Signal Transduction II Messengers and Receptors PDF
Document Details
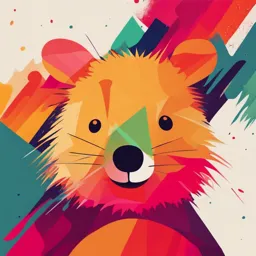
Uploaded by AmiableMoon
null
null
Alvin Hee
Tags
Summary
This document is a lecture or study guide on signal transduction. It covers cell signaling mechanisms, messengers, receptors, and related topics. The document includes a list of recommended readings and learning objectives. It's aimed at an undergraduate-level biology course.
Full Transcript
Signal Transduction Mechanisms: II. Messengers & Receptors Course instructor: Alvin Hee, Ph.D BGY 3002 Cell & Molecular Biology Compulsory reading material 1. Becker, W.M. et al. (2006). The World of the Cell. 6th Edition. California: Pearson Benjamin Cummings...
Signal Transduction Mechanisms: II. Messengers & Receptors Course instructor: Alvin Hee, Ph.D BGY 3002 Cell & Molecular Biology Compulsory reading material 1. Becker, W.M. et al. (2006). The World of the Cell. 6th Edition. California: Pearson Benjamin Cummings. Chapter 14 2. Karp, G. (2008). Cell and Molecular Biology. 5th Edition. New York: John Wiley & Sons. Chapter 15 Learning Outcomes Upon completing this lecture, you are expected to be able to: 1. Distinguish between cell-to-cell signalling by hormones and local mediators. 2. Describe the general flow of information during cell signalling. 3. Arrange the correct sequence of G proteins’ activation and inactivation. 4. Explain briefly the involvement of G protein-adenylyl cyclase in diseases such as cholera. All cells have some ability to sense and respond to specific chemical signals in their environment. Cells communicate with one another by displaying molecules on their surfaces that are recognized by receptors on the surface of other cells Alternatively, cells may release chemical signals that are recognized by another cell, nearby or at a distant location. Signaling molecules are often classified based on the distance between their site of production and the target tissue(s) upon which they act. Some messengers, such as hormones, act as endocrine signals (act away at a distance from the site of production). Others like growth factors, are released locally, where they diffuse to act at short distances on nearby tissues. Such signals are referred to as paracrine signals. Cell-to-Cell Signalling Other local mediators act in the same cell that produce them – autocrine signals. Once a signal reaches its target tissue, it binds to receptor on the surface, triggering a signal process. A ligand is any molecule coming either from a long or short distance to bind to a receptor. The ligand is a primary messenger. Often, the binding of a ligand to a receptor results in the production of additional molecules within the cell receiving the signal- these are known as second messengers. These second messengers are small molecules or ions can initiate a series of events in the cell leading to a change in identity or function of the cell. The ability of a cell to translate a receptor- ligand interaction to changes in its behaviour or gene expression is known as signal transduction. Binding of a ligand by a receptor activates a series of events known now as signal transduction that relays the signal to the interior of the cell, resulting in specific cellular responses and/or changes in gene in expression. How do cells distinguish messengers from the many chemicals in the cells? The answer lies in the specific way the messenger binds to the receptor. Binding is usually via non-covalent bonds. Such binding is similar to the binding of an enzyme to its substrate and have many properties in common – binding constant, saturation kinetics, etc. Signal transduction pathways can amplify the cellular response to an external signal. – An example is the breakdown of glycogen by the liver in response to the hormone, epinephrine. – A cascade effect is obtained ( similar to the blood coagulation cascade). – One molecule of epinephrine is capable of triggering the production of millions of glucose-1- phosphate molecules. Signal Transduction Pathways Can Amplify the Cellular Response to an External Signal. Depending on its mode of action, receptors can be classified into several basic categories: i. Ligand-gated channels ( transport across cellular membranes) ii. Intracellular receptors iii. Plasma membrane receptors We shall focus on the third category which can be further classified into two families - those linked to G-proteins and those linked to protein kinases. The G-Protein linked receptor family, so named because ligand binding causes a change in receptor conformation that activates a particular G-protein (guanine nucleotide binding protein). Structure of G-protein linked receptors. The G protein linked receptors all have a similar structure yet differ significantly in their amino acid sequences. The binding of the ligand to the receptor binding site on the cell surface, the change in conformation ( of the receptor) causes the G- protein to associate with the receptor. The G protein can be described as a type of molecular