Module Basic Medical Science Biochemistry And Nutrition PDF
Document Details
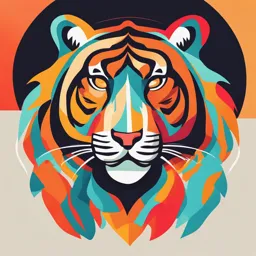
Uploaded by DazzledTelescope
UniKL RCMP
Tags
Summary
This document provides an overview of basic medical science topics, specifically biochemistry and nutrition, focusing on energy metabolism. It includes detailed information on insulin, glucagon, and other related topics. Learning outcomes are also specified.
Full Transcript
MODULE BASIC MEDICAL SCIENCE BIOCHEMISTRY AND NUTRITION RNB11903 THE BRAIN AND ENERGY METABOLISM THE BRAIN AND ENERGY METABOLISM* GLUCAGON AND INSULIN CREATINE PHOSPHATE CREATININE GLYCOGEN GLUCONEOGENESIS FRUCTOSE FATTY ACIDS THE KREBS CYCLES* FERMENTATIVE AN...
MODULE BASIC MEDICAL SCIENCE BIOCHEMISTRY AND NUTRITION RNB11903 THE BRAIN AND ENERGY METABOLISM THE BRAIN AND ENERGY METABOLISM* GLUCAGON AND INSULIN CREATINE PHOSPHATE CREATININE GLYCOGEN GLUCONEOGENESIS FRUCTOSE FATTY ACIDS THE KREBS CYCLES* FERMENTATIVE AND AEROBIC METABOLISM* *Doctor’s lessons LEARNING OUTCOMES: At end of the session studtend will able to Apply knowledge of glucose metabolism to evaluate the brain's energy demands during different cognitive tasks. explain the roles of glucagon and insulin in energy regulation and evaluate their effects on metabolic pathways under varying physiological conditions. Desribe the mechanism of ATP regeneration using creatine phosphate and assess its importance during high-intensity physical activities. Interpret creatinine levels as biomarkers for kidney function and synthesize information about its relationship with muscle metabolism. explain concepts of glycogen metabolism to real-world scenarios, such as athletic performance, and evaluate factors influencing glycogen synthesis and breakdown. Analyze the regulatory mechanisms of gluconeogenesis and create a model to illustrate its significance in maintaining glucose homeostasis during fasting. Evaluate the metabolic pathways of fructose and design an experiment to assess its effects on energy metabolism and health outcomes. Apply knowledge of fatty acid oxidation to predict energy yield during prolonged exercise and analyze the impact of different fatty acids on metabolism. Analyze the Krebs cycle's steps to synthesize a comprehensive overview of its role in energy production and metabolic intermediates Compare fermentative and aerobic metabolism, assess their efficiencies, and create a diagram illustrating their pathways and applications in different physiological contexts. GLUCAGON & INSULIN INTRODUCTION Insulin and glucagon are hormones that help to regulate the levels of blood glucose, or sugar, in the body. Glucose, which comes from the food, moves through the bloodstream to help fuel our body. Insulin and glucagon work together to balance the blood sugar levels, keeping them in the narrow range that our body requires. LEARNING OUTCOMES On successful completion of the lesson, the student will be able to: Describe the biological actions of insulin and glucagon; List the major target organs for insulin; Identify the effect of insulin on the target organs; Describe the control of glucagon secretion; Identify the target organ for glucagon. BIOLOGICAL ACTIONS OF INSULIN AND GLUCAGON 1. Insulin: A Peptide Hormone Produced By The Pancreas (Beta Cells). It Lowers Blood Glucose Levels By Facilitating Cellular Uptake Of Glucose. Glucagon: A Peptide Hormone Produced By The Pancreas (Alpha Cells). It Raises Blood Glucose Levels By Promoting Gluconeogenesis And Glycogenolysis In The Liver. DESCRIPTION ~ INSULIN The endocrine cells of the pancreas are located in islets. The islets contain four distinct cell types, each secreting a different peptide hormone. Approximately 75% of the islet cells are beta (β) cells which