions and stimulate chemokine secretion in both cell types. Ions released from a CoCr alloy (CoCr29Mo6) induced necrosis in osteoblasts and peripheral blood mononu- clear cells (PBMCs) and also stimulated IL-6, IL-8, and MMP-1 expression in these cell types. Co and Cr ions are highly cytotoxic for macrophages and lympho- cytes, inducing apoptosis. Exposure to CoCr particles, increase expression and/ or production of pro-inflammatory factors (IL-1α, IL-6, interleukin-10 (IL-10), IL-8, GM-CSF and PGE2) in monocyte-macrophage lineage cells, reduce viability in histiocytes and fibroblasts and affect osteogenic differentiation in MSCs [9, 16, 90]. Treatment of THP-1 cells with a CoCr alloy (ASTM F75) particles increase the production of TNF-α, IL-1β and IL-8 through TLR4 signaling pathway. In fibroblasts, CoCr nanoparticles increase the production of ROS and induce apopto- sis [9, 92]. Regarding size, CoCr nanoparticles release more ions and are more cytotoxic than CoCr microparticles. After being phagocytosed by macrophages metal nanoparticles trigger endoplas- mic reticule (ER) stress. Periprosthetic tissues express large amounts of ER stress- associated molecules (Ca2+, IRE1-α, GRP78/Bip, CHOP, cleaved Caspase-4, and JNK). It has been speculated that apoptosis in macrophages challenged to metal 12 G. Vallés and N. Vilaboa particles might be mediated by ER stress pathways, also linked to inflammation and osteoclastogenesis. Similar to UHMWPE particles, chemical changes in metal particles surface altere the recognition of particles by cells and their subsequent effects [9, 95]. Also, release of endogenous alarmins has been proposed to contribute to positive feedback mechanisms in the periprosthetic tissue challenged with metal- lic debris [84, 18]. Several studies report a scarce number of T-lymphocytes in periprosthetic tis- sues, concluding that osteolysis occurs independently of these cells. However, lymphocytes play a key role in hypersensitivity reactions leading to early osteoly- sis [45, 51]. Hypersensitivity or allergy to metallic components is a matter of con- cern to orthopaedic surgeons. This reaction is usually a cell-mediated (type-IV delayed hypersensitivity) response, characterized by activation of delayed-type hypersensitivity T lymphocytes by haptenic antigen and cytokine release which leads to recruitment of cytotoxic T-cells and macrophages activation. Activated macrophages mediate delayed-type hypersensitivity T lymphocytes activation, self-perpetuating the inflammatory response [9, 79]. Another adverse local tissue reaction is the aseptic lymphocyte-dominated vasculitis-associated lesions (ALVaL). This response is similar to the type IV hypersensitivity response, charac- terized by inflammation accompanied by lymphocytic infiltration, accumulation of plasma cells and macrophages and soft tissue necrosis [3, 9, 96]. Pseudotumors, mainly affecting soft tissues, are another complication of metal THA. Pseudotumors are identified as a solid or cystic mass-forming tissue characterized by necrosis areas, mononuclear cell infiltration and giant multinucleated cells, with high degree of perivascular lymphocytic aggregation. The prevalence of pseudotumors in hip implanted patients is a controversial issue. While some authors estimated a low frequency, just about a 1% within 5 years of MoM implantation, others have found a much higher incidence, up to 60% [3, 9, 97]. This absence of consensum may be explained due to the asymptomatic nature in some cases and the similarity with other adverse reactions. In this regard, Catelas et al. have stated relevant dif- ferences in the proteome of pseudotumors and osteolytic tissues which correlate with predominant adaptive immune responses in patients with pseudotumors and innate immune responses in patients with periprosthetic osteolysis. One molecular mechanism which might explain the local soft tissue growing around the implant (fibro-pseudotumors) is the induction of hypoxia and angiogenesis by metal debris, which increases the levels of transcription factor hypoxia inducible factor 1 alpha (HIF-1α) and VEGF. Finally, some studies suggested an asso- ciation between MoM implants and risk for developing cancer but epidemiological studies have not been able to prove increased incidence of cancer in patients with these implants [9, 99]. In summary, existing data employing metal and metal-alloys wear debris and their byproducts indicate their involvement in the induction of adverse local tissue reactions, based not only in the activation of inflammation and bone resorption- related mechanisms but also in the induction of the oxidative stress and cytotoxicity. These effects are mainly dose- and concentration-dependents which, in the context of metal particles exhibiting submicrometric and nanometric sizes and being 1 Osteolysis After Total Hip Arthroplasty: Basic Science 13 released in high amounts to the periprosthetic space, represent a clear threat to tis- sue homeostasis. Ceramic Particles Ceramics present chemical and mechanical advantageous properties for the manu- facturing of orthopaedic devices, especially for young patients, including biochemi- cal inertness, hardness, high-strength and corrosion and wear resistance [3, 100, 101]. Currently, the most used ceramic materials in clinical practice are alumina (AL2O3) and zirconia (ZrO2). Hip implant revisions of Al2O3-based components have been associated to their brittleness and the subsequent risk of catastrophic failures due to deficiencies in the manufacture [3, 102]. A great variability in the frequency of ceramics fracture rate has been reported but in general, incidence is small [3, 103]. Wear volume of ceramic bearing couples is lower than that of metal- lic and therefore a lower risk of revision is expected [3, 104]. Moreover, PE compo- nent exhibits a lower linear wear in CoP (0.034 mm/year) than in MoP (0.1 mm/ year) bearings. On crosslinked PE, ceramics display a wear rate of 0.019 mm/year compared to 0.03 for metals. Relative inertness and low abundance of ceramic wear debris in the periprosthetic space imply limited adverse biologic reactions and risk of osteolysis associated to this type of material. Ceramic debris appears as fine greyish-brown particles (ZrO2) and brown, brownish-green or black granules (Al2O3) [45, 105, 106]. Regarding size, ceramic particles are similar to metal particles, and ten times smaller than polymeric parti- cles. More in detail, some studies have shown a range from 0.13 to 7.2 μm in tissues around loosened CoC hip implants and others have reported a bimodal size distribu- tion, with