15.8-15.11 PDF: Synaptic Transmission and Striated Muscle
Document Details
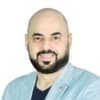
Uploaded by DR.MOATAZ
Tags
Summary
This document provides a detailed explanation of synaptic transmission and striated muscle, with diagrams. The text covers topics such as acetylcholine's role, calcium ions, and the processes involved.
Full Transcript
# Synaptic transmission and Striated Muscle ## 15.8 Synaptic transmission - **On these pages you will learn to:** - Describe a cholinergic synapse - Explain how it functions, including the role of calcium ions **Figure 1 shows transmission across a chemical synapse that involves the neuro...
# Synaptic transmission and Striated Muscle ## 15.8 Synaptic transmission - **On these pages you will learn to:** - Describe a cholinergic synapse - Explain how it functions, including the role of calcium ions **Figure 1 shows transmission across a chemical synapse that involves the neurotransmitter acetylcholine.** 1. The arrival of an action potential at the end of the presynaptic neurone causes voltage-gated calcium channels to open and calcium ions ($Ca^{2+}$) enter the synaptic knob. 2. The influx of calcium ions into the presynaptic neurone causes synaptic vesicles to fuse with the presynaptic membrane, so releasing acetylcholine by exocytosis into the synaptic cleft. Acetylcholine diffuses across the synaptic cleft to the postsynaptic neurone. 3. Acetylcholine molecules bind with receptor sites on two of the five protein sub-units that make up each sodium channel on the postsynaptic membrane. This causes the sodium channels to open, allowing sodium ions ($Na^{+}$) to diffuse in rapidly down a concentration gradient. 4. The influx of sodium ions generates a new action potential in the postsynaptic neurone. This is called the excitatory postsynaptic potential (EPSP). 5. The enzyme acetylcholinesterase hydrolyses acetylcholine into choline and acetate, which diffuse back across the synaptic cleft into the presynaptic neurone (= recycling). 6. ATP released by mitochondria is used to recombine choline and acetate into acetylcholine. This is stored in synaptic vesicles for future use. Sodium channels close in the absence of acetylcholine in the receptor sites. **Figure 1 Mechanism of transmission across a cholinergic synapse** ## **Summary Test 15.8** When an action potential arrives at the end of the presynaptic neurone, (1) ions enter the synaptic knob. These ions cause (2) to fuse with the presynaptic membrane so releasing acetylcholine, by the process of (3), into the (4). Channels on the postsynaptic neurone have receptor sites to which acetylcholine binds, causing them to open and allowing (5) ions to move in by the process of (6). The influx of these ions generates a new action potential known as the (7) potential. ## 15.9 Striated muscle - **On these pages you will learn to:** - Describe the ultrastructure of striated muscle with particular reference to sarcomere structure Muscles are effector organs that respond to nervous stimulation by contracting and so bring about movement. Striated muscle makes up the bulk of body muscle in vertebrates. It is attached to bone and acts under voluntary, conscious control. A rope is made up of millions of separate threads. Each thread has very little individual strength and can easily be snapped. Yet grouped together in a rope, these threads can support a mass running into hundreds of tonnes. Individual muscles are made up of thousands of muscle fibres, bundled in groups, and running parallel to the length of the muscle. Each muscle fibre is a mulitnucleate (many nuclei) cell containing many parallel myofibrils (see Figure 1). Early in development, separate muscle cells fuse to give a single muscle fibre. This fusion allows the muscle to contract efficiently and gives the muscle overall strength. Surrounding the myofibrils, is the muscle fibre cytoplasm, known as sarcoplasm, containing a large concentration of mitochondria and endoplasmis reticulum. **Microscopic structure of striated muscle** We can see from Figure 1 that each muscle fibre is made up of myofibrils. Myofibrils are made up of two types of protein filament: - Actin, which is thinner and consists of two strands twisted around one another - Myosin, which is thicker and consists of long rod-shaped fibres with bulbous heads that project to the side. **The arrangement of these filaments is shown in Figure 2.