produce insulin. Another 20% are alpha (α) cells that secrete glucagon. The delta (δ) cells produce the paracrine, somatostatin (SRIF). Somatostatin (Also Known As Growth Hormone-inhibiting Hormone (GHIH) Or Somatotropin Release-inhibiting Factor (SRIF). Which inhibits both insulin and glucagon secretion. DESCRIPTION ~ INSULIN (CONT.) Insulin is secreted from the pancreas by a process called glucose stimulated insulin secretion (GSIS) Glucose-induced insulin secretion (GSIS) is a physiological process by which the pancreas releases insulin in response to an increase in blood glucose levels. When glucose enters the bloodstream after a meal, it is taken up by β-cells in the pancreas through glucose transporters, primarily GLUT2 (3) Mechanism of insulin secretion from beta cells of pancreas PRODUCTION OF INSULIN Site Of Production: Insulin Is Synthesized In The Beta Cells Of The Islets Of Langerhans In The Pancreas. Synthesis Process: Preproinsulin Formation: Initially, The Insulin Precursor, Preproinsulin, Is Synthesized As A Single- chain Polypeptide In The Rough Endoplasmic Reticulum (RER). Conversion To Proinsulin: Preproinsulin Undergoes Proteolytic Cleavage To Remove The Signal Peptide, Resulting In Proinsulin, Which Consists Of Three Segments: A Chain, B Chain, And Connecting Peptide (C-peptide). Maturation To Insulin: Proinsulin Is Then Transported To The Golgi Apparatus, Where It Is Packaged Into Secretory Granules. Inside These Granules, Proinsulin Is Further Cleaved By Prohormone Convertases Into Insulin (A And B Chains) And C-peptide. Secretion: Upon Stimulation (E.G., High Blood Glucose), The Granules Fuse With The Plasma Membrane, Releasing Insulin Into The Bloodstream. MAJOR TARGET ORGANS FOR INSULIN Liver: stores glucose as glycogen. Muscle: increases glucose uptake for energy. Adipose tissue: promotes fat storage and inhibits lipolysis. DESCRIPTION ~ INSULIN (CONT.) Effects of insulin The primary targets for insulin are liver, skeletal muscle and fat. Insulin has multiple actions in each of these tissues, the net result of which is fuel storage (glycogen or fat). Glucose enters the circulation either from the diet or from synthesis in the liver. It enters all cells via the glucose transporter (glut). To prevent glucose from leaving the cells via this transporter, the glucose is rapidly phosphorylated to glucose-6-phosphate. DESCRIPTION ~ INSULIN (CONT.) Effects of insulin (cont.) There is a family of glucose transporters (e.G., Glut 1, glut 2, glut 4). In skeletal muscle and fat cells, insulin binds to the insulin receptor which causes the active recruitment of the glucose transporter, glut 4, to the cell surface. Once located at the cell surface, glut 4 increases the amount of glucose that enters fat and skeletal muscle cells. The glut 4 action enables a rapid removal of glucose from the circulation thereby restoring plasma levels to 4.4-5.5 mmol/L (80-100 mg/dl). DESCRIPTION ~ INSULIN (CONT.) Insulin deficiency Problems arise from: ❖Reduced glucose uptake into various tissues (energy starvation) ❖Increased release of glucose from the liver (hyperglycemia) The effect of these two deficiencies is simple: ❖Too little glucose inside cells ❖Too much glucose in the blood (hyperglycemia) DESCRIPTION ~ INSULIN (CONT.) Positive and negative regulation of secretion by the pancreatic beta cell. EFFECTS OF INSULIN ON TARGET ORGANS Liver: Glycogenesis: insulin promotes the conversion of glucose (C₆H₁₂O₆) to glycogen (C₂₄H₄₃O₂₄) by activating glycogen synthase. Inhibition of gluconeogenesis: insulin decreases the activity of key enzymes like phosphoenolpyruvate carboxykinase (pepck). Muscle: Glucose uptake: insulin stimulates translocation of GLUT4 transporters to the cell membrane, increasing glucose