most of them in the nanometric-order (5–90 nm) and submicron- to micron-scale for the rest (up to 3.2 μm) [3, 106, 107]. Manufacturing defects, instability or mal position of hip prosthesis components are main factors involved in the generation of ceramic particles, which once pro- duced are “potential” inducers of periprosthetic osteolysis [3, 60, 108]. In fact, pro- duction of ceramic debris associated to occasional osteolytic areas has been considered as the consequence of mechanical instability rather than the origin of failure. Typical foreign body reaction was only observed in association to large amounts of wear particles. The cellular mechanisms involved in the biological response to ceramic particles are a controversial issue, not fully eluci- dated. While some authors state that ceramics are able to activate cells in a similar manner to metallic debris due to their comparable size, others express doubts about the involvement of the same mechanisms. In fact, studies in retrieved tissues have shown a differential cellular response to accumulated ceramic wear debris. Biocompatibility of ZrO2 particles seems to be greater than that of Ti particles since induction of pro-inflammatory gene expression was significantly lower in macro- phages challenged with ZrO2. In vivo experiments support these findings, with higher extent of inflammation and bone resorption induced by Ti or PE particles 14 G. Vallés and N. Vilaboa than ceramics [83, 111]. Bylski et al. exposed THP-1 macrophage-like cells to dif- ferent concentrations of Al2O3 and Ti particles and found that, regardless of particle size and time exposure, ceramic caused only minor up-regulations of RANK, TNF-α and OPG mRNA levels while Ti highly stimulated the expression these genes and led to cytotoxic effects in a dose- and time-dependent manner. Also, minor induction in the production of pro-inflammatory proteins such as IL-1β and MCP-1 has been reported after exposure of primary human macrophages to Al2O3 particles. In a comparative study using murine macrophage cells treated with PE or ceramic particles, the latter led to lower release of TNF-α. The negligible in vitro effects of Al2O3 particles on cell viability and cytokines production may account for the low incidence of osteolysis in patients with CoC prosthesis. Once again, particle size, composition and concentration are rele- vant factors to consider [114, 115, 116]. Regarding induction of pro-inflammatory cytokines, neither Al2O3 or ZrO2 particles induced IL-1 or IL-6 production in human fibroblast-like synoviocytes isolated from patients with osteoarthritis or rheumatoid arthritis. Al2O3 and ZrO2 are susceptible of internalization in J774 cells, induc- ing both type of particles the release of TNF-α at same extent. Exposure of human macrophages to ZrO2 or TiO2 nanoparticles upregulated the expression of TLR7 and TLR10. Moreover, ZrO2 nanoparticles induced IL-1β production and IL-1Ra synthesis while in LPS-treated macrophages IL-1Ra release is reduced, therefore promoting the creation of a pro-inflammatory environment, amplifying the M1 macrophage-effector functions. A recent study has evaluated the ZrO2 effects on osteoclasts, whose recruitment and activation are directly involved in the periarticular osteolysis. Exposure of osteoclasts to ZrO2 increased cell fusion and expression of osteoclast function- and bone matrix-related proteins including vitronectin receptor (VNR), TRAP, RANK and cathepsin K without stimulating osteoclast bone resorptive capacity. Moreover, higher expression of MMP-1 and an impaired production of the tissue inhibitor of this metalloproteinase were observed. AL2O3 particles can be internalized by osteoblastic MG-63 cells which resulted in decreased proliferation, ALP activity and TGF-β1 secretion. In contrast, exposure of these cells to ZrO2 stimulated proliferation and ALP activity. Interestingly, both types of particles induced the production of PGE2 in a dose- dependent manner. Treatment of primary human osteoblasts with Al2O3 induced the expression and secretion of IL-6 and affected the osteoblastic function by decreasing the C-terminal type I procollagen (PICP) secretion and ALP activity. The paracrine interactions between macrophages and osteoblasts were also affected by treatment of these cell types with Al2O3 particles which resulted in increased production of IL-6 and GM-CSF as assessed in a a co-culture in vitro model. Another study examined the cross talk between macrophages and osteoblasts in the presence of Al2O3 particles employing media conditioned by osteoblasts exposed to particles in which a slight decrease in OPG-to-RANKL ratio was detected. In this study, secretion of TNF-α, IL-6 and GM-CSF by PBMCs was not induced by culturing these cells with media conditioned by osteoblast 1 Osteolysis After Total Hip Arthroplasty: Basic Science 15 exposed to Al2O3. Osteoclasts formation assays showed an increase in TRAP- positive aggregates which suggested the activation of osteoclastogenesis although osteoclast generation was not enhanced in PBMC cultures exposed to conditioned medium of osteoblasts challenged with Al2O3. In summary, although ceramics present advantages in comparison to other mate- rials, they still seem able to elicit an adverse local reaction. However, the moderate wear rate of ceramic components together with the limited effects of ceramic particles-induced biological responses may significantly account for the low inci- dence of osteolysis in patients bearing this type of prosthesis. Cement Particles PMMA debris arise from the fragmentation of the cement used to fix components of THA [3, 126]. Bone cement is frequently applied to the femoral stem but also the acetabular shell may be fixed to the adjacent bone. PMMA-based bone cement was introduced by Charnley as an effective mean to achieve stable fixation between the bone bed and the implant, and later as matrix for local delivery of antibiotics. Apart from favouring formation of a fibrous interfacial tissue, PMMA experiences brittleness and shrinkage, lack of adherence to the bone and during its application, the in situ exothermic polymerization reaction can damage the adjacent bone tissue. In general, cemented prostheses are not options for young and active patients, with good bone stock quality, as PMMA can cause third body wear and secondary loosening of the hip components. PMMA third-body abrasive particles originated from the failure of the cement mantle can induce surface damage, especially relevant in PE and Ti-based components [3, 128]. ZrO2 and BaSO4 are used as radio-opaque