17 Lecture Presentation: Expression of Genes PDF
Document Details
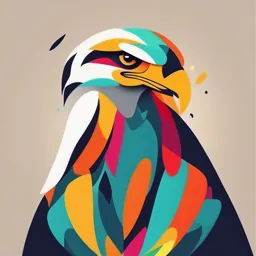
Uploaded by LivelyHexagon6175
An-Najah National University
Nicole Tunbridge and Kathleen Fitzpatrick
Tags
Summary
This lecture presentation explains gene expression, focusing on transcription and translation. It details the role of RNA in protein synthesis and introduces the genetic code. The presentation also highlights differences between prokaryotic and eukaryotic gene expression.
Full Transcript
Chapter 17 Expression of Genes Lecture Presentations by Nicole Tunbridge and © 2018 Pearson Education Ltd. Ka...
Chapter 17 Expression of Genes Lecture Presentations by Nicole Tunbridge and © 2018 Pearson Education Ltd. Kathleen Fitzpatrick The Flow of Genetic Information The information content of genes is in the specific sequences of nucleotides The DNA inherited by an organism leads to specific traits by dictating the synthesis of proteins Proteins are the links between genotype and phenotype Gene expression, the process by which DNA directs protein synthesis, includes two stages: transcription and translation © 2018 Pearson Education Ltd. © 2018 Pearson Education Ltd. Concept 17.1: Genes specify proteins via transcription and translation © 2018 Pearson Education Ltd. Basic Principles of Transcription and Translation Genes direct the synthesis of proteins, but they don’t directly synthesize them. RNA is the bridge between genes and the proteins for which they code Transcription is the synthesis of RNA using information in DNA Transcription produces messenger RNA (mRNA) Translation is the synthesis of a polypeptide, using information in the mRNA Ribosomes are the sites of translation © 2018 Pearson Education Ltd. In prokaryotes, translation of mRNA can begin before transcription has finished Why..?? Bacterial genetic DNA is in the cytoplasm, once RNA is under process, ribosomes will sense RNA and starts translation In a eukaryotic cell, the nuclear envelope separates transcription from translation Eukaryotic RNA transcripts are modified through RNA processing to yield the finished mRNA © 2018 Pearson Education Ltd. Keep in mind that there are more than one type of RNA: mRNA = protein synthesis tRNA = a.a transfer during protein synthesis rRNA= essential role within the ribosome And many others (SiRNA, snRNA…) © 2018 Pearson Education Ltd. Figure 17.4 Nuclear envelope DNA TRANSCRIPTION Pre-mRNA RNA PROCESSING NUCLEUS mRNA DNA TRANSCRIPTION CYTOPLASM CYTOPLASM mRNA TRANSLATION Ribosome Ribosome TRANSLATION Polypeptide Polypeptide (a) Bacterial cell (b) Eukaryotic cell © 2018 Pearson Education Ltd. A primary transcript is the initial RNA transcript from any gene prior to processing The central dogma is the concept that cells are governed by a cellular chain of command: DNA → RNA → protein DNA RNA Protein © 2018 Pearson Education Ltd. The Genetic Code How only 4 nucleotides forms 20 different a.a?? It is impossible that each letter will code for only one a.a , or for more than one a.a. so what is the case?? How many nucleotides correspond to an amino acid? CODONS IS THE ANSWER © 2018 Pearson Education Ltd. Codons: Triplets of Nucleotides The flow of information from gene to protein is based on a triplet code: a series of nonoverlapping, three- nucleotide words The words of a gene are transcribed into complementary nonoverlapping three-nucleotide words of mRNA These words are then translated into a chain of amino acids, forming a polypeptide © 2018 Pearson Education Ltd. Figure 17.5 DNA molecule Gene 1 Gene 2 Gene 3 DNA template 5′ 3′ strand A C C A A A C C G A G T T G G T T T G G C T C A 3′ 5′ TRANSCRIPTION mRNA U G G U U U G G C U C A 5′ 3′ Codon TRANSLATION