uptake. Protein synthesis: promotes amino acid uptake and decreases protein breakdown by inhibiting proteolytic enzymes. Adipose tissue: Lipogenesis: insulin encourages conversion of glucose to fatty acids (C₁₆H₃₃O₂) via pathways like the pentose phosphate pathway. Inhibition of lipolysis: insulin suppresses hormone-sensitive lipase activity, reducing fatty acid release. DESCRIPTION ~ GLUCAGON Glucagon is a peptide hormone secreted from the pancreatic islet alpha cells when glucose levels are less than 4.4 mmol/L or 80 mg/dl. Glucagon circulates unbound in the plasma; it has a half- life of 6 minutes. Glucagon is a peptide hormone. PRODUCTION OF GLUCAGON Site Of Production: Glucagon Is Synthesized In The Alpha Cells Of The Islets Of Langerhans In The Pancreas. Synthesis Process: Preproglucagon Formation: The Precursor Preproglucagon Is Synthesized In The Alpha Cells. This Protein Contains Multiple Peptide Sequences, Including Those For Glucagon, GLP-1, And GLP-2. Conversion To Proglucagon: Preproglucagon Undergoes Post-translational Modifications In The Rer To Become Proglucagon. Cleavage To Glucagon: Proglucagon Is Cleaved By Prohormone Convertases Into Glucagon And Other Peptides (Like Glp-1 And Glp-2) In The Secretory Granules. Secretion: Glucagon Is Secreted Into The Bloodstream In Response To Low Blood Glucose Levels Or Stimulation By Amino Acids. DESCRIPTION ~ GLUCAGON (CONT.) Glucagon binds a plasma membrane receptor which initiates a second messenger signaling cascade. The target tissue for glucagon is the liver. Glucagon causes the liver to secrete glucose leading to a net decrease in stored glycogen and an increase in plasma glucose. In the absence of insulin, glucagon is secreted. Glucagon acts in a synergistic manner with cortisol and epinephrine to raise blood glucose levels DESCRIPTION ~ GLUCAGON (CONT.) (Corticotropin-releasing factor) (Adrenocorticotropic hormone) (Epinephrine) CONTROL OF GLUCAGON SECRETION Stimuli for secretion: Low blood glucose levels: detected by alpha cells in the pancreas. Increased amino acids: high levels, particularly arginine and alanine, stimulate glucagon release. Sympathetic nervous system: activation increases glucagon secretion during stress. Inhibitors of secretion: High blood glucose levels: inhibits glucagon release. Elevated insulin levels: negative feedback from insulin suppresses glucagon secretion. 7. Target organ for glucagon Liver: Glycogenolysis: glucagon activates glycogen phosphorylase, promoting the breakdown of glycogen to glucose. Gluconeogenesis: stimulates key enzymes like pepck and fructose-1,6-bisphosphatase, facilitating glucose synthesis from amino acids and glycerol. CHEMISTRY INVOLVED: IONS AND MOLECULES KEY MOLECULES: INSULIN: COMPOSED OF TWO POLYPEPTIDE CHAINS LINKED BY DISULFIDE BONDS (C₆₁H₁₀₁N₁₉O₁₉S₃). GLUCAGON: A 29-AMINO-ACID PEPTIDE (C₁₄H₁₉N₃O₁₃S) THAT ACTS THROUGH A G-PROTEIN-COUPLED RECEPTOR (GLP-1 RECEPTOR). IONS INVOLVED: CALCIUM IONS (CA²⁺): INSULIN SECRETION IS TRIGGERED BY INCREASED INTRACELLULAR CALCIUM LEVELS FROM GLUCOSE METABOLISM. SODIUM IONS (NA⁺): GLUCOSE UPTAKE IN MUSCLE AND ADIPOSE TISSUES RELIES ON SODIUM-DEPENDENT GLUCOSE TRANSPORTERS. POTASSIUM IONS (K⁺): INSULIN PROMOTES POTASSIUM UPTAKE INTO CELLS, LOWERING EXTRACELLULAR POTASSIUM LEVELS. SUMMARY Endocrine pancreas produces insulin and glucagon which regulate fuel homeostasis in the fed and fasted states, respectively. Insulin is secreted primarily in response to an increased blood glucose level. Glucagon is secreted in response to decreased blood glucose level. In the fed state, insulin directs the storage of excess nutrients in the form of glycogen, triglycerides, and protein. The targets of insulin are liver, muscle, and adipose tissue. In the fasting