contrast media in bone cement, and therefore disintegration of the cement mantle can produce PMMA and/or ZrO2 or BaSO4 particles which can contribute to direct cell responses and also to third-body wear [3, 126]. Acrylic particles found in the capsular tissue present a size range from 1–2 μm to several hundreds of microns. Several shapes have been observed, being the smallest particles similar to dust granules and the largest ones like pearls clusters or grapes bunch. Under light microscopy, particles present a size between 0.5 and 2 μm with grey or yellow brown colour and under polarized light, they show slight white birefringence. Localized areas of osteolysis exhibiting foreign body response to cement parti- cles are frequently observed. PMMA, ZrO2 and BaSO4 particles of phagocytos- able size can be internalized and stored within macrophages while the non-phagocytosable large cement fragments are surrounded by foreign body giant cells. Aggressive granulomatous lesions as well as non-granulomatous AL around cemented total hip prostheses have been observed. PMMA particles are also able to activate the lymphocyte-mediated immune response. 16 G. Vallés and N. Vilaboa Cell surface receptor complement receptor 3 (CR3) is involved in the phagocy- tosis and activation of signaling pathways of macrophages exposed to PMMA. Production of inflammatory mediators is also mediated by TLRs in a manner partly dependent on MyD88 signaling pathway, since inhibition of this adaptor molecule decreased the induced production of TNF-α in RAW 264.7 murine macrophages exposed to PMMA. As described for polymeric and metallic particles, PMMA particles activate the NALP3 inflammasome. Phagocytosed cement particles induce caspase-1 activation in monocyte/macrophage cells and the release of down- stream effectors IL-1β and TNF-α, both involved in the amplification of osteoclas- togenesis mediated by RANKL. In vitro studies suggest that PMMA particles may have more potent osteolytic effects than high density PE particles. In fact, the activation of macrophages by PMMA particles has long been considered as a key mechanism in wear-induced osteolysis of cemented implants [3, 48]. Apart from peri-implant resident cell acti- vation, PMMA particles are also able to recruit peripheral monocyte/macrophages, promoting the systemic trafficking of macrophages to the site of inflammation , as observed after PMMA injection in the medullary canal of nude mice and the intravenous administration of RAW264.7 macrophages stably expressing a biolumi- nescent reporter gene. This finding was corroborated using a severe combined immunodeficiency mouse chimera model, in which fragments of periprosthetic granulomatous tissues and bone chips retrieved during revision surgery in loosened patients were implanted in mice. PBMCs isolated from patients during revi- sion surgery were fluorescently labeled and cultured with cement particles before intraperitoneal injection. Fluorescent-labeled PBMCs challenged with PMMA par- ticles and a high number of TRAP-positive cells accumulated in transplanted peri- prosthetic tissues. The chemotactic effects induced by PMMA particles leading to migration of human monocyte and MSCs have been studied in in vitro studies [60, 79]. Macrophages challenged with cement particles increase MCP-1 release. Like MCP- 1, MIP-1α is expressed in periprosthetic tissues and produced by cells of the mono- cytic/macrophagic lineage after priming with PMMA particles. Moreover, neutralizing antibody to MIP-1α lessened the migration of monocytes induced by media conditioned by macrophages exposed to PMMA particles. An indepen- dent study confirmed the involvement of MCP-1 and MIP-1α induced by PMMA particles in the homing of monocytes and MSCs, suggesting the involvement of various chemokines in the recruitment of macrophages and MSCs. Fibroblasts, a cell source of chemokines, increase MCP-1 release upon exposure to PMMA particles. Exposure of macrophages to PMMA increases the expression of pro-inflammatory cytokines, via activation of the NF-κΒ pathway [137, 138]. Among other macrophage-releated cell types, dose- and time-dependent induction of the secre- tion of IL-1 and PGE2 has been observed in peritoneal macrophages while induction in the release of IL-1β and TNF-α was described in bone marrow macrophages. In line with this, Pearle and colleagues performed microarrays profiling of monocytes and unfractionated PBMCs exposed to PMMA and Ti par- 1 Osteolysis After Total Hip Arthroplasty: Basic Science 17 ticles to assess, respectively, the activation of the innate immune system and the innate and adaptive immune system. Exposure of monocytes to PMMA induced the transcript levels of TNF-α, IL-1α, IL-1β, IL-6, IL-8 and COX-2, key regulator of PGE2 synthesis, as well as the chemokines MIP-3α and CCL11. Similar data were obtained in PBMC exposed to PMMA. Comparison with data obtained from cells exposed to Ti particles suggested that PMMA-inflammatory effects are mediated by the activation of the innate immune system, i.e., monocytes, in a T cell- independent manner, while lymphocytes are not essential mediators in PMMA- induced osteolysis. Apart from the induction in the inflammatory response, PMMA particles contrib- ute to mononuclear phagocytes and macrophages differentiation into TRAP-positive cells with resorptive capacity [131, 141]. Other osteoclastic phenotypic markers might be regulated by PMMA particles, as murine RAW264.7 cells exposed to PMMA upregulated cathepsin K and RANK expression. PMMA particles stimulate osteoclastogenesis, at least in part, by induction of RANKL and TNF-α expression and by activation of NF-кB. Signaling pathways that cooperate with RANKL-induced during differentiation of macrophages into osteoclasts, like MAPK pathway, are also activated. Expression and activity of the transcription fac- tor NFAT2, involved critically in osteoclast lineage commitment, increases in osteo- clast precursors exposed to PMMA particles. PMMA-derived effects on the osteoclastic lineage seem to be dependent on their stages of development, increas- ing the number of osteoclasts precursors and enhancing the number and bone resorption capacity of mature cells. As mentioned before, macrophages exhibit functional plasticity in order to adapt to the dynamic periprosthetic microenvironment. Macrophages challenged by PMMA particles exhibit characteristics of M1 phenotype. However, macro- phages may evolve from M1 to M2 phenotype as proven in in vitro experiments in which M1 macrophages were treated with IL-4 prior to exposure to PMMA parti- cles or were