switch whose “on” and “off” state will depend on whether the G protein is bound to GTP or GDP. Structure of G protein-linked receptors Binding causes receptor to activate an adjacent G protein which composes of 3 subunits- αβγ When a messenger binds to a G protein- linked receptor on the surface of the cell, the change in conformation of the receptor causes the G protein to associate with the receptor, which in turns causes the α subunit of the G protein (Gα subunit) to release its bound GDP, acquire a GTP, and then detach from the complex: Depending on the G protein and cell type, either the free GTP-Gα subunit or the G βγ complex can then initiate signal transduction event in the cell. In this step, a series of events take place: 1. Binding of ligand to G-protein coupled receptor 2. Change in conformation of the cytoplasmic loops of the receptor 3. Increase in the affinity for a G protein on the cytoplasmic surface of the membrane 4. G protein binds to activated receptor forming a receptor-G protein complex 5. Conformational shift in the α subunit of the G protein 6. Release of GDP by the G protein 7. Binding of GTP by the G protein The activity of the G protein persists only as long as the Gα is bound to GTP and the subunits remain separated. Once the GTP is hydrolysed, it will revert to the GDP bound state and reassociate back with Gβγ. This feature allows the signal transduction pathway to shut down rapidly when the messenger is removed. The large number of different G proteins provides for a diversity G protein-mediated signal transduction events. For example, G proteins can activate protein kinases in some organisms and lead to the formation or release of second messengers like cyclic AMP or calcium ions. Cyclic AMP (cAMP) is a second messenger used by one class of G proteins. cAMP appears to have one main intracellular target – cAMP- dependent protein kinase A (PKA). PKA phosphorylates a variety of cellular proteins by transferring a phosphate from ATP to a serine or threonine found within the target protein. The role of G proteins and cAMP in Signal Transduction cAMP is formed from adenylyl cyclase, anchored in the plasma membrane. The enzyme is inactive until by the binding of the activated α subunit of a specific G protein. When a ligand binds to the receptor, this causes the release of GDP and the acquiring of a GTP. This in turn causes the α subunit of the specific G protein to detach and binds to adenylyl cyclase. G proteins mediate signal transduction through G protein-linked receptors: 1. Activation of receptor by ligand binding leads to displacement of GDP by GTP. 2. GTP-Gsα complex binds to and activates membrane-bound adenylyl cyclase that synthesizes cAMP. 3. Activation ends when ligand leaves the receptor, GTP hydrolyzed to GDP by GTPase of the Gsα subunit and Gsα dissociates from adenylyl cyclase. 4. Adenylyl cyclase reverts to the inactive form, Gsα reassociates with the Gsβγ complex. 5. cAMP molecules in the cytosol are hydrolyzed to AMP by phosphodiesterase. The increase in cAMP can produce many different effects in different cell types. In skeletal muscle and liver cells, elevated cAMP levels stimulated the breakdown of glycogen. In cardiac muscle, it strengthens heart contraction, and in smooth muscle it inhibits contraction In blood platelets, elevation of cAMP inhibits mobilisation during blood coagulation and in epithelial cells, it causes the secretion of water and salts into the lumen of the gut. cAMP levels can be elevated in two ways: i. stimulating cAMP synthesis directly ii. Inhibiting its breakdown by the enzyme cAMP phosphodiesterase. Inhibiting cAMP phosphodiesterase allows the cAMP levels to accumulate without being degraded. Examples of phosphodiesterase inhibitors are the methylxanthines ( caffeine, theophylline) – present in tea, coffee and soft drinks. Disruption of G protein signaling causes several human diseases The bacteria Vibrio cholerae (which causes cholera) and Bordetella pertusis (which causes whooping cough) exert their effect on humans through G proteins. The cholera toxin alters secretion of salts ( NaCl and NaHCO3) and fluid in the intestine, which is normally regulated by hormones that act on the G protein Gs to alter intracellular cAMP levels. The toxin chemically modifies Gs such that it can no longer hydrolyse GTP to GDP. As a result, Gs cannot be shut off, cAMP levels remain high, and the intestinal cells secrete large amounts of water and salts. This is cholera. If untreated, it can lead to dehydration and eventually death, especially in young children. The pertussis toxin on the other hand, acts on another G protein called Gi –inhibitory G protein. This protein acts to shut off adenylyl cyclase. However, when inactivated by the toxin, Gi no longer inhibits adenylyl cyclase and this leads to whooping cough. Acknowledgements International Publishers - Wiley International - Pearson Benjamin Cummings Department colleague(s) - Assoc. Prof. Nor Aini M. Fadzillah, Ph.D et al Assoc. Prof. ST Lim, Ph.D