Structural Chromosome Abnormalities PDF
Document Details
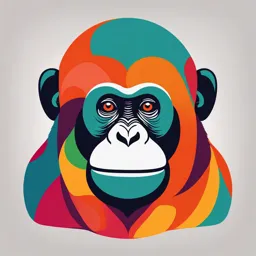
Uploaded by OrderlyTuba9689
Tags
Summary
This document discusses structural abnormalities in chromosomes, including inversions, insertions, and balanced translocations. It also covers aneuploidy, Robertsonian translocations, and their implications. The text provides a detailed explanation of the mechanisms, clinical consequences, and importance of these genetic variations.
Full Transcript
**L2: Structural abnormalities** **Balanced gross structural chromosome rearrangements: Inversion** Inversions require two breakpoints in the same chromosome, can be located either side of the centromere (pericentric), or both on the same arm (paracentric - more difficult to spot by karyotype anal...
**L2: Structural abnormalities** **Balanced gross structural chromosome rearrangements: Inversion** Inversions require two breakpoints in the same chromosome, can be located either side of the centromere (pericentric), or both on the same arm (paracentric - more difficult to spot by karyotype analysis because they don't alter morphology of the the chromosome). **Insertion:** Two breakpoints in the same chromosome and one in another - particularly rare, because more events that need to take place at the same time in the same space. Inversions and insertions form abnormal loop structures in prophase and metaphase 1, these loops can be sufficient to disrupt spermatogenesis. If a crossover occurs in the inverted segment, unbalanced gametes will form - most likely leading to a spontaneous abortion or serious health complications. Carriers of structural rearrangements are still able to have viable offspring, normal or carriers. Abnormal clinical manifestations related to gametogenesis and risk of chromosomally unbalanced conceptions. ### **Balanced Translocations** More common in the population than inversions and insertions. Arise via illegitimate recombination, though unlike aneuploidy (attributed largely to maternal meiotic errors), they mainly form during spermatogenesis. A derivative chromosome is the term used to describe a chromosome that has been generated (derived) from abnormal events. **Pachytene cross:** homologous chromosomes attempt to align despite structural rearrangement. MI Alternate segregation: Production of 4 viable gametes. MI Adjacent I segregation: 4 unbalanced gametes (longest synaptic axis) MI adjacent II segregation: 4 unbalanced gametes (short axis) 3:1 malsegregation: gametes 3 & 4 = monosomy (abort before pregnancy clinically recognised), gametes 1 & 2 = partial trisomy - possible but unlikely for pregnancy to come to term, and if it did the baby would be seriously affected. 1. - - - - - - - - - - - 2. - - - - ### **Aneuploidy and Its Implications** Aneuploidy is one of the leading causes of pregnancy loss in humans (abnormal complement of chromosomes) 1. - - - - 2. - - ### **Robertsonian Translocations** 1. - - 2. - - 3. - - 4. - - - - - - 5. - - - ### **Mal-Segregation in Robertsonian Translocations** 1. - - - - - - 2. - - ### **Aneuploid Rescue** 1. - - - - 2. - - - - - ### **Imprinted Chromosomes and Related Disorders** 1. - - 2. - - - 3. - This lecture will focus on gross structural abnormalities that are carried in the population, which predispose to chromosome mal segregation in meiosis. This is because they are thought to disturb the normal pairing of chromosomes by changing the number and position of crossovers. These factors thus increase the risk of abnormal gamete formation, that can lead to pregnancy loss, stillbirth and genetic disease. There is an assumption that no genetic material is gained or lost in a balanced event. This is not the case for unbalanced rearrangements. Robertsonian translocations -- considered at the end of this lecture are an unusual rearrangement, that "behave" like balanced rearrangements, but they are in fact unbalanced, resulting in large deletions of the genome.... Balanced rearrangements largely go undetected until adulthood, as they are usually identified following investigations for reduced fertility or recurrent miscarriage. Gross structural abnormalities in the genome are thought to be mediated by recombination events, between homologous sequences in an illegitimate/non-allelic manner. Under normal circumstances sites of DNA damage are repaired by using homologous sequences located in equivalent positions in the sister chromatid (during mitosis) and the homolog (in meiosis). Illegitimate recombination is used to describe repair events where the template and repair sites are located at non-allelic genomic positions. For example, if a site of damage in the P arm of a chromosome is repaired using homologous template sequences in the q arm this would be an example of illegitimate repair. INVERSIONS: There are 2 different types of inversion, those that include the centromere, and those that don't. If the two inversion break sites are located on different arms of the same chromosome respectively, so that the fragment of DNA which is flanked by these regions includes the centromere, then the inversion is described as pericentric (example shown in the slide). Other than a change in the banding structure, one of the