simultaneously treated with IL-4 and particles. Exposure to PMMA particles impairs viability, proliferation and osteogenic dif- ferentiation of osteoprogenitor cells. Transcript levels of the transcription factors Runx2, Osx and Dlx5 that orchestrate osteogenic differentiation, and OCN decrease after exposure of MC3T3-E1 to PMMA particles [138, 147]. Effects on osteopro- genitor proliferation and differentiation have been found to be dose-dependent. The more potent inhibitory effects of PMMA particles have been detected in the early phase of osteoprogenitor differentiation, as shown in cultures of murine bone marrow cells [148, 149]. PMMA-induced inhibition of osteoblastic differentiation is characterized by altered expression of genes encoding bone morphogenetic protein 3 (BMP3) and SOST, which are negative regulators of bone formation. Regarding mature bone-forming cells, proliferation and collagen synthesis of osteoblastic cells exposed to PMMA were reported to be inhibited whereas OCN and IL-6 production were stimulated. Additionally, apoptotic rates and MMP-1 expression were enhanced in osteoblasts exposed to PMMA. As observed with other types of particles, the mechanisms involved in osteoblasts dys- function after exposure to PMMA particles are not totally understood. Among other 18 G. Vallés and N. Vilaboa participating mechanisms, inhibition of MAPK activity and reduced TGF-β1 pro- duction, which play an essential role in differentiation and the response to environ- mental stress, have been proposed. A recent study has addressed the consequences of the physical stress stimuli that PMMA particles impose to osteo- blasts that include an increase in the elastic modulus with time, production of calcium and changes in cytoskeleton organisation which influence cell behaviour and function. Addition of radio-opaque agents favors macrophage-osteoclast differentiation and their resorptive activity, being more resorptive PMMA contain- ing BaSO4 than ZrO2. Moreover, exposure of osteoblastic cells to cements containing these radio-opaque agents increased the expression ratio RANKL/OPG. Such imbalance might be related to stimulation of osteoclast differentiation and inhibition of osteoclast apoptosis. Collected evidence indicates that cement-derived wear particles elicit important adverse cellular reactions that lead to to periprosthetic osteolysis. Cement particles are found in high proportion in the periprosthetic bone bed which facilitates their direct action and severe effects on bone and immune cells. Perspectives Research efforts in THR have been oriented toward the improvement of the tribol- ogy of materials used in prostheses manufacturing as well as to the elucidation of the biological processes triggered by wear particles. Important advances in orthopaedic materials concerning their microstructure, surface characteristics and/ or design have been made and multiple biomaterials have been generated as promis- ing alternatives including advanced composites and hybrid materials [155–157]. Among others, coatings of the material surfaces with bioceramics or functionaliza- tion with extracellular matrix proteins, biological peptides or growth factors are expected to stimulate physiological mechanisms that counterbalance the develop- ment of osteolysis [50, 158]. However, novel materials still face issues regarding their long-term performance and biological response to wear and corrosion debris. Moreover, a deeper understanding of the local and systemic biological responses to materials is needed. The identification of signaling pathways and cellular and molecular mediators that contribute to periprosthetic osteolysis will facilitate not only the generation of mimetic, self-diagnosing and multifunctional materials but also the development of targeted and personalized therapeutic strategies. Innovations in genotyping, pharmacogenomics and large-scale molecular phenotyping will facilitate the identification of the mechanisms involved in the pathogenesis of oste- olysis that then will be used to design strategies for the diagnosis, prevention and treatment of AL [24, 159]. For instance, recent progresses in the understanding of the signaling pathways that integrate ER stress, apoptosis, inflammation or osteo- clastogenesis have been proposed as promising therapeutic targets to mitigate wear particle-induced osteolysis [94, 160]. Acting upstream of the inflammatory cascade activated by wear particles might lead to better therapeutic control of the local 1 Osteolysis After Total Hip Arthroplasty: Basic Science 19 process, in opposition to systemic management which might be associated to adverse side-effects. Three main events triggered by the presence of particles can be targeted: cellular chemotaxis, polarization of macrophages and NF-κΒ signaling [60, 85, 161, 162]. Protein phosphatase 2A, a major serine-threonine phosphatase involved in NF-кB and c-Jun N-terminal kinase signaling pathways has been pro- posed as new target for pharmacological intervention in Ti-induced osteolysis. Inhibition of the glycogen synthetase kinase 3 beta, regulator in the canonical Wnt signaling pathway which is essential in the maintenance of normal bone mass, has been suggested as a target of molecules to treat wear-debris induced osteolysis. Other proposed targets explored are bone morphogenetic proteins and protein kinase C. Therefore, the more we know about intracellular signaling activated by wear particles, the more options we have to develop therapeutic inter- ventions for aseptic implant loosening. Other therapeutic intervention has focused in controlling the levels of RANKL and OPG. Specific materials, such as biosilica and microstructured Ti surfaces, are able to stimulate the endogenous production of OPG. Treatments with anti- resorptive bisphosphonates (e.g. alendronate and zolendronate), RANKL antibodies or OPG-like molecules have been proposed. Some of these drugs have proven their efficacy in pathologies associated to catabolic bone disorders, but might have an important impact at systemic level. Unwanted side effects have been also associated to anti-inflammatory drugs as corticosteroids or TNF-α antagonists or IL-1 antago- nists, which have been suggested to treat wear-induced osteolysis. Other phar- macological agents assayed include the synthetic molecules OA-14 ((N-(3-(dodecylcarbamoyl)phenyl)-1H-indole-2-carboxamide)) and metho- trexate or the ursolic acid. An active research has been performed testing agents able to modulate the NF-кB signaling pathway, including natural bioactive compounds such as trip- tolide, notoginsenoside