Protein Trp Phe Gly Ser Amino acid © 2018 Pearson Education Ltd. One of the two DNA strands, the template strand, provides a template for ordering the sequence of complementary nucleotides in an RNA transcript The template strand is always the same strand for a given gene The strand used as the template is determined by the orientation of the enzyme that transcribes the gene This in turn, depends on the DNA sequences associated with the gene © 2018 Pearson Education Ltd. During translation, the mRNA base triplets, called codons, are read in the 5′ → 3′ direction The non-template strand is called the coding strand because the nucleotides of this strand are identical to the codons, except that T is present in the DNA in place of U in the RNA Each codon specifies the amino acid (one of 20) to be placed at the corresponding position along a polypeptide © 2018 Pearson Education Ltd. Cracking the Code All 64 codons were deciphered in the early 1960s Of the 64 triplets, 61 code for amino acids; 3 triplets are “stop” signals to end translation The genetic code is redundant (more than one codon may specify a particular amino acid) but not the opposite !; no codon specifies more than one amino acid Codons must be read in the correct reading frame (correct groupings) in order for the specified polypeptide to be produced © 2018 Pearson Education Ltd. Figure 17.6 Second mRNA base U C A G UUU Phe UCU UAU Tyr UGU Cys U UUC (F) UCC (Y) (C) C U Ser UAC UGC UUA Leu UCA (S) UAA Stop UGA Stop A UUG (L) UCG UAG Stop UGG Trp (W) G Third mRNA base (3′ end of codon) First mRNA base (5′ end of codon) CUU CCU CAU His CGU U CUC Leu (H) C CCC Pro CAC CGC Arg C CUA (L) CCA (P) CAA (R) A Gln CGA CUG CCG CAG (Q) CGG G AUU ACU AAU Asn AGU Ser U Ile (N) AGC (S) AUC (I) ACC Thr AAC C A (T) AAA AUA ACA Lys AGA Arg A AUG Met (M) or start ACG AAG (K) AGG (R) G GUU GCU GAU Asp GGU U GUC Val GCC Ala GAC (D) GGC Gly C G (V) (A) (G) GUA GCA GAA Glu GGA A GUG GCG GAG (E) GGG G © 2018 Pearson Education Ltd. Concept 17.2: Transcription is the DNA-directed synthesis of RNA: a closer look Transcription is the first stage of gene expression © 2018 Pearson Education Ltd. Molecular Components of Transcription RNA synthesis is catalyzed by RNA polymerase, which pries the DNA strands apart and joins together the RNA nucleotides ( no need for helicase enzyme!) The RNA is complementary to the DNA template strand RNA polymerase does not need any primer RNA synthesis follows the same base-pairing rules as DNA, except that uracil substitutes for thymine As in DNA polymerase, RNA polymerase synthesize RNA from 5´ 3´ © 2018 Pearson Education Ltd. Difference between DNA /RNA polymerase DNA Polymerase RNA Polymerase function DNA replication Transcription (RNA synthesis ) Starting point Origin of replication Promoter sequence nucleotides Adds A C G T Adds A C G U Primer Essential to be present Doesn’t need a primer Synthesis direction of 5´ to 3´ 5´ to 3´ the new strand Helicase enzyme Essential to separate 2 Not needed, RNA strands Polymerase separates 2 strands of DNA © 2014 Pearson Education, Inc. RNA transcription occur in 3 stages : 1- Initiation 2- Elongation 3- Termination © 2018 Pearson Education Ltd. Figure 17.8_1 Promoter Transcription unit 5′ 3′ 3′ 5′ Start point DNA RNA polymerase 1 Initiation Unwound DNA Nontemplate strand of DNA 5′ 3′ 3′ 5′ Template strand of DNA RNA transcript RNA polymerase will bind to the promoter sequence > RNA polymerase will separate two strands of DNA > One strand will be used as a template, other strand will be a non-template. Transcription unit : DNA stretch from promoter to terminator that forms RNA molecule © 2018 Pearson Education Ltd. Figure 17.8_2 Promoter Transcription