most obvious features of pericentric inversions is a change to the morphology of the chromosome -- shown here and this is mainly due to the asymmetrical positioning of inversion "breakage points" with respect to the centromere. The examples shown in the slide is a pericentric inversion of chromosome 9. The abnormal chromosome is shown on the right, the normal 9 is on the left. The blue lines indicate the flanking boundaries of the inverted region. Look how the position of the centromere shifts towards the middle of the chromosome for the inverted 9. The pale band in the q arm has shifted to the p arm and now has an inverted orientation. Another example (far right), is a bigger pericentric inversion affecting chromosome 9. The breakpoints (blue lines) are shown on the "normal 9" (second from right). Again, you can see that the p arm is much larger in the inverted 9 in comparison to the normal 9. The band in the p arm of the normal 9 (purple arrow) has moved to the q arm of the inverted chromosome. The band shown by the black arrow sits outside of the inverted segment and has therefore not changed position. Both examples shown are from real patients. Inversions of chromosome 9 are the most common inversions in humans, and carriers are often not identified until adulthood. The main reason for the "late" detection of such abnormalities is because the invasions tend not to disrupt gene function or expression (as the proximal regions near to 9 centromere tend to be heterochromatin/not expressed), so the patient is essentially "normal".. Inversions do create abnormal chromosome structures during meiosis and these can be problematic and disruptive to spermatogenesis (resulting in male infertility or reduced fertility), and can increase the risk of unbalanced gamete formation if gametogenesis is permissible (more on this shortly). If the chromosome breakpoints do not flank the centromere, then the inversion is said to be paracentric. These are often more difficult to spot by karyotype analysis as they do not alter the morphology of the chromosome just banding structure. The example shown in the slide is from a patient referred for fertility investigations. This band marked with the red arrow is normally positioned proximal to the centromere, has been moved to a more distal locus in the abnormal chromosome (shown on the right). Insertions are far rarer. This is because there are more events that need to take place at the same time in the same space (thus lower probability) than for inversions. Sadly insertions are so rare that I do not have a clinical example to show you. The vast majority of insertions detected clinically are sub-microscopic/cryptic. Carriers of structural rearrangements have 1 thing in common -- and that is that when homologous chromosomes are paired in prophase and metaphase I -- the pairing structures formed by rearranged chromosomes are abnormal. Spermatogenesis is highly susceptible to these abnormal structures in MI, and so male carriers of large rearrangements are often infertile as they are unable to form mature spermatids. This often results in a low sperm count (oligospermia), or a lack of sperm in the semen (azoospermia). Oogenesis is far more robust and is more permissive of these abnormal structures. It's worth bearing this in mind when we consider the nature of the abnormal pairing structures, and the consequences for chromosomally balanced gamete production. Inversions and insertions will form abnormal loop structures in prophase and metaphase I. As I mentioned before these loops can be sufficient to disrupt spermatogenesis. However unbalanced gametes are possible and are inevitable when a crossover event occurs within the loop. The loop shown in the slide is the kind of structure expected for a paracentric inversion. But similar structures are also formed in during meiosis for carriers of pericentric inversions. Insertions are a little more complex -- but the underlying principle is the same. If a crossover occurs within the inverted segment -- then unbalanced gametes will form. In the example shown, the crossover generates an centromeric fragment, that would be lost resulting in a large imbalance that would be inviable. (full animation available here: https://www.youtube.com/watch?v=TidOfMM6SuM) The crossover would also generate a highly unstable dicentric-chromatid, that would most likely form an anaphase bridge in MI, resulting in this chromatid fragmenting under the tensional stresses imposed by the spindle -- and resulting in unbalanced gamete formation. The take home message in this slide is that inversions and insertions generate abnormal looped structures in meiosis I -- and these can be sufficient to disrupt spermatogenesis. If a crossover occurs within the inverted region then unbalanced gametes may form, that'll lead to karyotypically abnormal conceptions, that'll most likely spontaneously abort or lead to serious health complications in any new born. For this module you need to be aware that carriers of structural rearrangements are still able to have viable offspring, that are either karyotypically normal or a carrier of the structural abnormality. The only abnormal clinical manifestations are expected to be related