R1 or sophocarpine [170–172], antibiotics as rifampin , bioflavonoids such as anthocyanin or amentoflavone, [174, 175], compo- nents of the omega-3 fatty acids such as the docosahexanoic acid or the pro- biotic Lactobacillus casei. In this search of modulators of NF-кB, the hormone melatonin has been reported as candidate for the treatment of wear debris-induced osteolysis. Sirtuin 1, NAD(+)-dependent histone deacetylase which regulates the transcriptional activity of NF-κB, has been considered as a pharmacological target in osteoblast and macrophages challenged to metal particles [179, 180]. Statins such as simvastatin and pitavastatin, employed as lipid-lowering medica- tion, have been also proposed for the prevention and/or treatment in wear particle- induced bone resorption. Our group reported that simvastatin down-regulates IL-6 secretion in osteoblastic cells cultured in isolation or co-cultured with macrophages and exposed to Ti particles. In order to improve the local efficacy of anti- inflammatory and/or anti-osteolytic therapeutic agents different methods to concen- trate the drug preferentially into the inflammatory area have been proposed, avoiding or reducing systemic exposure. In this regard, local delivery of dexamethasone con- jugated to the copolymer HPMA or covalently conjugated to TiO2 particles has been assayed [182, 183]. 20 G. Vallés and N. Vilaboa Gene therapy has also emerged as a potential therapeutic avenue. For instance, in vitro and in vivo models have shown the effectiveness of viral gene delivery of IL-10, IL-1Ra and OPG in Ti- and UHMWPE-induced osteolysis models [184– 189]. Other therapeutic experimental approaches employed small interfering RNA to silence TNF-α, the catalytic subunit of phosphoinositide 3-kinase (PI3K) p110β or the chemokine receptor CXCR2 [190–192]. Although promising preliminary results have been achieved, the implementation of gene therapy into orthopaedic practice is still a distant possibility. Other important aspect to consider is the early diagnosis of wear particles- induced osteolysis. Therefore, efforts are focused in the search of biomarkers as diagnostic and prognostic tools to monitor the progression of the disease. Different biomarkers in synovial fluid, urine and serum have been proposed, but still none have proved relevant clinical utility [193, 194]. In this regard, our group has been focused during the last years in the identification of serum proteins with potential to be regarded as biomarkers. Concluding Remarks Wear particles-induced osteolysis is one of the major challenges for orthopedic sur- geons due to the absence of clinical signs and symptoms until late stages of destruc- tion and failure. The increasing demand of hip arthroplasties and the growing incidence in young patients, with predictions pointing to a substantial increase in revision surgeries, highlights that this issue has to be seriously considered. Currently, strategies are focused on improving the implant behaviour and limiting the biologi- cal response to its degradation products. Despite intense research efforts in the materials field, the long-term performance of novel biomaterials is still unknown. In the biomedical field, it is imperative to unravel the cellular and molecular mecha- nisms triggered by wear debris to establish effective medical interventions. Too many questions that still remain not answered and become major challenges for basic research groups. References 1. Learmonth ID, Young C, Rorabeck C. The operation of the century: total hip replacement. Lancet. 2007;370:1508–19. 2. Apostu D, Lucaciu O, Berce C, Lucaciu D, Cosma D. Current methods of preventing aseptic loosening and improving osseointegration of titanium implants in cementless total hip arthro- plasty: a review. J Int Med Res. 2017; https://doi.org/10.1177/0300060517732697. 3. Bitar D, Parvizi J. Biological response to prosthetic debris. World J Orthop. 2015;6:172–89. https://doi.org/10.5312/wjo.v6.i2.172. 4. Singh JA. Epidemiology of knee and hip arthroplasty: a systematic review. Open Orthop J. 2011;5:80–5. https://doi.org/10.2174/1874325001105010080. 1 Osteolysis After Total Hip Arthroplasty: Basic Science 21 5. Kremers HM, Larson DR, Crowson CS, Kremers WK, Washington RE, Steiner CA, et al. Prevalence of total hip and knee replacement in the United States. J Bone Joint Surg Am. 2015;97:1386–97. https://doi.org/10.2106/JBJS.N.01141. 6. Dobzyniak M, Fehring TK, Odum S. Early failure in total hip arthroplasty. Clin Orthop Relat Res. 2006;447:76–8. 7. Melvin JS, Karthikeyan T, Cope R, Fehring TK. Early failures in total hip arthro- plasty – a changing paradigm. J Arthroplast. 2014;29:1285–8. https://doi.org/10.1016/j. arth.2013.12.024. 8. Cherian JJ, Jauregui JJ, Banerjee S, Pierce T, Mont MA. What host factors affect asep- tic loosening after THA and TKA? Clin Orthop Relat Res. 2015;473:2700–9. https://doi. org/10.1007/s11999-015-4220-2. 9. Gibon E, Amanatullah DF, Loi F, Pajarinen J, Nabeshima A, Yao Z, et al. The biological response to orthopaedic implants for joint replacement: part I: metals. J Biomed Mater Res B Appl Biomater. 2017;105:2162–73. https://doi.org/10.1002/jbm.b.33734. 10. Jansen P, Mumme T, Randau T, Gravius S, Hermanns-Sachweh B. Endoglin (CD105) expres- sion differentiates between aseptic loosening and periprosthetic joint infection after total joint arthroplasty. Springerplus. 2014;3:561. https://doi.org/10.1186/2193-1801-3-561. 11. Mäkelä KT, Eskelinen A, Pulkkinen P, Paavolainen P, Remes V. Results of 3,668 primary total hip replacements for primary osteoarthritis in patients under the age of 55 years. Acta Orthop. 2011;82:521–9. https://doi.org/10.3109/17453674.2011.618908. 12. Landgraeber S, Jäger M, Jacobs JJ, Hallab NJ. The pathology of orthopedic implant failure is mediated by innate immune system cytokines. Mediat Inflamm. 2014;2014:185150. https:// doi.org/10.1155/2014/185150. 13. Sukur E, Akman YE, Ozturkmen Y, Kucukdurmaz F. Particle disease: a current review of the biological mechanisms in periprosthetic osteolysis after hip arthroplasty. Open Orthop J. 2016;10:241–51. https://doi.org/10.2174/1874325001610010241. 14. Pajarinen J, Lin TH, Nabeshima A, Jämsen E, Lu L, Nathan K, et al. Mesenchymal stem cells in the aseptic loosening of total joint replacements. J Biomed Mater Res A. 2017;105:1195– 207. https://doi.org/10.1002/jbm.a.35978. 15. MacInnes SJ, Gordon A, Wilkinson JM. Risk factors for aseptic loosening following Total hip arthroplasty. Recent Adv Arthroplast. 2012; https://doi.org/10.5772/26975. 