Lecture Notes PDF
Document Details
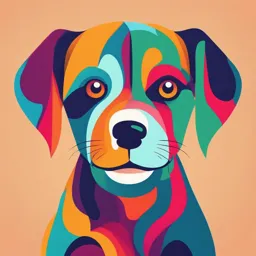
Uploaded by .keeks.
Marian University
Tags
Summary
This document provides a lecture on genetics, focusing on concepts like X-linked dominance, recessive traits and pedigrees. It touches on the complex interactions between genetics, environment, and lifestyle. Additionally, examples are used to illustrate complex diseases, particularly in the case of cancer.
Full Transcript
It's one o'clock, and I know we're gonna be kind of trickling in from those lovely head shots, so we'll sort of take our time and get started. We are picking up with the X-linked dominance piece. As I wander around on camera, I'm just looking like I don't have the space. All right, so what we are tr...
It's one o'clock, and I know we're gonna be kind of trickling in from those lovely head shots, so we'll sort of take our time and get started. We are picking up with the X-linked dominance piece. As I wander around on camera, I'm just looking like I don't have the space. All right, so what we are trying to do with today and Friday session is sort of get through some of the more complicated pieces so that what I record is really just a nice summary and it doesn't feel like we're getting too much extraneous information that's super complicated on an asynchronous day. Because remember, you are encouraged to go to the lovely research day both for the benefit it would present to your capstones and just to see what other people are doing here at the university, right? So I did make one announcement that you probably haven't seen, so I wanna make sure I have it verbally somewhere in this. I have reopened every past quiz to have a due date of tomorrow by 8 p.m. And the reason I do this is to encourage that one last run-through on that material before we start applying it like crazy in block four. So that extra time means any maybe some missed points because we kind of ran out of time in a previous block or we just didn't quite get there with that. We now have that built-in practice. The quizzes remain available to you but you will only be able to get credit for them up until 8 p.m. tomorrow. So that's an additional 24-hour period with a little bit of wiggle room of about seven hours there to help you find those places where you are missing information, especially from block three to block four so that we can really have the strongest start for block four that we can, right? Everything we've done so far has led to what we are doing in this block. The only reason that I do that is because the course is cumulative. Whereas all of the other courses have those like set blocks. This is the one that has the most bleed-through so I want you to have that extra time. Does that make sense? You don't have to do them again if you've already done them and are happy with the points but the opportunity is there for you. Okay, so let's get back into X-linked dominance. The most important thing when trying to discern *** linkage versus autosomal is being able to compare the parent to child specifically mother to son, father to daughter. If it is a dominant condition that the male is affected with, what should we see? All of the daughters should be affected, correct? If it is a dominant condition that the mother is affected with, it's not as direct and that side. When does it become 100% of her son's being affected when it's recessive? Does this make sense? So those are quick, fast ways to read a pedigree and so I want to talk a little bit about what we're gonna be doing with pedigrees because you should be able to reach a place where you can draw these, practice these. That is what our learning activity this block is about and we will be using some real life pedigrees as part of us. So just giving you a heads up. So now from dominant, we're gonna move to that recessive and our example for the recessive is the hemophilia. Many of the recessive conditions that we see when they are *** linked are gonna have that discrepancy that will immediately signal, huh, that clearly appears to be a recessive pattern and there's a clear delineation between males and females. Typically you do not actually designate things like a carrier with a dot, but this is one of those situations. What do you see in this picture of a pedigree, this particular pedigree? So you do see every generation affected, what does that usually indicate? Usually indicates dominance, right? That's not, but what do you, definitely see in terms of this, in terms of a discrepancy? Every affected individual is male. And so that kind of obvious difference in affected, that immediately should start to trigger that idea of, oh, this could be *** links. So then we look at mom to child and unfortunately we don't have any affected moms, but we can clearly see that there was a chance here, this is one of our really obvious groupings, affected, unaffected, unaffected, gives us that strong indication that she's a heterozygotic carrier. So when you're looking at a pedigree, it's best to look at individual relationships after you've done some of those very specific quick, is every generation affected? Do I see affected couplings having unaffected offspring? These are key things to look for. Make sense? Okay, two, two, two, two. All right, I've said this and I wanted it in writing. We don't really have monozygotic, we don't really have monogenetic traits, not really. Your genes do not exist in a vacuum and the expression of them is influenced by multiple factors. So we are having to consider in every situation, environment, lifestyle and genetic interactions, every time. Then there are of course those conditions that are truly complex and multifactorial, where you do not exhibit any of the phenotypes without additional change. Cancer being the most obvious example. A cancer predisposition syndrome is simply inheriting a risk. You are not guaranteed by your genetics to get cancer or no. Your lifestyle, your environment, additional exposures all play into your overall package of risk. Does it make sense? Some of our inherited risks can be incredibly high risks. 