unit 5′ 3′ 3′ 5′ Start point DNA RNA polymerase 1 Initiation Unwound DNA Nontemplate strand of DNA 5′ 3′ 3′ 5′ Template strand of DNA RNA transcript 2 Elongation Rewound 5′ DNA 3′ 3′ 3′ 5′ 5′ RNA Direction of transcript transcription (“downstream”) Transcription : Upstream Downstream © 2018 Pearson Education Ltd. Figure 17.8_3 Promoter Transcription unit 5′ 3′ 3′ 5′ Start point DNA RNA polymerase 1 Initiation Unwound DNA Nontemplate strand of DNA 5′ 3′ 3′ 5′ Template strand of DNA RNA transcript 2 Elongation Rewound 5′ DNA 3′ 3′ 3′ 5′ 5′ RNA Direction of transcript transcription 3 Termination (“downstream”) 5′ 3′ 3′ 5′ 5′ 3′ Completed RNA transcript © 2018 Pearson Education Ltd. The DNA sequence where RNA polymerase attaches is called the promoter In bacteria, the sequence signaling the end of transcription is called the terminator The stretch of DNA that is transcribed is called a transcription unit © 2018 Pearson Education Ltd. RNA Polymerase Binding and Initiation of Transcription Start point: is the sequence that RNA polymerase starts transcription. Promoter is found upstream the start point. Transcription factors mediate the binding of RNA polymerase and the initiation of transcription The completed assembly of transcription factors and RNA polymerase II bound to a promoter is called a transcription initiation complex A promoter called a TATA box is crucial in forming the initiation complex in eukaryotes © 2018 Pearson Education Ltd. Figure 17.9 Promoter Nontemplate strand DNA 5′ T A T AAAA 3′ 3′ ATAT T T T 5′ 1 A eukaryotic promoter TATA box Start point Template strand Transcription factors 5′ 3′ 2 Several 3′ 5′ transcription factors bind to DNA. RNA polymerase II Transcription factors 5′ 3′ 3′ 3 Transcription 3′ 5′ 5′ initiation RNA transcript complex forms. © 2018 Pearson Education Ltd. Elongation of the RNA Strand As RNA polymerase moves along the DNA, it untwists the double helix, 10 to 20 bases at a time Transcription progresses at a rate of 40 nucleotides per second in eukaryotes A gene can be transcribed simultaneously by several RNA polymerases Nucleotides are added to the 3′ end of the growing RNA molecule © 2018 Pearson Education Ltd. Figure 17.10 Nontemplate strand of DNA RNA nucleotides RNA polymerase C C A A T A T 3′ T U 5′ C T 3′ end G U A A G C C A C C A U C 5′ A 3′ T A A G G T T 5′ Direction of transcription Template strand of DNA Newly made RNA © 2018 Pearson Education Ltd. Termination of Transcription The mechanisms of termination are different in bacteria and eukaryotes In bacteria, the polymerase stops transcription at the end of the terminator and the mRNA can be translated without further modification In eukaryotes, RNA polymerase II transcribes the polyadenylation signal sequence (AAUAAA); the RNA transcript is released 10–35 nucleotides past this polyadenylation sequence © 2018 Pearson Education Ltd. Concept 17.3: Eukaryotic cells modify RNA after transcription Enzymes in the eukaryotic nucleus modify pre- mRNA (RNA processing) before the genetic messages are dispatched to the cytoplasm During RNA processing, both ends of the primary transcript are altered Also, in most cases, certain interior sections of the molecule are cut out and the remaining parts spliced together (Introns) © 2018 Pearson Education Ltd. Alteration of mRNA Ends Each end of a pre-mRNA molecule is modified in a particular way The 5′ end receives a modified nucleotide 5′ cap The 3′ end gets a poly-A tail (after poly-A signal AAUAAA) an enzyme adds 50-250 adenine A units These modifications share several functions They seem to facilitate the export of mRNA to the cytoplasm They protect mRNA from hydrolytic enzymes in the cytoplasm They help ribosomes attach to the 