state, glucagon directs the movement of stored nutrients into the blood. Liver is the main physiological target of glucagon. SUMMARY Insulin and glucagon are vital hormones that regulate blood glucose levels. Insulin production involves several steps starting from preproinsulin synthesis in beta cells, While glucagon production originates from preproglucagon in alpha cells. Understanding these processes and the chemistry of the involved ions and molecules is crucial for comprehending metabolic pathways, especially in clinical settings like diabetes management. KEY TERMS GLYCOGENESIS: FORMATION OF GLYCOGEN FROM GLUCOSE. GLUCONEOGENESIS: SYNTHESIS OF GLUCOSE FROM NON-CARBOHYDRATE SOURCES. LIPOGENESIS: SYNTHESIS OF FAT FROM GLUCOSE. LIPOLYSIS: BREAKDOWN OF FAT. GLP-1 RECEPTOR: A RECEPTOR THAT GLUCAGON AND RELATED PEPTIDES BIND TO, INVOLVED IN GLUCOSE HOMEOSTASIS. SUMMARY CREATINE PHOSPHATE INTRODUCTION Creatine phosphate (CP) or pcr (pcr) also known as phosphocreatine. It is a phosphorylated creatine molecule that serves as a rapidly mobilizable reserve of high-energy phosphates in skeletal muscle and the brain to recycle ADENOSINE TRIPHOSPHATE (ATP), the energy currency of the cell. LEARNING OUTCOMES On successful completion of the lesson, the student will be able to: Describe the chemistry of creatine phosphate; Identify the function of creatine phosphate. CHEMISTRY OF CREATINE PHOSPHATE MOLECULAR STRUCTURE: Creatine phosphate (also known as phosphocreatine) is a phosphorylated form of creatine. CHEMICAL FORMULA: C₄H₁₁N₃O₁₃P IT CONSISTS OF A CREATINE MOLECULE (C₄H₉N₃O₂) BOUND TO A PHOSPHATE GROUP (PO₄³⁻). SYNTHESIS: Creatine Phosphate Is Synthesized From Creatine And Atp (Adenosine Triphosphate) In A Reaction Catalyzed By The Enzyme Creatine Kinase. The reaction is as follows: STORAGE: Creatine Phosphate Is Primarily Stored In Muscle Tissue And The Brain, Functioning As An Energy Reservoir. FUNCTION OF CREATINE PHOSPHATE ENERGY SOURCE: Rapid ATP Regeneration: During Short Bursts Of High-intensity Activity (Like Sprinting Or Heavy Lifting), ATP Is Rapidly Consumed. Creatine Phosphate Donates Its Phosphate Group To ADP To Regenerate ATP Quickly: CREATINE PHOSPHATE + ADP → CREATINE + ATP This reaction provides a rapid source of energy, allowing muscles to sustain contraction for a short period. FUNCTION OF CREATINE PHOSPHATE Muscle Performance: Enhanced Exercise Capacity: By Replenishing ATP, Creatine Phosphate Allows For Increased Endurance And Performance In High- intensity, Short-duration Exercises. It Is Particularly Important In Activities Like Weightlifting, Sprinting, And Other Forms Of Anaerobic Exercise. Role In Recovery: After Exercise, Creatine Phosphate Levels Help Replenish ATP Stores In Muscle Cells, Aiding In Recovery. FUNCTIONS CLINICAL RELEVANCE Supplementation: Creatine supplements are popular among athletes and bodybuilders to enhance performance and promote muscle recovery. Pathophysiological conditions: Abnormal Creatine And Creatine Phosphate metabolism may be linked to certain muscle disorders and neurological conditions, making it a focus of research in these areas. Diagnostic marker: Elevated levels of Creatine And Creatine Phosphate in the blood can indicate muscle damage or stress. RELATED DISEASES AND CONDITIONS Muscular dystrophies: Conditions like duchenne muscular dystrophy (DMD) involve impaired muscle function and energy metabolism. Abnormal creatine metabolism may contribute to muscle degeneration. Metabolic myopathies: Disorders affecting energy production, such as mitochondrial myopathies, can lead to deficiencies in ATP and creatine phosphate levels, resulting in muscle weakness and exercise intolerance. Heart disease: Creatine kinase levels are often measured in diagnosing myocardial