to gametogenesis and the risk of chromosomally unbalanced conceptions. If an apparently balanced inversion or insertion was observed prenatally, in a new born, or infant and the normal development of that foetus or child was in question -- then further investigations would need to be conducted to exclude the possibility that the rearrangement was linked to the abnormal clinical phenotype i.e. via a change to the expression or function of genes in the vicinity of the breakpoints. Logically the first thing to check is whether the abnormality occurred de-novo -- or if it was inherited. If inherited from a parent who is apparently normal -- then the assumption is that the inversion does not disrupt gene function and is therefore thought to be benign. Balanced translocations are thought to be rather more common in the population than inversions and insertions -- roughly 1 in 1,000 are carriers. They are thought to arise via illegitimate recombination, though unlike the origins of aneuploidy, which are largely attributed to maternal meiotic errors, translocations are thought to mainly form during spermatogenesis. There are 2 categories of balanced translocation in the population: 1, Unique translocations/familial -- which are so rare they they only re-occur in individuals of the same family via inheritance of the derivative chromosomes (considered now). 2: Recurrent translocations (considered next). This slide shows the balanced exchange of material between chromosomes 11 and 14. The materials for this slide were kindly made available by the Sheffield Children's Genomics facility via the JGC. The patient in question was male and referred for genomic investigations following the observation of low sperm count in the semen (oligospermia). Chromosomes right to left = Normal 11, derivative 11, derivative 14, normal 14. A derivative chromosome is the term used to describe a chromosome that has been generated (derived) from abnormal events. The identity of a chromosome is determined by the origin of the centromeric region. If you look carefully, you should be able to see that the breakpoint in the 14 is very close to the telomere of the long arm, as the banding structure between the 2 14s is the same for almost the entire length of the chromosome. The break in the 11 is very close to the centromere. This translocation would be expected to generate abnormal structures during MI, and these would likely be disruptive to spermatogenesis. Therefore, this finding could explain male oligospermia. Finding such as this could also be identified in several other clinical scenarios. For example, Azospermia (absence of sperm in the semen), recurrent miscarriage (3 or more spontaneous loss of pregnancy) or failure to establish a clinically recognisable pregnancy. To make it easier to visualise -- I have artificially coloured material from chromosome 11 in red, material derived from 14 is in blue. In MI, all chromosome regions must pair. For balanced translocations, it is not possible to pair all regions with a standard bivalent. Instead -- chromosomes pair up to form a pachytene cross. You'll have to excuse the rather crude nature of this figure, but I hope it helps you to visualise the structure -- and how it offers a solution to the cell to synapse all regions. The pachytene cross is susceptible to errors in chromosome segregation -- and we'll consider this now.. Chromosomes of pachytene crosses segregate in a variety of ways. The first type of segregation is called alternate segregation. This results in the production of 4 viable gametes, each with a full and balanced haploid complement of chromosomes. The following animation will walk you through the steps. At anaphase of meiosis I, alternate centromeres are segregated to the same pole of the cell Sister chromatids are then segregated at anaphase in meiosis II into 4 gametes This is a crucially important segregation pattern as it shows that a carrier of a translocation is able to generate a viable balanced pregnancy. Fusion of gametes 1 or 2 with another normal gamete would yield a chromosomally normal conceptus. Gametes 3 or 4 would generate a viable and chromosomally balanced conceptus - the foetus would carry the translocation in these cases. In all likelihood the foetus would develop normally, but would perhaps suffer reduced fertility later in life, particularly if male. Adjacent I segregation of the pachytene cross generates 4 unbalanced gametes, as adjacent centromeres are co-segregated.. The results of this segregation pattern are shown in the slide. Hopefully this makes it clear how abnormal genetically unbalanced pregnancies are possible and more likely for carriers of large structural rearrangements. Consider the consequences of either gamete 1 or gamete 2 fusing with a second normal gamete that has a full-haploid chromosome complement. The resulting conception would have a partial trisomy for the distal part of 14q (a small region close to the telomere). Remember chromosome 14 material is in blue. The zygote would also have a partial monosomy for chromosome 11 (shown in red) -- affecting almost the entire long arm. Such huge imbalance would almost certainly result in spontaneous abortion of a pregnancy. When