5′ end © 2018 Pearson Education Ltd. Figure 17.11 A modified guanine 50–250 adenine nucleotide added to nucleotides added the 5′ end to the 3′ end Region that includes Polyadenylation protein-coding segments signal 5′ 3′ G P P P AAUAAA AAA···AAA Start Stop 5′ Cap 5′ UTR codon codon 3′ UTR Poly-A tail © 2018 Pearson Education Ltd. Split Genes and RNA Splicing Most eukaryotic genes and their RNA transcripts have long noncoding stretches of nucleotides that lie between coding regions These noncoding regions are called intervening sequences, or introns The other regions are called exons because they are eventually expressed, usually translated into amino acid sequences RNA splicing removes introns and joins exons, creating an mRNA molecule with a continuous coding sequence © 2018 Pearson Education Ltd. Figure 17.12 Pre-mRNA 5′ Cap Intron Intron 3′ Poly-A tail 1–30 31–104 105–146 Introns cut out and exons spliced together 5′ Cap mRNA Poly-A tail 1–146 5′ UTR 3′ UTR Coding segment © 2018 Pearson Education Ltd. RNA splicing is carried out by spliceosomes Spliceosomes consist of a variety of protein complex and several small RNAs (snRNA) that recognize the splice sites (introns) The RNAs of the spliceosome also catalyze the splicing reaction © 2018 Pearson Education Ltd. Figure 17.13 Spliceosome Small RNAs 5′ Pre-mRNA Exon 1 Exon 2 Intron Spliceosome components mRNA 5′ Cut-out Exon 1 Exon 2 intron © 2018 Pearson Education Ltd. Ribozymes Ribozymes are catalytic RNA molecules that function as enzymes and can splice RNA (ex. snRNA) The discovery of ribozymes rendered obsolete the belief that all biological catalysts were proteins Not proteins/enzymes are the only catalysts, RNA can function as an enzyme also! © 2018 Pearson Education Ltd. Three properties of RNA enable it to function as an enzyme RNA is single stranded, but it can base-pair to form secondary structure and thus It can form a three- dimensional structure as in proteins Some bases in RNA contain functional groups that may participate in catalysis (the as some a.a in proteins have functional groups ) RNA may hydrogen-bond with other nucleic acid molecules (RNA & DNA) thus it promotes/catalyze the reaction/process. © 2018 Pearson Education Ltd. The Functional and Evolutionary Importance of Introns Some introns contain sequences that may regulate gene expression Some genes can encode more than one kind of polypeptide, depending on which segments are treated as exons during splicing This is called alternative RNA splicing Consequently, the number of different proteins an organism can produce is much greater than its number of genes © 2018 Pearson Education Ltd. Proteins often have a modular architecture consisting of discrete regions called domains In many cases, different exons code for the different domains in a protein Exon shuffling may result in the evolution of new proteins © 2018 Pearson Education Ltd. Figure 17.14 Gene DNA Exon 1 Intron Exon 2 Intron Exon 3 Transcription RNA processing Translation Domain 3 Domain 2 Domain 1 Polypeptide © 2018 Pearson Education Ltd. Concept 17.4: Translation is the RNA-directed synthesis of a polypeptide: a closer look Genetic information flows from mRNA to protein through the process of translation © 2018 Pearson Education Ltd. Molecular Components of Translation A cell translates an mRNA message into protein with the help of transfer RNA (tRNA) tRNAs transfer amino acids to the growing polypeptide in a ribosome tRNA are not all identical, and each type of tRNA molecule translates a particular mRNA codon into a particular amino acid Translation is a complex process in terms of its biochemistry and mechanics © 2018 Pearson Education Ltd. Figure 17.15 Amino Polypeptide