infarction (heart attack). Elevated creatine kinase, particularly the MB isoenzyme, can indicate heart muscle damage. Rhabdomyolysis: A condition characterized by the breakdown of muscle tissue, leading to the release of myoglobin into the bloodstream. This can cause elevated levels of creatine kinase and creatine phosphate, indicating muscle damage. Neurological conditions: Research suggests that altered creatine metabolism may be involved in neurodegenerative diseases such as alzheimer's and parkinson's disease. Creatine supplementation has been investigated for potential neuroprotective effects. SUMMARY Phosphocreatine acts as high-energy reserve in a coupled reaction. The energy given off from donating the phosphate group is used to regenerate the other compound - in this case, ATP. Phosphocreatine plays a particularly important role in tissues that have high, fluctuating energy demands such as muscle and brain. SUMMARY Creatine Phosphate Is Essential For Energy Metabolism In Muscle Cells, Facilitating Rapid ATP Regeneration During High-intensity Exercise. Its Clinical Relevance Spans Various Conditions, Including Muscular Dystrophies, Metabolic Myopathies, Heart Disease, Rhabdomyolysis, And Neurodegenerative Disorders. Understanding The Biochemical Role Of Creatine Phosphate Is Vital For Nursing Professionals In The Context Of Patient Care And Management. Key Terms Creatine: An Amino Acid Derivative Involved In Energy Metabolism. Atp (Adenosine Triphosphate): The Primary Energy Carrier In Cells. Creatine Kinase: The Enzyme Responsible For The Conversion Of Creatine And Atp To Creatine Phosphate And Adp. Rhabdomyolysis: A Syndrome Caused By Muscle Injury, Leading To The Release Of Myoglobin And Creatine Kinase Into The Bloodstream. CREATININE INTRODUCTION Creatinine (Cr) is a breakdown product or waste product of creatine phosphate in muscle that is cleared from the body through the urine. It is usually produced at a fairly constant rate by the body (depending on muscle mass). LEARNING OUTCOMES On successful completion of the lesson, the student will be able to: Describe the physiology of creatinine; Identify the diagnostic use of creatinine. PHYSIOLOGY Creatinine is produced via a biological system involving creatine, creatine phosphate and adenosine triphosphate (ATP). Creatine is synthesized primarily in the liver from the methylation of glycocyamine by s- adenosyl methionine. It is then transported through blood to the other organs, muscle, and brain, where, through phosphorylation, it becomes the high-energy compound creatine phosphate. Creatinine is a breakdown product of creatine phosphate in muscle. PHYSIOLOGY (CONT.) PHYSIOLOGY (CONT.) Creatinine is removed from the blood chiefly by the kidneys, primarily by glomerular filtration. Little or no tubular reabsorption of creatinine occurs. If the filtration in the kidney is deficient, blood creatinine concentrations rise. PHYSIOLOGY (CONT.) Therefore, creatinine concentrations in blood and urine may be used to calculate the CREATININE CLEARANCE (CRCL), which correlates approximately with the GLOMERULAR FILTRATION RATE (GFR). Blood creatinine concentrations may also be used alone to calculate the estimated GFR (EGFR). The GFR is clinically important because it is a measurement of renal function. PHYSIOLOGY (CONT.) Each day, 1% to 2% of muscle creatine is converted to creatinine. The conversion is nonenzymatic and irreversible. Men tend to have higher concentrations of creatinine than women because, in general, they have a greater mass of skeletal muscle. Increased dietary intake of creatine or eating a lot of protein (like meat) can increase daily creatinine excretion. DIAGNOSTIC USE Serum creatinine is the most commonly used indicator (but not