95% of the people with this change exhibit X, but it's still just one factor of many. Does this make sense? When it comes to a polygenic or complex trait, oftentimes what we're seeing is not one change that completely eliminates one function, but rather many more subtle changes that affect level of expression, that affect availability of multiple pathways. And it is only the interaction of all of those changes that give you the phenotype. So much like the loss of a piece of chromosome is affecting multiple genes, sometimes hundreds of genes, and we see that systemic expression as a result of that, it's a similar concept. We're losing little bits or parts or pieces or lowering the activity of multiple things and collectively that gives us our phenotype. Collectively that causes the condition. When it comes to how this works, you could have, let's think of one metabolic pathway we're really familiar with, right? Growth factor signaling. We remember this from two blocks ago. We didn't block it out as traumatic, right? No, we're good. The classic Ras-Raf-Mech-Erk, right? Okay, yes, the groans tell me that this was not a fond thing that we are being recalled to, but let's think about that. A change that reduces the activity of one of those and a change that increases the activity of the other, that could cancel out, couldn't it? But if we slowly have multiple changes that increase each step just a little, we can have drastic overall impact. We have a little bit more Ras activity. We have a little bit more Ras activity. As we move down that pathway, if each molecule exhibits a slight increase in activity, by the time we hit the end, we have a drastic increase in activity. Does that make sense? Any step in a pathway like that could be a place for activity changes. And if we add that, that's the pro or the go pathway, and we come in and we have all those regulators and we turn them off, now we've got a very active pro-growth pathway and the potential for hyperplasia. Does that make sense? I know that's the term, it just means lots of growth. We're gonna get to that in the cancer block, but I just want us to really think about this from this. Small changes to multiple things can be more than additive. It could be synergistic. You could have exponential changes in activity from a bunch of smaller changes. This is the somatic mutation theory of cancer, which we will also talk about in the later block. When it comes to linked genes or the ability to look at how two genes are relating to each other in terms of considering a phenotype, cancer, of course, being our most extreme, gazoonite, cancer being our most extreme version of this, where we have a lot of complex molecular and genetic interactions, something that's a little bit more subtle, where you need a mutation in A and B to cause your phenotype. We need to make sure that we are looking at those correlations. If you have a change in A, you may not be guaranteed to have any phenotype, but once you have a change in A and B, now you have your phenotype, we need to look at how those two pieces are being inherited. And if they are literally on the same chromosome, they are literally linked in terms of physical space, that is going to have a different implication than if they were on separate chromosomes, right? The risks of inherited together go up drastically if they're physically on the same material versus if it's completely different chromosomes. And so all of these things play into the complex picture of will I end up with a phenotype? And it of course throws off all of our Mendelian analyses. So complex diseases by their very nature are diseases caused by the combination of genetics, lifestyle, and environment. This is where I always have a little bit of fun. What does it mean when we say environment? The stuff that surrounds us, right? Well, what are you compared to a bacteria besides special and lovely? You're a multicellular, right? Your populations of cells. Sorry, yes, multicellular, yes, you are populations of cells. So does your liver live in the same environment as your brain? No, there's literally a special barrier in here, right? Somebody tell me if I'm right or not because the brain's not my area. So that's a different environment. It literally has a different biochemical potential in terms of environment. So when we talk about environment, we also mean how your lifestyle influences the physical arena in which your cells are growing. Some things you do pass the blood-brain barrier, yes? Some chemical exposures. I don't know why I'm doing this to imitate blood-brain barrier, but we'll roll with it. That is a vastly different environment than your liver, which, what's the job of the liver? It's a detox center, right? Maintain your blood glucose. Environmental changes, changes in your blood volume, changes in your blood composition. Totally different effect, right? Not to