DNA Replication and Gene Expression PDF Lecture Notes
Document Details
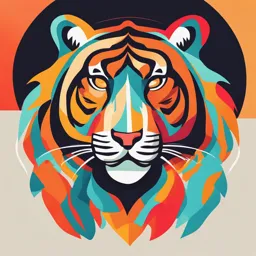
Uploaded by DelightfulCopper4960
Tags
Summary
These lecture notes cover DNA replication and gene expression. Topics include the role of DNA, discovery of DNA, DNA structure, origins of replication, and DNA replication. The notes also discuss the experiments of Griffith, Avery/MacLeod/McCarty, and Hershey-Chase.
Full Transcript
Lecture 15 DNA Replication and Gene Expression Chapter 8 in the textbook 1 Outline: The role of DNA Discovery of DNA DNA Structure Origins of Replication DNA replication 2 The R...
Lecture 15 DNA Replication and Gene Expression Chapter 8 in the textbook 1 Outline: The role of DNA Discovery of DNA DNA Structure Origins of Replication DNA replication 2 The Role of DNA How do we know that DNA is the hereditary molecule? o The process by which cells use hereditary information stored in DNA is very elegant. DNA must be retained intact yet copied to make new cells (DNA replication). It must be turned into multiple “working copies” in the form of RNA to provide instructions to produce enzymes/structural proteins (transcription). The RNA molecules must be read and decoded to form the enzymes/structural proteins of the cell (translation). The systems must have the ability to deal with damage to the core DNA molecules of the cell (DNA repair). 3 The Role of DNA o The Griffith experiment (1920s) One of the earliest experiments that pointed out that DNA could contribute hereditary information in bacteria was the work of Griffith in the 1920s with smooth (S) and rough (R) strains of S. pneumoniae. His work hinted at the idea of something (he didn’t know what at the time) that could transform a nonpathogenic R strain into a pathogenic S strain of microbes when the two were mixed together. 4 The Role of DNA o The Avery, MacLeod, and McCarty experiment (1940s) Attempted to determine if it was DNA, RNA or protein that was responsible for the “transformation” effect observed in Griffith’s experiments. Used mixtures obtained from S strains but digested away one component at a time. Only the mixture with DNA left over could still transform R cells into S cells, indicating DNA was the molecule responsible for transformation. 5 The Role of DNA o The Hershey‒Chase experiment (1950s) Some biologists thought that contaminating bits of protein or RNA could still be present in the DNA-only mixture of the Avery/MacLeod/McCarty experiments. Hershey and Chase used radioactive labeling of either proteins or DNA in bacteriophages. They let the labeled phages infect bacterial cells, then determined where the tag ended up for each setup. Only the labeled phage DNA went into the bacterial cells, further proving that DNA (not protein) was the hereditary molecule in these experiments. 6 The Role of DNA Structure of DNA o Despite all of this evidence on what the hereditary molecule of cells was, the structure was still not well characterized. o The work of Rosalind Franklin, Maurice Wilkins, James Watson, and Francis Crick in the early 1950s elucidated the now-well-known double-stranded double helix structure of DNA. o Each nucleotide building block of DNA consists of A five-carbon sugar, 2-deoxyribose A phosphate group attached to the 5' carbon of the sugar A nitrogenous base attached to the 1' carbon of the sugar 7 The Role of DNA Structure of DNA o One strand of DNA is complementary to the other strand. o Two complementary strands arranged in opposite orientations (5’ to 3’) o A pairs with T – 2 hydrogen bonds o G pairs with C – 3 hydrogen bonds o Phosphodiester covalent bonds form the sugar/phosphate backbone of each strand. A. Structure of B. Structure of nucleotide polymer double-stranded DNA 8 The Role of DNA Structure of DNA o The circular chromosome in bacterial cells is supercoiled, causing the molecule to form a tightly compacted structure o The wrapping of dsDNA around histones helps compact the very large chromosome structures of eukaryotic cells. DNA is organized around a core eight histone proteins that interact with the DNA in a very specific fashion, forming nucleosomes An additional histone, H1, stabilizes the nucleosome structure 9 DNA Replication How does DNA replicate? o DNA replication is a semiconservative process. This means that each time the dsDNA is copied, each copy carries one strand of the original molecule, and one newly made strand 10 DNA Replication Origins of replication – Bacteria o Even after the structure of DNA was revealed, how it was copied took more research. Approximately 10 years after Watson–Crick’s work, Jacob, Brenner, and Cuzin discovered how DNA replication was initiated in E. coli cells. They proposed the process would require two main components—a replicator and an initiator. Bacteria were an excellent model system to study DNA replication. DNA replication begins at a specific sequence in the molecule called the origin of replication (oriC) 11 DNA Replication Origins of replication – Bacteria Bacterial replication initiation o DnaA protein binds to oriC. o DnaB (a helicase) is recruited with DnaC (a helicase loader). o DnaG (a primase) is recruited to lay down initial RNA primers needed for DNA polymerases to work. o Single-stranded DNA binding proteins (SSBs) are recruited to help keep the DNA unwound. Replication bubble 12 DNA Replication Origins of replication – Eukarya o Eukaryal replication initiation Multiple origins of replication (ARS) on each chromosome Chromosomes are much larger, so they need multiple starting points for replication Studied in yeast (Saccharomyces cerevisiae) ORC – origin recognition complex MCM – mini-chromosomal maintenance complex Very similar to process in bacteria, just using different proteins Replication mechanism in archaea is more closely resemble eukarya 13 DNA Replication Initiation and elongation – Bacteria o Once the replication fork forms, DNA polymerase III adds nucleotides to the initial RNA primers (synthesized by primase) o All DNA polymerases only add new nucleotides onto the free 3′-OH group of a nucleotide o A continuous leading strand and a discontinuous lagging strand (forming Okazaki fragments) are formed o This process is virtually identical in both bacteria and eukarya 14 DNA Replication Initiation and elongation DNA replication of the lagging strand o The lagging strand can’t be left in fragments! DNA polymerase I removes the RNA primers and fills in the gaps with new nucleotides. DNA ligase seals the sugar/phosphate backbone. 15 DNA Replication Initiation and elongation o Many of the enzymes used across the domains are roughly the same. o This shows how the processes of DNA replication are well conserved. DNA replication – 3D: https://www.youtube.co m/watch?v=TNKWgcFP Hqw DNA replication in prokaryotes: https://www.youtube.co m/watch?v=IjVLhoyfGA M 16 DNA Replication Termination of DNA replication of a circular chromosome o In E. coli, a series of ter (termination sites) exist roughly opposite the origin of replication (oriC). o After bidirectional replication begins at oriC, the Tus protein binds to these ter sites and stops the progress of the replication forks. o Topoisomerase II forms a transient break in the DNA, allowing the two circles to become disentangled. 17 DNA Replication Termination of DNA replication in a linear chromosome 18