Enzymology 15316 Lecture 9 - Factors Affecting Enzyme Reaction PDF
Document Details
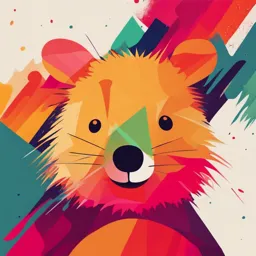
Uploaded by ManeuverableSerpentine5206
Dr Hassan Aboufarrag
Tags
Summary
This document details the factors affecting enzyme activity in Enzymology 15316, including temperature, pH, substrate concentration, and enzyme concentration. It also covers enzyme inhibitors and their types. The content is geared towards an understanding of enzyme mechanisms and biochemistry.
Full Transcript
Enzymology 15316 I. Factors affecting enzyme activity Different enzymes show different responses to changes in substrate and enzyme concentration, temperature, pH, inhibitors and activators. Enzymic responses to these factors give us valuable clues as to how enzymes function in...
Enzymology 15316 I. Factors affecting enzyme activity Different enzymes show different responses to changes in substrate and enzyme concentration, temperature, pH, inhibitors and activators. Enzymic responses to these factors give us valuable clues as to how enzymes function in living cells (that is, in vivo). 1. Temperature Velocity increase with temperature. This increase is the result of the increased kinetic energy of substrate to pass over the energy barrier and form the products of the reaction. Increasing the kinetic energy also increases their motion and therefore the frequency of their collision and approaching within bond-forming distance. However, the reaction velocity increases with temperature until a peak velocity is reached. Further elevation of the temperature causes a decrease in reaction velocity as a result of denaturation of the enzyme’s protein. The optimum temperature for most human enzymes is between 35°C and 40°C. Human enzymes start to denature at temperatures above 40°C, but thermophilic bacteria found in hot springs have optimum temperatures of 70°C. 2. Hydrogen Ion Concentration (pH) The rate of almost all enzyme-catalyzed reactions exhibits a significant dependence on hydrogen ion concentration. pH affects the ionization state of the enzyme (mainly the active site), the substrates, or both. Each enzyme has an optimum pH range. Changing the pH outside of this range will slow enzyme activity. Extremes of pH can also lead to denaturation of the enzyme. For example, catalytic activity may require that an amino group of the enzyme be in the protonated form (NH 3+). Because this group is deprotonated at alkaline pH, the rate of the reaction declines at high pH. The pH at which maximal enzyme activity is achieved is different for different enzymes and often reflects the [H+] at which the enzyme works in the body. For example, pepsin, a digestive enzyme in the stomach, is maximally active at pH 2, whereas other enzymes, designed to work at neutral pH, are denatured by such an acidic environment. 3. Substrate Concentration [S] The rate of an enzymatic reaction increases with substrate concentration [S] until a maximal velocity (Vmax) is reached. At low concentration [S], the catalytic site of the enzyme is empty, so, there is a steep increase in the rate of reaction with increasing [S] until the enzyme becomes saturated with substrate and any substrate increase will have no effect on the rate of reaction. Most enzymes show Michaelis- Menten kinetics (the plot of initial velocity (v0) against [S] is hyperbolic). In contrast, allosteric enzymes show a sigmoidal curve 4. Enzyme Concentration [E] Increasing enzyme concentration [E] will speed up the reaction, as long as there is substrate available to bind to. Once all of the substrate is bound, the reaction will no longer speed up, since there will be nothing for additional enzymes to bind to. The relation between [E] and reaction rate is hyperbolic. Dr Hassan Aboufarrag 1 Enzymology 15316 5. Inhibitors Enzyme inhibitors (I) are small molecules that binds to an enzyme and interferes with catalysis, slowing or halting enzymatic reactions. Inhibitors can act by preventing the formation of the ES complex or by blocking the chemical reaction that leads to the formation of product. The equilibrium between free enzyme (E), inhibitor (I) and the EI complex is characterized by a dissociation constant. In this case, the constant is called the inhibition constant, Ki. The smaller the Ki, the more potent the inhibition. Enzyme inhibition serves as a major control mechanism in biological systems. So, it should not be surprising that enzyme inhibitors are among the most important pharmaceutical agents that used in many drugs and toxic agent. For example, aspirin (acetylsalicylate) inhibits the enzyme that catalyzes the first step in the synthesis of prostaglandins, compounds involved in producing pain. The study of enzyme inhibitors also has helped studying enzyme mechanisms to define and decipher some metabolic pathways ▪ Classes of Enzyme Inhibitors There are two broad classes of enzyme inhibitors: (A) Reversible and (B) Irreversible. A. Reversible Inhibitors: Most enzyme inhibitors act reversibly. They do not cause any permanent changes in the enzyme. Most biologically relevant inhibition is reversible. Reversible inhibitors are bound to enzymes by the same weak noncovalent forces that bind substrates and products with enzyme (such as hydrogen bonds, ionic bonds or hydrophobic bonds). The basic types of