acids tRNA with amino acid attached Ribosome Trp Phe Gly tRNA C C C C A G Anticodon A A A U G G U U U G G C 5′ Codons 3′ mRNA © 2018 Pearson Education Ltd. The Structure and Function of Transfer RNA Each tRNA molecule enables translation of a given mRNA codon into a certain amino acid, it has two functions: Each carries a specific amino acid on one end Each has an anticodon on the other end; the anticodon base-pairs with a complementary codon on mRNA © 2018 Pearson Education Ltd. tRNA is synthesized in the nucleus and transferred to the cytoplasm. A tRNA molecule consists of a single RNA strand that is only about 80 nucleotides long Flattened into one plane to reveal its base pairing, a tRNA molecule looks like a cloverleaf © 2018 Pearson Education Ltd. Because of hydrogen bonds, tRNA actually twists and folds into a three-dimensional molecule tRNA is roughly L-shaped with the 5' and 3' ends both located near one end of the structure The protruding 3' end acts as an attachment site for an amino acid Anti-codon region of tRNA is from 3’ to 5 ’, it is complementary to mRNA codon (5 ’ 3’ ) © 2018 Pearson Education Ltd. Figure 17.16 3′ A Amino acid C attachment C site A 5′ C G Amino acid G C attachment site C G 5′ U G U A 3′ A U U C A U * C A C AG U A G * A * C U C G * C G U G U * C G A G G Hydrogen * * C A G U *GA * G bonds G G C C Hydrogen U A bonds * G * A A C * U A A G A A G 3′ 5′ 3′ 5 ′ Anticodon Anticodon Anticodon (a) Two-dimensional (b) Three-dimensional (c) Symbol used structure structure in this book © 2018 Pearson Education Ltd. Accurate translation requires two steps First: a correct match between a tRNA and an amino acid from cytoplasm, done by the enzyme aminoacyl-tRNA synthetase Second: a correct match between the tRNA anticodon and an mRNA codon Flexible pairing at the third base of a codon is called wobble and allows some tRNAs to bind to more than one codon That’s why multiple codons code for the same amino acid © 2018 Pearson Education Ltd. If we will consider that there are 64 codons, so.. There should be 64 tRNA. However, there are only 45 tRNA molecules. Base-pairing between codon-anticodon is dependent on the first 2 nucleotides only. The third base can base pair with other nucleotides. For example U (in the third position) can base pair with A or G UCU (anti-codon) can base-pair with AGG or AGA © 2018 Pearson Education Ltd. Figure 17.17_1 Tyrosine (Tyr) 1 Amino acid (amino acid) and tRNA Tyrosyl-tRNA enter active synthetase site. Tyr-tRNA A U A Each aminoacyl tRNA Complementary synthetase combines the tRNA anticodon correct tRNA to the correct a.a. There are more than 20 different aminoacyl tRNA synthetase for 20 different a.a © 2018 Pearson Education Ltd. Figure 17.17_2 Tyrosine (Tyr) 1 Amino acid (amino acid) and tRNA Tyrosyl-tRNA enter active synthetase site. Tyr-tRNA Aminoacyl-tRNA synthetase A U A ATP Complementary AMP + 2 P i tRNA tRNA anticodon 2 Using ATP, Amino synthetase acid catalyzes covalent bonding. Computer model © 2018 Pearson Education Ltd. Figure 17.17_3 Tyrosine (Tyr) 1 Amino acid (amino acid) and tRNA Tyrosyl-tRNA enter active synthetase site. Tyr-tRNA Aminoacyl-tRNA synthetase A U A ATP Complementary AMP + 2 P i tRNA tRNA anticodon 3 Aminoacyl tRNA 2 Using ATP, Amino released. synthetase acid catalyzes covalent bonding. Computer model © 2018 Pearson Education Ltd. The Structure and Function of Ribosomes Ribosomes facilitate specific coupling of tRNA anticodons with mRNA codons in protein synthesis The two ribosomal subunits (large and small) are made of proteins and ribosomal RNA (rRNA) Bacterial and eukaryotic ribosomes are somewhat similar but have significant differences Some antibiotic drugs specifically target