16. Veronesi F, Tschon M, Fini M. Gene expression in osteolysis: review on the identification of altered molecular pathways in preclinical and clinical studies. Int J Mol Sci. 2017;25:18. https://doi.org/10.3390/ijms18030499. 17. Nich C, Takakubo Y, Pajarinen J, Ainola M, Salem A, Sillat T, et al. Macrophages-key cells in the response to wear debris from joint replacements. J Biomed Mater Res A. 2013;101:3033– 45. https://doi.org/10.1002/jbm.a.34599. 18. Gallo J, Goodman SB, Konttinen YT, Raska M. Particle disease: biologic mechanisms of periprosthetic osteolysis in total hip arthroplasty. Innate Immun. 2013;19:213–24. https://doi. org/10.1177/1753425912451779. 19. Camuzard O, Breuil V, Carle GF, Pierrefite-Carle V. Targeting autophagy to inhibit wear debris-induced osteolysis. AME Med J. 2017;2:5. 20. Yan Y, Hu J, Lu H, Wang W. Genetic susceptibility to total hip arthroplasty failure: a case- control study on the influence of MMP 1 gene polymorphism. Diagn Pathol. 2014;9:177. https://doi.org/10.1186/s13000-014-0177-9. 21. Towle KM, Monnot AD. An assessment of gender-specific risk of implant revision after primary total hip arthroplasty: a systematic review and meta-analysis. J Arthroplast. 2016;31:2941–8. https://doi.org/10.1016/j.arth.2016.07.047. 22. Gu Q, Shi Q, Yang H. The role of TLR and chemokine in wear particle-induced aseptic loos- ening. J Biomed Biotechnol. 2012;2012:596870. https://doi.org/10.1155/2012/596870. 23. Hallab NJ, Jacobs JJ. Biologic effects of implant debris. Bull NYU Hosp Jt Dis. 2009;67:182–8. 24. Terkawi MA, Hamasaki M, Takahashi D, Ota M, Kadoya K, Yutani T, et al. Transcriptional profile of human macrophages stimulated by ultra-high molecular weight polyethylene partic- 22 G. Vallés and N. Vilaboa ulate debris of orthopedic implants uncovers a common gene expression signature of rheuma- toid arthritis. Acta Biomater. 2018;65:417–25. https://doi.org/10.1016/j.actbio.2017.11.001. 25. Ulrich SD, Seyler TM, Bennett D, Delanois RE, Saleh KJ, Thongtrangan I, et al. Total hip arthroplasties: what are the reasons for revision? Int Orthop. 2008;32:597–604. 26. Howie DW, Neale SD, Haynes DR, Holubowycz OT, McGee MA, Solomon LB, et al. Periprosthetic osteolysis after total hip replacement: molecular pathology and clini- cal management. Inflammopharmacology. 2013;21:389–96. https://doi.org/10.1007/ s10787-013-0192-6. 27. Iorio R, Robb WJ, Healy WL, Berry DJ, Hozack WJ, Kyle RF, et al. Orthopaedic surgeon workforce and volume assessment for total hip and knee replacement in the United States: preparing for an epidemic. J Bone Joint Surg Am. 2008;90:1598–605. 28. McGrory BJ, Etkin CD, Lewallen DG. Comparing contemporary revision burden among hip and knee joint replacement registries. Arthroplast Today. 2016;2:83–6. https://doi. org/10.1016/j.artd.2016.04.003. 29. Nich C, Goodman SB. Role of macrophages in the biological reaction to wear debris from joint replacements. J Long-Term Eff Med Implants. 2014;24:259–65. 30. Girard J, Glorion C, Bonnomet F, Fron D, Migaud H. Risk factors for revision of hip arthro- plasties in patients younger than 30 years. Clin Orthop Relat Res. 2011;469:1141–7. https:// doi.org/10.1007/s11999-010-1669-x. 31. McGonagle L, Siney PD, Raut VV. Fate of the unrevised cemented stem following cup only revision: 227 hips at an average of 6 years follow-up. Orthop Traumatol Surg Res. 2015;101:781–4. https://doi.org/10.1016/j.otsr.2015.08.005. 32. Magone K, Luckenbill D, Goswami T. Metal ions as inflammatory initiators of osteolysis. Arch Orthop Trauma Surg. 2015;135:683–95. https://doi.org/10.1007/s00402-015-2196-8. 33. Jiang Y, Jia T, Wooley PH, Yang SY. Current research in the pathogenesis of aseptic implant loosening associated with particulate wear debris. Acta Orthop Belg. 2013;79:1–9. 34. Pajarinen J, Gallo J, Takagi M, Goodman SB, Mjöberg B. Particle disease really does exist. Acta Orthop. 2018;89:133–6. https://doi.org/10.1080/17453674.2017.1402463. 35. Bordini B, Stea S, De Clerico M, Strazzari S, Sasdelli A, Toni A. Factors affecting aseptic loosening of 4750 total hip arthroplasties: multivariate survival analysis. BMC Musculoskelet Disord. 2007;24(8):69. 36. Engh CA, Ho H, Powers CC, Huynh C, Beykirch SE, Hopper RH Jr. Osteolysis propensity among bilateral total hip arthroplasty patients. J Arthroplast. 2011;26:555–61. https://doi. org/10.1016/j.arth.2010.05.014. 37. Stelmach P, Kauther MD, Fuest L, Kurscheid G, Gehrke T, Klenke S, et al. Relationship between GNAS1 T393C polymorphism and aseptic loosening after total hip arthroplasty. Eur J Med Res. 2017;22:29. https://doi.org/10.1186/s40001-017-0271-z. 38. MacInnes SJ, Del Vescovo E, Kiss-Toth E, Ollier WE, Kay PR, Gordon A, et al. Genetic variation in inflammatory and bone turnover pathways and risk of osteolytic responses to prosthetic materials. J Orthop Res. 2015;33:193–8. https://doi.org/10.1002/jor.22755. 39. Kolundzić R, Orlić D, Trkulja V, Pavelić K, Troselj KG. Single nucleotide polymorphisms in the interleukin-6 gene promoter, tumor necrosis factor-alpha gene promoter, and transform- ing growth factor-beta1 gene signal sequence as predictors of time to onset of aseptic loosen- ing after total hip arthroplasty: preliminary study. J Orthop Sci. 2006;11:592–600. 40. Gallo J, Mrazek F, Petrek M. Variation in cytokine genes can contribute to severity of acetabu- lar osteolysis and risk for revision in patients with ABG 1 total hip arthroplasty: a genetic asso- ciation study. BMC Med Genet. 2009;10:109. https://doi.org/10.1186/1471-2350-10-109. 41. Malik MH, Jury F, Bayat A, Ollier WE, Kay PR. Genetic susceptibility to total hip arthro- plasty failure: a preliminary study on the influence of matrix metalloproteinase 1, interleukin 6 polymorphisms and vitamin D receptor. Ann Rheum Dis. 2007;66:1116–20. 42. Kremers HM, Lewallen EA, van Wijnen AJ, Lewallen DG. Clinical factors, disease param- eters, and molecular therapies affecting osseointegration of orthopedic implants. Curr Mol Biol Rep. 2016;2:123–32. https://doi.org/10.1007/s40610-016-0042-6. 1 Osteolysis After Total Hip Arthroplasty: Basic Science 23 43. Ollivere B, Wimhurst JA, Clark IM, Donell ST. Current concepts in osteolysis. J Bone Joint Surg Br. 2012;94:10–5. https://doi.org/10.1302/0301-620X.94B1.28047. 44. Ries MD, Link TM. Monitoring and risk of progression of osteolysis after total hip arthro- plasty. J Bone Joint Surg Am. 2012;94:2097–105. 45. Revell PA. The combined role of wear particles, macrophages and lymphocytes in the loos- ening of total joint prostheses. J R Soc Interface. 2008;5:1263–78. https://doi.org/10.1098/ rsif.2008.0142. 46. Kumar N, Arora GN, Datta B. Bearing surfaces in hip replacement – evolution and likely future. Med J Armed Forces India. 