direct measure) of renal function. Elevated creatinine is not always representative of a true reduction in gfr. A high reading may be due to increased production of creatinine not due to decreased kidney function, to interference with the assay, or to decreased tubular secretion of creatinine. DIAGNOSTIC USE (CONT.) An increase in serum creatinine can be due to increased ingestion of cooked meat (which contains creatinine converted from creatine by the heat from cooking) or excessive intake of protein and creatine supplements, taken to enhance athletic performance. Intense exercise can increase creatinine by increasing muscle breakdown. Dehydration secondary to an inflammatory process with fever may cause a false increase in creatinine concentrations not related to an actual kidney injury, as in some cases with cholecystitis. Several medications and chromogens can interfere with the assay. Creatinine secretion by the tubules can be blocked by some medications, again increasing measured creatinine. DIAGNOSTIC USE (CONT.) Urine creatinine In normal circumstances, all this daily creatinine production is excreted in the urine. Creatinine concentration is checked during standard urine drug tests. SUMMARY GLYCOGEN INTRODUCTION Glycogen is a multibranched polysaccharide of glucose that serves as a form of energy storage. The polysaccharide structure represents the main storage form of glucose in the body. Glycogen functions as one of two forms of long-term energy reserves, with the other form being triglyceride stores in adipose tissue (i.E., Body fat). LEARNING OUTCOMES On successful completion of the lesson, the student will be able to: Describe the structure of glycogen; Identify the function of glycogen; Explain the metabolism of glycogen. STRUCTURE Glycogen is a branched biopolymer consisting of linear chains of glucose residues with an average chain length of approximately 8–12 glucose units. Glucose units are linked together linearly by α(1→4) glycosidic bonds from one glucose to the next. Branches are linked to the chains from which they are branching off by α(1→6) glycosidic bonds between the first glucose of the new branch and a glucose on the stem chain. Due to the way glycogen is synthesized, every glycogen granule has at its core a glycogenin protein. STRUCTURE (CONT.) STRUCTURE (CONT.) Glycogen in muscle, liver and fat cells is stored in a hydrated form, composed of three or four parts of water per part of glycogen associated with 0.45 millimoles (18 mg) of potassium per gram of glycogen. Glucose is an osmotic molecule and can have profound effects on osmotic pressure in high concentrations possibly leading to cell damage or death if stored in the cell without being modified. Glycogen is a non-osmotic molecule, so it can be used as a solution to storing glucose in the cell without disrupting osmotic pressure. STRUCTURE (CONT.) FUNCTIONS Liver As a meal containing carbohydrates or protein is eaten and digested, blood glucose levels rise and the pancreas secretes insulin. Blood glucose from the portal vein enters liver cells (hepatocytes). Insulin acts on the hepatocytes to stimulate the action of several enzymes, including glycogen synthase. Glucose molecules are added to the chains of glycogen as long as both insulin and glucose remain plentiful. In this postprandial or "fed" state, the liver takes in more glucose from the blood than it releases. FUNCTIONS (CONT.) Liver (cont.) After a meal has been digested and glucose levels begin to fall, insulin secretion is reduced and glycogen synthesis stops. When it is needed for energy, glycogen is broken down and converted again to glucose. Glycogen phosphorylase is the primary enzyme of glycogen breakdown. For the next 8–12 hours, glucose derived from liver