bacterial ribosomes without harming eukaryotic ribosomes Ex. Tetracycline antibiotic © 2018 Pearson Education Ltd. A ribosome has three binding sites for tRNA The A site holds the tRNA that carries the next amino acid to be added to the chain The P site holds the tRNA that carries the growing polypeptide chain The E site is the exit site, where discharged tRNAs leave the ribosome A ribosome has one binding sites for mRNA © 2018 Pearson Education Ltd. Figure 17.18 Growing polypeptide Exit tunnel for growing polypeptide Large subunit EP A tRNA molecules Small subunit 5′ mRNA 3′ (a) Computer model of functioning ribosome P site (peptidyl-tRNA Amino binding site) end Growing polypeptide Exit tunnel Carboxyl Next amino acid end to be added to A site (aminoacyl- polypeptide chain E site tRNA binding site) (exit site) E P A E tRNA Large mRNA 3′ subunit mRNA binding site Small Codons 5′ subunit (b) Schematic model showing binding sites (c) Schematic model with mRNA and tRNA © 2018 Pearson Education Ltd. Figure 17.18b P site (peptidyl-tRNA binding site) Exit tunnel A site (aminoacyl- E site tRNA binding site) (exit site) E P A Large subunit mRNA binding site Small subunit (b) Schematic model showing binding sites © 2018 Pearson Education Ltd. Figure 17.18c Amino end Growing polypeptide Carboxyl end Next amino acid to be added to polypeptide chain E tRNA mRNA 3′ 5′ Codons (c) Schematic model with mRNA and tRNA © 2018 Pearson Education Ltd. Building a Polypeptide The three stages of translation: Initiation Elongation Termination All three stages require protein “factors” that aid in the translation process Energy is required for some steps, too © 2018 Pearson Education Ltd. Ribosome Association and Initiation of Translation The start codon (AUG) signals the start of translation First, a small ribosomal subunit binds with mRNA and a special initiator tRNA Then the small subunit moves along the mRNA until it reaches the start codon Proteins called initiation factors bring in the large subunit that completes the translation initiation complex © 2018 Pearson Education Ltd. Figure 17.19 3′ U A C 5′ Large P site Met 5′ A U G 3′ ribosomal Met subunit Pi + Initiator tRNA GTP GDP E A mRNA 5′ 5′ 3′ 3′ Start codon Small ribosomal mRNA binding site subunit Translation initiation complex 1 Small ribosomal subunit binds 2 Large ribosomal subunit to mRNA. completes the initiation complex. © 2018 Pearson Education Ltd. Elongation of the Polypeptide Chain During elongation, amino acids are added one by one to the C-terminus of the growing chain Each addition involves proteins called elongation factors Elongation occurs in three steps: codon recognition, peptide bond formation, and translocation Energy expenditure occurs in the first and third steps For accurate and efficient codon binding © 2018 Pearson Education Ltd. Translation proceeds along the mRNA in a 5′ → 3′ direction and The ribosome and mRNA synthesizes a polypeptide chain from N-terminus to C-terminus rRNA catalyze the formation of peptide bond between a.a move relative to each other, codon by codon The elongation cycles takes less than a tenth of a second in bacteria © 2018 Pearson Education Ltd. Figure 17.20_1 Carboxyl end of polypeptide Amino end of polypeptide 1 Codon recognition E 3′ mRNA P A 5′ site site GTP GDP + P i E P A © 2018 Pearson Education Ltd. Figure 17.20_2 Carboxyl end of polypeptide Amino end of polypeptide 1 Codon recognition E 3′ mRNA P A 5′ site site GTP GDP + P i E P A 2 Peptide bond formation E P A © 2018 Pearson Education Ltd. Figure 17.20_3 Carboxyl end of polypeptide Amino end of polypeptide 1 Codon recognition E 3′ mRNA Ribosome ready for P A next aminoacyl tRNA 5′ site site GTP GDP + P i E E P A