2014;70:371–6. https://doi.org/10.1016/j.mjafi.2014.04.015. 47. Callaghan JJ, Pedersen DR, Johnston RC, Brown TD. Clinical biomechanics of wear in total hip arthroplasty. Iowa Orthop J. 2003;23:1–12. 48. Noordin S, Masri B. Periprosthetic osteolysis: genetics, mechanisms and potential therapeu- tic interventions. Can J Surg. 2012;55:408–17. https://doi.org/10.1503/cjs.003711. 49. Sundfeldt M, Carlsson LV, Johansson CB, Thomsen P, Gretzer C. Aseptic loosening, not only a question of wear: a review of different theories. Acta Orthop. 2006;77:177–97. 50. Kapasa ER, Giannoudis PV, Jia X, Hatton PV, Yang XB. The effect of RANKL/OPG bal- ance on reducing implant complications. J Funct Biomater. 2017;8(4):E42. https://doi. org/10.3390/jfb8040042. 51. Kandahari AM, Yang X, Laroche KA, Dighe AS, Pan D, Cui Q. A review of UHMWPE wear-induced osteolysis: the role for early detection of the immune response. Bone Res. 2016;4:16014. https://doi.org/10.1038/boneres.2016.14. 52. Vallés G, Gil-Garay E, Munuera L, Vilaboa N. Modulation of the cross-talk between macro- phages and osteoblasts by titanium-based particles. Biomaterials. 2008;29:2326–35. https:// doi.org/10.1016/j.biomaterials.2008.02.011. 53. Vallés G, González-Melendi P, Saldaña L, Rodriguez M, Munuera L, Vilaboa N. Rutile and titanium particles differentially affect the production of osteoblastic local factors. J Biomed Mater Res A. 2008;84:324–36. 54. Vallés G, González-Melendi P, González-Carrasco JL, Saldaña L, Sánchez-Sabaté E, Munuera L, et al. Differential inflammatory macrophage response to rutile and titanium par- ticles. Biomaterials. 2006;27:5199–211. 55. Kobayashi A, Freeman MA, Bonfield W, Kadoya Y, Yamac T, Al-Saffar N, et al. Number of polyethylene particles and osteolysis in total joint replacements. A quantitative study using a tissue-digestion method. J Bone Joint Surg Br. 1997;79:844–8. 56. Lähdeoja T, Pajarinen J, Kouri VP, Sillat T, Salo J, Konttinen YT. Toll-like receptors and aseptic loosening of hip endoprosthesis-a potential to respond against danger signals? J Orthop Res. 2010;28:184–90. https://doi.org/10.1002/jor.20979. 57. Xing Z, Pabst MJ, Hasty KA, Smith RA. Accumulation of LPS by polyethylene particles decreases bone attachment to implants. J Orthop Res. 2006;24:959–66. 58. Alley C, Haggard W, Smith R. Effect of UHMWPE particle size, dose, and endotoxin on in vitro macrophage response. J Long-Term Eff Med Implants. 2014;24:45–56. 59. Zaveri TD, Dolgova NV, Lewis JS, Hamaker K, Clare-Salzler MJ, Keselowsky BG. Macrophage integrins modulate response to ultra-high molecular weight polyethylene particles and direct particle-induced osteolysis. Biomaterials. 2017;115:128–40. https://doi. org/10.1016/j.biomaterials.2016.10.038. 60. Gibon E, Córdova LA, Lu L, Lin TH, Yao Z, Hamadouche M, et al. The biological response to orthopedic implants for joint replacement. II: polyethylene, ceramics, PMMA, and the foreign body reaction. J Biomed Mater Res B Appl Biomater. 2017;105:1685–91. https://doi. org/10.1002/jbm.b.33676. 61. Maitra R, Clement CC, Scharf B, Crisi GM, Chitta S, Paget D, et al. Endosomal damage and TLR2 mediated inflammasome activation by alkane particles in the generation of aseptic osteolysis. Mol Immunol. 2009;47:175–84. https://doi.org/10.1016/j.molimm.2009.09.023. 62. Goodman SB, Ma T. Cellular chemotaxis induced by wear particles from joint replacements. Biomaterials. 2010;31:5045–50. https://doi.org/10.1016/j.biomaterials.2010.03.046. 24 G. Vallés and N. Vilaboa 63. Gibon E, Ma T, Ren PG, Fritton K, Biswal S, Yao Z, et al. Selective inhibition of the MCP-1- CCR2 ligand-receptor axis decreases systemic trafficking of macrophages in the presence of UHMWPE particles. J Orthop Res. 2012;30:547–53. https://doi.org/10.1002/jor.21548. 64. Liu A, Richards L, Bladen CL, Ingham E, Fisher J, Tipper JL. The biological response to nanometre-sized polymer particles. Acta Biomater. 2015;23:38–51. https://doi.org/10.1016/j. actbio.2015.05.016. 65. Kauther MD, Xu J, Wedemeyer C. Alpha-calcitonin gene-related peptide can reverse the catabolic influence of UHMWPE particles on RANKL expression in primary human osteo- blasts. Int J Biol Sci. 2010;6:525–36. 66. Atkins GJ, Welldon KJ, Holding CA, Haynes DR, Howie DW, Findlay DM. The induction of a catabolic phenotype in human primary osteoblasts and osteocytes by polyethylene particles. Biomaterials. 2009;30:3672–81. https://doi.org/10.1016/j.biomaterials.2009.03.035. 67. Chiu R, Ma T, Smith RL, Goodman SB. Ultrahigh molecular weight polyethylene wear debris inhibits osteoprogenitor proliferation and differentiation in vitro. J Biomed Mater Res A. 2009;89:242–7. https://doi.org/10.1002/jbm.a.32001. 68. Ormsby RT, Cantley M, Kogawa M, Solomon LB, Haynes DR, Findlay DM, Atkins GJ. Evidence that osteocyte perilacunar remodelling contributes to polyethylene wear particle induced osteolysis. Acta Biomater. 2016;33:242–51. https://doi.org/10.1016/j. actbio.2016.01.016. 69. Sartori M, Vincenzi F, Ravani A, Cepollaro S, Martini L, Varani K, et al. RAW 264.7 co- cultured with ultra-high molecular weight polyethylene particles spontaneously differenti- ate into osteoclasts: an in vitro model of periprosthetic osteolysis. J Biomed Mater Res A. 2017;105:510–20. https://doi.org/10.1002/jbm.a.35912. 70. Pan X, Mao X, Cheng T, Peng X, Zhang X, Liu Z, et al. Up-regulated expression of MIF by interfacial membrane fibroblasts and macrophages around aseptically loosened implants. J Surg Res. 2012;176:484–9. https://doi.org/10.1016/j.jss.2011.09.047. 71. Rao AJ, Nich C, Dhulipala LS, Gibon E, Valladares R, Zwingenberger S, et al. Local effect of IL-4 delivery on polyethylene particle induced osteolysis in the murine calvarium. J Biomed Mater Res A. 2013;101:1926–34. https://doi.org/10.1002/jbm.a.34486. 72. Du Z, Zhu Z, Wang Y. The degree of peri-implant osteolysis induced by PEEK, CoCrMo, and HXLPE wear particles: a study based on a porous Ti6Al4V implant in a rabbit model. J Orthop Surg Res. 2018;13:23. https://doi.org/10.1186/s13018-018-0736-y. 73. Vallés G, García-Cimbrelo E, Vilaboa N. Involvement of extracellular Hsp72 in wear particle-mediated osteolysis. Acta Biomater. 2012;8:1146–55. https://doi.org/10.1016/j. actbio.2011.12.001. 74. Vaculova J, Gallo J, Hurnik P, Motyka O, Goodman SB, Dvorackova J. Low intrapatient vari- ability of histomorphological findings in periprosthetic tissues from revised metal/ceramic on polyethylene joint arthroplasties. J Biomed Mater Res B Appl Biomater. 2017; https://doi. org/10.1002/jbm.b.33990. 