** Myofibrils appear striped due to their alternating light-coloured and dark-coloured bands. The light bands are called isotropic bands (I-bands). They appear lighter because the actin and myosin filaments do not overlap in this region. The dark bands are called anisotropic bands (A-bands). They appear darker because the actin and myosin filaments overlap in this region. **Figure 1 The gross and microscopic structure of striated muscle** At the centre of each anisotropic band is a lighter coloured region called the H-zone. At the centre of each isotropic band is a line called the Z-line. The distance between adjacent Z-lines is called a sarcomere (Figure 1). When a muscle contracts, these sarcomeres shorten and the pattern of light and dark bands change. Two other important proteins are found in muscle: - Tropomyosin, which forms a fibrous strand around the actin filament - Troponin, a globular protein involved in muscle contraction. **The neuromuscular junction** The neuromuscular junction is the point at which a motor neurone meets a striated muscle fibre. There are many such junctions along the muscle. If there were only one junction of this type it would take time for a wave of contraction to travel across the muscle - in which case, not all the fibres would contract simultaneously and the movement would be slow. As rapid muscle contraction is frequently essential for survival, there are many neuromuscular junctions spread throughout the muscle. This is to ensure that contraction of a muscle is rapid and powerful when it is simultaneously (at the same time) stimulated by action potentials. All muscle fibres supplied by a single motor neurone act together as a single functional unit and are known as a motor unit. This arrangement gives control over the force that the muscle exerts. If only slight force is needed, only a few units are stimulated. If a greater force is required, a larger number of units are stimulated. When a nerve impulse is received at the neuromuscular junction, the synaptic vesicles fuse with the presynaptic membrane and release acetylcholine. The acetylcholine diffuses to the postsynaptic membrane, altering its permeability to sodium ions, which enter rapidly, depolarising the membrane. The description of how this leads to the contraction of the muscle is given in Topic 15.10. The acetylcholine is broken down by acetylcholinesterase to ensure that the muscle is not over-stimulated. The resulting choline and acetate diffuse back into the neurone, where they are recombined to form acetylcholine using energy provided by the mitochondria found there. **The structure of a neuromuscular junction is shown in Figure 4.** **Figure 3 Transmission electron micrograph of striated muscle** **Figure 4 The neuromuscular junction** **Figure 5 Photomicrograph of a neuromuscular junction** **Remember:** To help you remember which band is the dark band and which is the light one, look at the vowels in 'light' and 'dark'. In each one, the vowel is the first letter of the relevant band. Therefore, the dark band is the A-band and the light band is the I-band. ## 15.10 Muscle contraction - **On these pages you will learn to:** - Describe the roles of neuromuscular junctions, transverse system tubules and sarcoplasmic reticulum in stimulating contraction in striated muscle - Explain the sliding filament model of muscular contraction including the roles of troponin, tropomyosin, calcium ions and ATP Having looked at the structure of striated muscle in Topic 15.9, let us turn our attention to how exactly the arrangement of the various proteins brings about contraction of the muscle fibre. The process involves the actin and myosin filaments sliding past one another and is therefore called the sliding filament mechanism. **Evidence for the sliding filament mechanism** In Topic 15.9 we saw that myofibrils appear darker in colour where actin and myosin filaments overlap and lighter where they do not. If the sliding filament mechanism is correct, then there will be more overlap of actin and myosin in a contracted muscle than in a relaxed one. Look at Figure 1. You will see that when a muscle contracts, the following changes occur to a sarcomere: - The I-band becomes narrower - The Z-lines move closer together (in other words, the sarcomere shortens) - The H-zone becomes narrower. The A-band remains the same width. As the width of this band is determined by the length of the myosin filaments, it follows that the myosin filaments have not become shorter. **Figure 1 Comparison of two sarcomeres in a relaxed and a contracted muscle** Before we look at how the sliding filament mechanism works, let us take a closer look at the four main proteins involved in the process. - **Myosin** (see Figure 2) Each myosin filament is made up of a several hundred myosin molecules. Each myosin molecule is composed of several polypeptide chains and is made up of a globular 'head' section and a long 'tail' region. **Figure 2 Structure of the myosin filament** - **Actin** is a globular protein whose molecules are arranged into long chains that are twisted around one another to form a helical strand. - **Tropomyosin** forms long thin threads that are wound around actin filaments. - **Troponin** is a globular protein attached to tropomyosin to form a troponin-tropomyosin complex. **The arrangement of the molecules of actin and tropomyosin are shown in Figure 3.