P A GDP + P i 2 Peptide bond 3 Translocation GTP formation E P A © 2018 Pearson Education Ltd. Termination of Translation Elongation continues until a stop codon in the mRNA reaches the A site of the ribosome The A site accepts a protein called a release factor The release factor causes the addition of a water molecule instead of an amino acid This reaction releases the polypeptide through Exit tunnel, and the translation assembly comes apart using two GTP molecules © 2018 Pearson Education Ltd. Figure 17.21_1 Release factor 3′ 5′ Stop codon (UAG, UAA, or UGA) 1 Ribosome reaches a stop codon on mRNA. © 2018 Pearson Education Ltd. Figure 17.21_2 Release Free factor polypeptide 3′ 3′ 5′ 5′ Stop codon (UAG, UAA, or UGA) 1 Ribosome reaches a stop 2 Release factor promotes codon on mRNA. hydrolysis. © 2018 Pearson Education Ltd. Figure 17.21_3 Release Free factor polypeptide 5′ 3′ 3′ 3′ 5′ 5′ 2 GTP 2 GDP + 2 P i Stop codon (UAG, UAA, or UGA) 1 Ribosome reaches a stop 2 Release factor promotes 3 Ribosomal subunits codon on mRNA. hydrolysis. and other components dissociate. © 2018 Pearson Education Ltd. Making Multiple Polypeptides in Bacteria and Eukaryotes Multiple ribosomes can translate a single mRNA simultaneously, forming a polyribosome (or polysome) Polyribosomes enable a cell to make many copies of a polypeptide very quickly © 2018 Pearson Education Ltd. A bacterial cell ensures a streamlined process by coupling transcription and translation In this case the newly made protein can quickly diffuse to its site of function © 2018 Pearson Education Ltd. Figure 17.24 RNA polymerase DNA mRNA Polyribosome 0.25 µm RNA Direction of polymerase transcription DNA Polyribosome Polypeptide (amino end) Ribosome mRNA (5′ end) © 2018 Pearson Education Ltd. In eukaryotes, the nuclear envelope separates the processes of transcription and translation RNA undergoes processing before leaving the nucleus © 2018 Pearson Education Ltd. Figure 17.23 Growing polypeptides Completed Incoming polypeptide ribosomal subunits Polyriboso me Start of End of mRNA mRNA (5′ end) (3′ end) (a) Several ribosomes simultaneously translating one mRNA molecule Ribosomes mRNA 0.1 µm (b) A large polyribosome in a bacterial cell (TEM) © 2018 Pearson Education Ltd. Figure 17.25a DNA TRANSCRIPTION 3′ A ly- Po 5′ RNA RNA polymerase transcript Exon RNA RNA transcript PROCESSING (pre-mRNA) Intron Aminoacyl-tRNA y-A Pol synthetase NUCLEUS Amino acid AMINO ACID CYTOPLASM ACTIVATION tRNA mRNA 5′ Cap Aminoacyl (charged) tRNA © 2018 Pearson Education Ltd. Figure 17.25b mRNA Growing polypeptide 5′ Cap 3′ A -A Aminoacyl E P oly P Ribosomal (charged) subunits tRNA 5′ Cap TRANSLATION C U A C A E A C A A A Anticodon U G G U U U A U G Codon Ribosome © 2018 Pearson Education Ltd. BioFlix Animation: Protein Synthesis © 2018 Pearson Education Ltd. Animation: Translation © 2018 Pearson Education Ltd. Figure 17.UN02 DNA TRANSCRIPTION Pre-mRNA RNA PROCESSING mRNA TRANSLATION Ribosome Polypeptide © 2018 Pearson Education Ltd. Figure 17.UN03 DNA TRANSCRIPTION Pre-mRNA RNA PROCESSING mRNA TRANSLATION Ribosome Polypeptide © 2018 Pearson Education Ltd. Figure 17.UN04 DNA TRANSCRIPTION mRNA Ribosome TRANSLATION Polypeptide © 2018 Pearson Education Ltd. Figure 17.UN05d Ribosome binding site on mRNA 5′ 3′ © 2018 Pearson Education Ltd. Figure 17.UN07 Transcription unit Promoter 5′ 3′ 3′ 3′ 5′ 5′ Template strand RNA polymerase of DNA RNA transcript © 2018 Pearson Education Ltd. Figure 17.UN08 5′ Cap Poly-A tail 5′ Exon Intron Exon Intron Exon 3′ Pre-mRNA RNA splicing mRNA 5′ UTR Coding 3′ UTR segment © 2018 Pearson Education Ltd. Figure 17.UN09 Polypeptide Amino acid tRNA E A Anti- codon Codon mRNA Ribosome © 2018 Pearson Education Ltd.