75. Hirayama T, Tamaki Y, Takakubo Y, Iwazaki K, Sasaki K, Ogino T, et al. Toll-like receptors and their adaptors are regulated in macrophages after phagocytosis of lipopolysaccharide- coated titanium particles. J Orthop Res. 2011;29:984–92. https://doi.org/10.1002/jor.21369. 76. Dapunt U, Giese T, Lasitschka F, Reinders J, Lehner B, Kretzer JP, et al. On the inflam- matory response in metal-on-metal implants. J Transl Med. 2014;12:74. https://doi. org/10.1186/1479-5876-12-74. 77. Drynda A, Singh G, Buchhorn GH, Awiszus F, Ruetschi M, Feuerstein B, et al. Metallic wear debris may regulate CXCR4 expression in vitro and in vivo. J Biomed Mater Res A. 2015;103:1940–8. https://doi.org/10.1002/jbm.a.35330. 78. Geng D, Xu Y, Yang H, Wang J, Zhu X, Zhu G, et al. Protection against titanium parti- cle induced osteolysis by cannabinoid receptor 2 selective antagonist. Biomaterials. 2010;31:1996–2000. https://doi.org/10.1016/j.biomaterials.2009.11.069. 79. Hallab NJ, Jacobs JJ. Chemokines associated with pathologic responses to orthopedic implant debris. Front Endocrinol (Lausanne). 2017;8:5. https://doi.org/10.3389/fendo.2017.00005. 1 Osteolysis After Total Hip Arthroplasty: Basic Science 25 80. Chen M, Chen PM, Dong QR, Huang Q, She C, Xu W. p38 signaling in titanium particle- induced MMP-2 secretion and activation in differentiating MC3T3-E1 cells. J Biomed Mater Res A. 2014;102:2824–32. https://doi.org/10.1002/jbm.a.34956. 81. Qiu S, Zhao F, Tang X, Pei F, Dong H, Zhu L, et al. Type-2 cannabinoid receptor regu- lates proliferation, apoptosis, differentiation, and OPG/RANKL ratio of MC3T3-E1 cells exposed to titanium particles. Mol Cell Biochem. 2015;399:131–41. https://doi.org/10.1007/ s11010-014-2240-y. 82. Fu C, Xie J, Hu N, Liang X, Chen R, Wang C, et al. Titanium particles up-regulate the activity of matrix metalloproteinase-2 in human synovial cells. Int Orthop. 2014;38:1091–8. https:// doi.org/10.1007/s00264-013-2190-0. 83. Obando-Pereda GA, Fischer L, Stach-Machado DR. Titanium and zirconia particle-induced pro-inflammatory gene expression in cultured macrophages and osteolysis, inflamma- tory hyperalgesia and edema in vivo. Life Sci. 2014;97:96–106. https://doi.org/10.1016/j. lfs.2013.11.008. 84. Greenfield EM, Beidelschies MA, Tatro JM, Goldberg VM, Hise AG. Bacterial pathogen- associated molecular patterns stimulate biological activity of orthopaedic wear particles by activating cognate Toll-like receptors. J Biol Chem. 2010;285:32378–84. https://doi. org/10.1074/jbc.M110.136895. 85. Pajarinen J, Kouri VP, Jämsen E, Li TF, Mandelin J, Konttinen YT. The response of mac- rophages to titanium particles is determined by macrophage polarization. Acta Biomater. 2013;9:9229–40. https://doi.org/10.1016/j.actbio.2013.06.027. 86. Preedy EC, Perni S, Prokopovich P. Cobalt, titanium and PMMA bone cement debris influ- ence on mouse osteoblast cell elasticity, spring constant and calcium production activity. RSC Adv. 2015;5:83885–98. 87. Howie DW, Vernon-Roberts B. Synovial macrophage response to aluminium oxide ceramic and cobalt-chrome alloy wear particles in rats. Biomaterials. 1988;9:442–8. 88. Devitt BM, Queally JM, Vioreanu M, Butler JS, Murray D, Doran PP, et al. Cobalt ions induce chemokine secretion in a variety of systemic cell lines. Acta Orthop. 2010;81:756–64. https://doi.org/10.3109/17453674.2010.537806. 89. Jonitz-Heincke A, Tillmann J, Klinder A, Krueger S, Kretzer JP, Høl PJ, et al. The impact of metal ion exposure on the cellular behavior of human osteoblasts and PBMCs: in vitro analyses of osteolytic processes. Materials (Basel). 2017;10:E734. https://doi.org/10.3390/ma10070734. 90. Rakow A, Schoon J, Dienelt A, John T, Textor M, Duda G, Perka C, Schulze F, Ode A. Influence of particulate and dissociated metal-on-metal hip endoprosthesis wear on mesenchymal stromal cells in vivo and in vitro. Biomaterials. 2016;98:31–40. https://doi. org/10.1016/j.biomaterials.2016.04.023. 91. Potnis PA, Dutta DK, Wood SC. Toll-like receptor 4 signaling pathway mediates proinflam- matory immune response to cobalt-alloy particles. Cell Immunol. 2013;282:53–65. https:// doi.org/10.1016/j.cellimm.2013.04.003. 92. Raghunathan VK, Devey M, Hawkins S, Hails L, Davis SA, Mann S, et al. Influence of par- ticle size and reactive oxygen species on cobalt chrome nanoparticle-mediated genotoxicity. Biomaterials. 2013;34:3559–70. https://doi.org/10.1016/j.biomaterials.2013.01.085. 93. Papageorgiou I, Brown C, Schins R, Singh S, Newson R, Davis S, Fisher J, Ingham E, Case CP. The effect of nano- and micron-sized particles of cobalt-chromium alloy on human fibro- blasts in vitro. Biomaterials. 2007;28:2946–58. 94. Liu G, Guo T, Zhang Y, Liu N, Chen J, Chen J, Zhang J, Zhao J. Apoptotic pathways of macrophages within osteolytic interface membrane in periprosthestic osteolysis after total hip replacement. APMIS. 2017;125:565–78. https://doi.org/10.1111/apm.12679. 95. Lewis AC, Ladon D, Heard PJ, Peto L, Learmonth I. The role of the surface chemistry of CoCr alloy particles in the phagocytosis and DNA damage of fibroblast cells. J Biomed Mater Res A. 2007;82:363–72. 96. Athanasou NA. The pathobiology and pathology of aseptic implant failure. Bone Joint Res. 2016;5:162–8. https://doi.org/10.1302/2046-3758.55.BJR-2016-0086. 26 G. Vallés and N. Vilaboa 97. Daniel J, Holland J, Quigley L, Sprague S, Bhandari M. Pseudotumors associated with total hip arthroplasty. J Bone Joint Surg Am. 2012;94:86–93. https://doi.org/10.2106/ JBJS.J.01612. 98. Catelas I, Lehoux EA, Ning Z, Figeys D, Baskey SJ, Beaulé PE. Differential proteomic anal- ysis of synovial fluid from hip arthroplasty patients with a pseudotumor vs. periprosthetic osteolysis. J Orthop Res. 2018; https://doi.org/10.1002/jor.23858. 99. McCarthy CL, Uchihara Y, Vlychou M, Grammatopoulos G, Athanasou NA. Development of malignant lymphoma after metal-on-metal hip replacement: a case report and review of the literature. Skelet Radiol. 2017;46:831–6. https://doi.org/10.1007/s00256-017-2612-y. 100. Gallo J, Goodman SB, Lostak J, Janout M. Advantages and disadvantages of ceramic on ceramic total hip arthroplasty: a review. Biomed Pap Med Fac Univ Palacky Olomouc Czech Repub. 2012;156:204–12. https://doi.org/10.5507/bp.2012.063. 101. Mehmood S, Jinnah RH, Pandit H. Review on ceramic-on-ceramic total hip arthroplasty. J Surg Orthop Adv. 2008;17:45–50. 102. Tateiwa T, Clarke IC, Williams PA, Garino J, Manaka M, Shishido T, Yamamoto K, Imakiire A. Ceramic total hip arthroplasty in the United States: safety and risk issues revisited. Am J Orthop (Belle Mead NJ). 2008;37:E26–31. 103. Howard DP, Wall PDH, Fernandez MA, Parsons H, Howard PW.