reversible inhibition are competitive, uncompetitive, noncompetitive and mixed. These can be distinguished experimentally by their effects on the kinetic behavior of the inhibited and uninhibited reactions. A.1. Competitive Inhibitors Competitive inhibitors are the most commonly encountered inhibitors in biochemistry. In competitive inhibition, the inhibitor can bind only to free enzyme molecules that have not bound any substrate. Once a competitive inhibitor is bound to an enzyme molecule, a substrate molecule cannot bind to that enzyme molecule. Conversely, the binding of substrate to an enzyme molecule prevents the binding of an inhibitor. In other words, S and I compete for binding to the enzyme molecule. Most commonly, S and I bind at the same site on the enzyme, the active site. Dr Hassan Aboufarrag 2 Enzymology 15316 When both I and S are present in a solution, the proportion of the enzyme that is able to form ES complexes depends on the concentrations of substrate and inhibitor and their relative affinities for the enzyme. The amount of EI can be reduced by increasing the concentration of S. At sufficiently high concentrations the enzyme can still be saturated with substrate. Therefore, the maximum velocity is the same in the presence or in the absence of an inhibitor. The more competitive inhibitor present, the more substrate needed for half-saturation (Km). In the presence of increasing concentrations of a competitive inhibitor, Km increases (1/Km decreases in double reciprocal or Lineweaver-Burk plot) meaning that more substrate is needed to obtain the same reaction rate. The new value is usually referred to as the apparent Km (Kmapp). Many classical competitive inhibitors are substrate analogs—compounds that are structurally similar to substrates, but, they do not react. For example, the enzyme succinate dehydrogenase converts succinate to fumarate. Malonate resembles succinate and acts as a competitive inhibitor of the enzyme. Many drugs are competitive inhibitors. Statins are drugs that reduce high cholesterol levels by competitively inhibiting a key enzyme in cholesterol biosynthesis (hydroxymethylglutaryl coenzyme A reductase, HMG CoA reductase). Statins are structural analogs of the natural substrate for this enzyme and compete effectively to inhibit HMG CoA reductase lowering plasma cholesterol levels. Ibuprofen, the active ingredient in many over-the-counter painkillers, is a competitive inhibitor of the enzyme cyclooxygenase. A.2. Uncompetitive Inhibitors An uncompetitive inhibitor binds at a site distinct from the substrate active site and, unlike a competitive inhibitor, binds only to the ES complex not to free enzyme to form enzyme–substrate– inhibitor complex, ESI. An uncompetitive inhibitor need not resemble the substrate of the reaction it is inhibiting. This is the opposite for competitive inhibition in which inhibitors resemble the substrate and bind with free enzyme. In uncompetitive inhibition, ESI, does not go on to form any product. Because the enzyme is inactive when the uncompetitive inhibitor is bound (ESI), the inhibitor effectively removes (depletes) some fraction of the enzyme molecules from the reaction by conversion of ES into ESI. Given that Vmax depends on [E], the observed Vmax decreases. Uncompetitive inhibitors also decrease the Km (1/Km increases in double reciprocal or Lineweaver- Burk plot) by the same amount. Since the inhibitor does not compete with the substrate for the same binding site, the inhibition cannot be overcome by increasing the substrate concentration. Inhibitors with purely uncompetitive effects are rare. The herbicide glyphosate, also known as Roundup, is an uncompetitive inhibitor of an enzyme essential for aromatic amino acids biosynthesis. This mode of action is attractive for drug design as the inhibitors bind to the enzyme target only when the target is active and substrate present. Human alkaline phosphatases have been found to be over-expressed in certain types of cancers. These enzymes are inhibited uncompetitively by amino acids such as leucine and phenylalanine A.3. Noncompetitive inhibitors Noncompetitive inhibition occurs when the inhibitor and substrate bind at different sites on the enzyme. The noncompetitive inhibitor can bind either to free enzyme or the enzyme–substrate complex, thereby preventing the reaction from occurring. Noncompetitive inhibitors generally bear Dr Hassan Aboufarrag 3 Enzymology 15316 little or no structural resemblance to the substrate. Noncompetitive inhibition cannot be overcome by increasing the concentration of substrate. Noncompetitive inhibitors decrease the apparent Vmax of the reaction. Since they do not interfere with the binding of substrate to enzyme, noncompetitive inhibitors do not affect Km value. Most enzymes do not conform to the classic form of noncompetitive inhibition where Km is unchanged. In most cases, both Km and Vmax are affected (the Km may increase or decrease based on the difference of affinity of the inhibitor to bind with E or with ES. These cases are often referred to as mixed inhibition. B. Irreversible Inhibitors Irreversible inhibitors permanently modify the target enzyme. Irreversible inhibitors are tightly bound to the enzyme, (mainly by covalent bonds, however, noncovalent binding is enough if that