** **Figure 3 The relationship of tropomyosin and troponin to an actin filament** **The sliding filament mechanism of muscle contraction** The hypothesis that actin and myosin filaments slide past one another during muscle contraction is supported by the changes in the band pattern on myofibrils. The next question that scientists had to answer was: by what mechanism do the filaments slide past one another? Clues to the answer lie in the shape of the various proteins involved. To summarise: the bulbous heads of the myosin filaments form cross bridges with actin filaments. They do this by attaching themselves to binding sites on the actin filaments, and then flexing (bending) in unison, pulling the actin filaments along the myosin filaments. They then become detached and, using ATP as a source of energy, return to their original angle and reattach themselves further along the actin filament. This process is repeated up to 100 times a second. The procedure is similar to the way a ratchet operates. The following account describes the sliding filament of muscle contraction in detail. The process is continuous but for ease of understanding has been divided into muscle stimulation, contraction and relaxation. **Remember:** The action of the myosin heads is similar to the rowing action of rowers in a boat. The oars (myosin heads) are dipped into the water, flexed as the rowers pull on them, removed from the water and then dipped back into the water further along. The rowers work in unison and the boat and water move relative to one another. **Muscle stimulation** - An action potential reaches many neuromuscular junctions simultaneously, causing calcium channels to open and calcium ions to move into the synaptic knob. - The calcium ions cause synaptic vesicles to fuse with the presynaptic membrane and release their acetylcholine into the synaptic cleft. - Acetylcholine diffuses across the synaptic cleft and binds with receptors on the postsynaptic membrane, causing it to depolarise. **Muscle contraction** This part of the process is illustrated in Figure 4. - The action potential travels deep into the fibre through a network of tubules called transverse system tubules (T-tubules) that branch throughout the cytoplasm of the muscle (sarcoplasm). - The tubules are in contact with the endoplasmic reticulum of the muscle, known as the sarcoplasmic reticulum, which have actively absorbed calcium ions from the sarcoplasm. - The action potential opens the calcium channels on the endoplasmic reticulum and calcium ions flood into the muscle cytoplasm down a diffusion gradient. - Some calcium ions attach to troponin and cause the troponin-tropomyosin complex to change shape. - The change in shape of the troponin-tropomyosin complex causes the tropomyosin molecules that were blocking the binding sites on the actin filaments to be displaced (Figure 4, stages 1 and 2). - The ADP molecule attached to the myosin heads means they are now in a state to bind to the actin filament and form a cross-bridge (Figure 4, stage 3). This means that the ATP that was previously attached has been hydrolysed and the energy released can be used for the next stage. - Once attached to the actin filament, the myosin heads change their angle, pulling the actin filament along as they do so (power stroke) and releasing a molecule of ADP (Figure 4, stage 4). - An ATP molecule attaches to each myosin head, causing it to become detached from the actin filament (Figure 4, stage 5). - The calcium ions then activate the myosin molecule, which is also an ATPase that catalyses the hydolysis ATP to ADP (Figure 4, stage 6). - The myosin head, once more with an attached ADP molecule, then reattaches itself further along the actin filament and the cycle is repeated as long as nervous stimulation of the muscle continues (Figure 4, stage 7). **Muscle relaxation** - When nervous stimulation ceases, calcium ions are actively transported back into the sarcoplasmic reticulum using energy from the hydrolysis of AТР. - This reabsorption of the calcium ions allows tropomyosin to block the actin filament again. - Myosin heads are now unable to bind to actin filaments and contraction stops, i.e. the muscle relaxes. **Remember:** An action potential is the result of depolarisation of part of a membrane. The spread of the action potential across the muscle is therefore often referred to as a 'wave of depolarisation'. **Remember:** The hydrolysis of ATP releases energy and produces ADP and inorganic phosphate (Pi). For simplicity, this account just refers to ADP as the product. **Summary test 15.10** According to the sliding filament theory of muscle contraction, when a muscle contracts the (1) move closer together and the (2) and (3) become narrower. For contraction to occur an (4) must reach the (5), where it travels deep into a network of tubules called (6) tubules. These tubules are connected to the endoplasmic reticulum of the muscle, which is called the (7). Some of the calcium ions from the endoplasmic reticulum attach to a protein molecule called (8), which changes shape and causes the displacement of a molecule called (9) from the actin filament. This displacement allows the head of a (10) molecule to attach to the actin filament and to change the angle of its head and so pull the actin filament along. The molecule detaches from the actin filament when a molecule of (11) attaches to it. **Figure 4 Sliding filament mechanism of muscle contraction (showing only one myosin head throughout)**