glycogen is the primary source of blood glucose used by the rest of the body for fuel. FUNCTIONS (CONT.) Liver (cont.) Glucagon, another hormone produced by the pancreas, serves as a countersignal to insulin. In response to insulin levels being below normal (when blood levels of glucose begin to fall below the normal range), glucagon is secreted in increasing amounts and stimulates both glycogenolysis (the breakdown of glycogen) and gluconeogenesis (the production of glucose from other sources). FUNCTIONS (CONT.) Muscle Muscle cell glycogen appears to function as an immediate reserve source of available glucose for muscle cells. As muscle cells lack GLUCOSE-6-PHOSPHATASE, which is required to pass glucose into the blood, the glycogen they store is available solely for internal use and is not shared with other cells. This is in contrast to liver cells, which, on demand, readily do break down their stored glycogen into glucose and send it through the blood stream as fuel for other organs. METABOLISM Glycogen degradation consists of three steps: 1) The release of GLUCOSE 1-PHOSPHATE from GLYCOGEN 2) The remodeling of the GLYCOGEN substrate to permit further degradation 3) The conversion of GLUCOSE 1-PHOSPHATE into GLUCOSE 6-PHOSPHATE for further metabolism The glucose 6-phosphate derived from the breakdown of glycogen has three fates: 1) It is the initial substrate for glycolysis 2) It can be processed by the pentose phosphate pathway to yield NADPH AND RIBOSE DERIVATIVES 3) It can be converted into free glucose for release into the bloodstream (this conversion takes place mainly in the liver and to a lesser extent in the intestines and kidneys.) METABOLISM (CONT.) GLUCOSE 6-PHOSPHATE derived from glycogen can 1) be used as a fuel for anaerobic or aerobic metabolism as in, for instance, muscle 2) be converted into free glucose in the liver and subsequently released into the blood 3) be processed by the PENTOSE PHOSPHATE pathway to generate NADPH OR RIBOSE in a variety of tissues. NADPH is a cofactor, used to donate electrons and a hydrogens to reactions catalyzed by some enzymes. METABOLISM (CONT.) Glycogen synthesis requires an activated form of glucose, URIDINE DIPHOSPHATE GLUCOSE (UDP-GLUCOSE), which is formed by the reaction of UTP and GLUCOSE 1- PHOSPHATE. UDP-GLUCOSE is added to the nonreducing end of glycogen molecules. As is the case for glycogen degradation, the glycogen molecule must be remodeled for continued synthesis. METABOLISM (CONT.) The regulation of these processes is quite complex. Several enzymes taking part in glycogen metabolism allosterically respond to metabolites that signal the energy needs of the cell. These allosteric responses allow the adjustment of enzyme activity to meet the needs of the cell in which the enzymes are expressed. Glycogen metabolism is also regulated by hormonally stimulated cascades that lead to the reversible phosphorylation of enzymes, which alters their kinetic properties. Regulation by hormones allows glygogen metabolism to adjust to the needs of the entire organism. By both these mechanisms, glycogen degradation is integrated with glycogen synthesis. METABOLISM (CONT.) SUMMARY Approximately 4 grams of glucose are present in the blood of humans at all times. In fasted individuals, blood glucose is maintained constant at this level at the expense of glycogen stores in the liver and skeletal muscle. Liver glycogen stores serve as a store of glucose for use throughout the body, particularly the central nervous system. Glycogen stores in skeletal muscle serve as a form of energy storage for the muscle itself; however, the breakdown of muscle glycogen impedes muscle glucose uptake, thereby increasing the amount of blood glucose available for use in other tissues.