gametes 3 or 4 fuse with a normal gamete -- the resulting conception has a large partial trisomy for most of the long arm of chromosome 11, and a partial monosomy for the very end of chromosome 14. Again -- this would almost certainly result in an inviable pregnancy. Adjacent II segregation of the pachytene cross also generates 4 unbalanced gametes. In the previous slide (adjacent I) -- adjacent centromere segregate either side of the longest synaptic axis. In adjacent II, centromere either side of the short axis co-segregate. The imbalances generated are slightly different but the impact is very similar, a viable pregnancy would not be expected. However there is a segregation pattern that we have not yet covered, that could generate a relatively small imbalance. Such imbalances are more likely to result in a pregnancy that would be viable for longer into the gestational period, and could even come to term -- and if this happened, the new born would almost certainly be affected by profound intellectual disability as well as other physical and physiological abnormalities. The type of segregation is called 3:1 malsegregation -- shown on the following slide 3:1 mal segregation errors are more likely when there is an additional long axis for the cell to choose from -- as shown here. The gametes that are generated are interesting. Gametes 3 and 4 result in such a massive genomic imbalance that the pregnancy would not be viable -- in fact it would probably abort before the pregnancy is clinically recognised. Gametes 1 and 2 result in a conception that is not affected by monosomy (which is highly significant). There is a partial trisomy for the small arm of chromosome 11 and the very distal part of 14q. Now it is still likely that a pregnancy affected by such an imbalance would abort -- however due to the lack of a monosomy and the relatively small nature of the partial trisomy -- it's quite possible for the pregnancy to come to term -- and if it did, the the baby would be affected by serious conditions and morphological features. I mentioned a few slides ago that there are 2 types of balanced translocations. We have just considered an example of a familial translocation -- which is a rearrangement in the genome that is so rare that it's only really detected in members of the same family. The second group of translocations are considered to be recurrent. In other words, the derivative chromosomes are observed in the general population -- in unrelated families. This is because there are known non-allelic homologous repeat sequences that are brought together in the genome at defined times -- and these are particularly vulnerable to illegitimate recombination events. Perhaps the best example of this is the recurrent translocation that is observed between chromosomes 11 and 22. I have provided some reading on this translocation for you. If you engage with this reading you'll learn that this translocation is particularly vulnerable to 3:1 mal segregation, and gametes formed in this way are actually far more likely to result in a viable pregnancy and if this comes to term -- will be affected by Emanuel syndrome (Kurahashi et al 2010). It's well worth reading on this as the paper may provide you with opportunities to demonstrate further reading in the exam. It is estimated that roughly 15% of clinically recognised pregnancies miscarry. Although different studies generate slightly different figures, it's clear that somewhere between 30 and 50% of all these miscarriages have an abnormal complement of chromosomes -- meaning that aneuploidy is one of the leading causes of pregnancy loss in humans. The aneuploid conceptions that survive result in developmental and intellectual disability. Aneuploidy is therefore considered to be the leading cause of these clinical problems in the population. The Robertsonian translocations - are a special type of unbalanced rearrangement that predispose to chromosome malsegregation, resulting in common aneupoidy disorders such as Down syndrome, Patau syndrome, and (as we'll see later on) imprinting disorders! The Robertsonian translocations are the most common genetic rearrangement in humans -- present in around 1/1000 individuals. They occur between any two acrocentric chromosomes, and result in the formation of a dicentric chromosome, though only one centromere is thought to be active. The rearrangement fuses 2 acrocentric long arms together. They are considered to be unbalanced as a small acentric fragment consisting of satellited p arm material is lost. There are no know adverse clinical consequences to loss of acrocentric p arm material. This is mainly thought to be because these satellited chromosomal regions are mainly composed or repetitive elements and rDNA genes -- that are present in the genome in such high copy numbers - that the losses have negligible impact on cellular function. Robertsonian translocations are either homologous (i.e. the two fused long arms are derived from homologous chromosomes) or non-homologous -- which are more common. The most common Robertsonian translocations include the t(13;14) fusion, which accounts for roughly three quarters of them, and the t(14;21) which accounts for around 10% - shown on the following slide With thanks to the Sheffield Children's and JGC for patient materials. 