binding is so tight that is hard to dissociate the inhibitor). Therefore, the inhibitor dissociates very slowly from its target enzyme. Some irreversible inhibitors are important drugs. Penicillin acts by covalently modifying the enzyme transpeptidase, thereby preventing the synthesis of bacterial cell walls and thus killing the bacteria. Aspirin acts by covalently modifying the enzyme cyclooxygenase, reducing the synthesis of signaling molecules in inflammation. Irreversible inhibitors are very important to determine what functional groups are required for enzyme activity, the first step in understanding the mechanism of enzyme reactions. Irreversible inhibitors can be divided into three categories: group-specific reagents, reactive substrate analogs (also called affinity labels), and suicide inhibitors. B.1. Group-specific Inhibitors These types of inhibitors react with specific side chains of amino acids destroying the functional group that is essential for the enzyme’s activity. An example of a group-specific reagent is diisopropylphosphofluoridate (DIPF). DIPF modifies serine residues in the proteolytic enzyme chymotrypsin so inhibits the enzyme, implying that this serine residue is especially reactive. DIPF also revealed a reactive serine residue in acetylcholinesterase, an enzyme important in the transmission of nerve impulses. Thus, DIPF and similar compounds that bind and inactivate acetylcholinesterase are potent nerve gases. B.2. Reactive Substrate Analogs (Affinity labels) These are molecules that are structurally similar to the substrate for an enzyme. However, they covalently bind to active-site residues. Therefore, that the enzyme is irreversibly inhibited. They are thus more specific for the enzyme’s active site than are group- specific reagents. B.3. Suicide Inhibitors A special class of irreversible inhibitors is the suicide inhibitors. These compounds are relatively unreactive until they bind to the active site. They undergo the first few chemical steps of the normal enzymatic reaction, but instead of being transformed into product, they are converted into reactive compounds that combine irreversibly with the enzyme. Dr Hassan Aboufarrag 4 Enzymology 15316 6. Activators Enzyme activators are chemical compounds that increase a velocity of enzymatic reaction. Their actions are opposite to the effect of enzyme inhibitors. Among activators we can find ions, small organic molecules, as well as peptides, proteins, and lipids. A. Activators can bind with enzyme: Some enzymes usually have special site for activators. The binding of activators results in the change of enzyme conformation that increase enzyme activity. Example are enzymes that are specifically and directly activated by small inorganic molecules, mainly by cations such as Ca2+ (phospholipases, protein kinases). B. Activators can bind with substrate: Activators can bind with the substrate increasing its affinity to the enzyme that increase the rate of the enzyme reaction. For example, magnesium ions interact with ATP or with other nucleotides that are negatively charged molecules, decreasing their charge that provides effective binding of nucleotides (substrates) with the enzymes and increasing their activity. C. Activators may eliminate inhibitors: Some cations including heavy metal cations inhibit definite enzymes. Chelating agent such as ethylene glycol tetraacetic acid (EGTA) and ethylenediaminetetraacetic acid (EDTA) bind these inhibitory cations and eliminate their inhibitory effect. In total this effect looks as enzyme activation. II. Isoenzymes Isoenzymes (also called isozymes) are enzymes that catalyze the same reaction but differ slightly in structure and more obviously in Km and Vmax values as well as in regulatory properties. Often, isozymes are expressed in a different tissue or organelle, or at a different stage of development. Because isoenzymes contain different amino acid sequence and different numbers of charged amino acids, their electrophoresis pattern is different. Isoenzymes provide a way for varying regulation of the same reaction to meet the specific physiological needs in the particular tissue at a particular time. In addition, isoenzymes are a good tool for diagnosis. Important example is lactate dehydrogenase (LDH) enzyme. There are two different LDH subunits in the organism—M and H—which have a slightly different amino acid sequence and consequently different catalytic properties. As these two subunits associate to form tetramers, they form a total of five different isoenzymes. the H subunit contains more acidic and fewer basic residues than the M form, and it therefore has a more strongly negative charge. Therefore, the isoenzyme LDH-1 (consisting of four H subunits) migrates fastest, and the LDH-5 (consisting of four M subunits) isoenzyme is slowest. Normally, only small amounts of enzyme activity are found in serum. When an organ is damaged, intracellular enzymes enter the blood and can be demonstrated in serum enzyme diagnosis). The total activity of an enzyme reflects the severity of the damage, while the type of isoenzyme found in the blood reflects the site of injury. Following cardiac infarction, for example, there is a strong increase in LDH-1 in the blood, while the concentration of LDH-5 hardly changes. Dr Hassan Aboufarrag 5