14;21 Rob shown in this slide was harvested from a PND case, suspected of being affected by Down Syndrome (and later proven to be). The foetus had inherited 2 normal copies of Ch21 and an additional copy via the Rob. The abnormal chromosomal imbalance is not shown in the slide, but the derivative 14;21 Robertsonian chromosome from the patient is shown (right). Normal copies of chromosomes 14 (left) and 21 (inverted -- middle) are also shown for comparison. I mentioned before that it's thought the underlying mechanism behind robertsonian formation involves illegitimate recombination between satellites on the p arms. But this is only part of the story as this only provides an explanation for their existence -- it does not explain the prevalence of these abnormal chromosomes. It's thought that the rDNA genes are involved in this side of the story -- as acrocentric p arms come together at defined points in the cell cycle forming the nucleolus (Telophase into interphase, disassembly leading into mitosis), to bring the rDNA genes together and enable efficient expression. Thus the prevalence of these derivative chromosomes is thought to be driven by 2 factors 1. 2. I have made some reading available to you (Page et al 1996) that'll explore this fascinating topic in more detail -- please take the time to read this material. Just like for the balanced translocations -- abnormal synaptic structures form in meiosis I, that predispose to mal-segregation and the formation of unbalanced gametes that can result in inviable pregnancy or genetic disease. The 14;21 robertsonian, mal segregation events may lead to gametes that are disomic for chromosome 21. If such a gamete fuses with a chromosomally normal gamete -- then the conception will be affected by trisomy for 21. There'd be roughly an 80% chance of the pregnancy spontaneously aborting (a 20% chance of it coming to term).. and if this were to happen, the child would be affected by down syndrome. This is what has likely occurred for the patient whose 14;21 Rb has been shown in the slides. Other imbalances that are caused by mal segregation events would include monosomy of 21, monosomy of 14 and trisomy for chromosome 14 -- all of which result in inviable pregnancies. The most common robertsonian translocations (which fuse q arms belonging to 13 and 14) -- would of course elevate the risk of pregnancies being affect with Patau syndrome. There are two important concepts that we need to cover now so that you have a fuller understanding of the consequences of aneuploidy and related genetic diseases. The following slides briefly cover these topics, and "further reading" for the exam has also been provided. 1. 2. Throughout my lectures I have referred to pregnancies that are affected by large genomic imbalances as "inviable". And this is true in almost all cases -- however there is an exception to this rule. Sometimes, the very errors that drive the formation of aneuploid gamete formation, i.e. chromosome non-disjunction -- can rescue an imbalance. Lets consider the example above. A female patient has been found to be a carrier of a 14;15 robertsonian translocation. The three way pairing structure is liable to 2:1 mal segregation, and this can lead to trisomy of 14 or 15 -- both are inviable but........ Consider the "made up" gamete in the bottom right of the slide (red chromosomes). This gamete is unbalanced due to disomy for chromosome 15 (abnormal), there is also 1 copy of chromosome 14 in the gamete, and 1 copy of each of the other chromosomes (not shown to keep things simple). It is possible for this gamete to fuse with another to create a "balanced" zygote (below) 1. Or a balanced foetus.... 2. 3. 4. Walk through example: Post conceptus mitotic NDJ: After fertilisation, a zygote forms that has 2 copies of chromosome 14 (one copy in the robertsonian chromosome, the other from the male gamete) and three copies of chromosome 15 (2 from mum, and 1 from dad). A mitotic non-disjunction event has occurred removing the paternal chromosome 15, resulting in a major cell line being established soon after fertilization, which his disomic for both 14 and 15. But -- both copies of chromosome 15 are derived from the mother! This is a problem because chromosome 15 is an imprinted chromosome. This means that some genes are silenced (via methylation) on the maternally inherited Ch15. An opposite pattern of methylation (silenced expression) is expected on the paternally inherited chromosome. Thus appropriate levels of expression (gene dosage) is only established in a developing foetus if two copies of Ch15 have been inherited, and each has been inherited from either parent. Inheritance of 2 maternal CH15 and no paternal Ch15s results in over expression of some CH15 genes, and no expression of others. This results in Prader-Willi Syndrome. Inheritance of 2 Paternal CH15 and no Maternal copies results in the opposite gene expression scenario and Angelman Syndrome. Other imprinted chromosomes are listed. Note in the slide Ch16! This is the most common human trisomy. CH16 NDJ events can lead to confined placental mosaicism -- as mentioned previously.