mention what we can do to red blood cells if we mess with that blood compartment. Lack of a better phrasing, I'm sorry. So when we think about environment, we really do have to consider both what the literal world around us, temperature, exposures, availability of or access to clean water. Those are environmental pieces. Do I live in a place where I run a higher risk of UV light exposure? Do I live in a place where there's a lot more smog? Those are vastly different exposures and concerns than if not, right? So environment is both internal biochemical environment we create by the choices of our external environment, and the natural environment created by your cells and their normal biochemical behavior. So environment's a weird one. And of course, lifestyle influences environment, particularly internal environment. What is a difference between a muscle for someone who exercises regularly versus someone who does not? Level of mitochondria, ability to retain and hold onto glycogen, which we'll talk about next semester. There are literal physiological changes to muscular activity that impact the environment of the muscle, the literal exposures of those cells and behavior of those cells, potential of those cells. Make sense? Environment's the tricky one. This is most human disease. You do not exist in a vacuum. Because you couldn't for the lack of oxygen, but your genes in particular are not expressed in the absence of other biochemical information. So having a gene, having a pathogenic variation is only one piece to the bigger picture. And that is why genetics fundamentally cares about molecular biology. When you have more than one gene involved, when you have more than one factor, you end up with what we call the ability for spectrums, where you can have two extremes and everything in between. Just depends on how those things are interacting, what the level of penetrance is, what the level of expressivity is, how much you've exposed yourself environmentally, what lifestyle choices you make, all of these things come into play. And the more complex, the more adult onset a condition is, the broader that spectrum becomes. Does this make sense? When we're talking about development, congenital defects are not all exactly the same. If the expected alteration or deviation is based on length, right, femur length, for example, there's a wide range of deviations that could be considered statistically significant. If we have a measurement where we have average, then it's really about how far from average do we deviate. And if there are 10 genes playing a role, this is my example, sorry, there are 10 genes playing a role in femur length, and one individual has a change in three of them, their condition could look very different than someone who has a change in 10 of them. And it would of course be dependent on what those changes were and what the developmental environment was in utero, because that's also always playing a role. A good example of environment impact is of course our spina bifida, where we have specific, I'm sorry, open neural tube defects, but spina bifida particularly as a category, specific correlation to access to folic acid. Folic acid is considered completely preventative, and we'll get into that topic later, but I just want us to get an understanding. When we are talking about this genetics piece, we are talking about wide range of possibilities. All of our adult onset. Every single person with a BRCA1 pathogenic variant has an elevated risk of breast cancer and ovarian cancer, but not every single person will develop breast or ovarian cancer. That is because there is a wide range of types of pathogenic variation and several other factors that come into play in that risk. Huntington's disease can have variable levels of effectiveness, right? Different times when you would start to exhibit symptoms. This is a dominant condition where inheriting the genetic information is a guarantee of some level of disease involvement, but the severity of that can be wide ranging. There are factors that can mitigate the outcomes, and it's not just a treatment intervention, but there are actual changes that can happen across the span of a life that can be influential. All human diseases fall into this. So when it comes to saying this is the blatant statement, we're not following Mendel. We cannot just use simple inheritance patterns. The only thing we can use Mendel's laws for is to tell us what the risk was of getting that variant. If I've got two versions of a gene, two different variations of a gene, two different alleles, what's my chance of passing one versus the other? Every time, no matter what we're talking about, whether it's male versus female, what's my chance? Well, I guess in my chance, I can only pass an X, so 100% for an X, but what do we mean? What's one and two every single time? And so when we're looking at this, that's our starting place. You have a one and two chance of this risk, unless I am what? Homozygous for that change, in which case it's 100% chance of passing that. That's our first piece is just what can parent to child look like in terms of inheritance? What's the possibility if it were a simple land? And then it becomes about all of the other factors that go into life. So the risk assessments that come from things like cancer, unclear inheritance of polygenic traits, it becomes about what is the chance you had this pattern? And if you have clear cut 50-50, you can do that math of one and a half times one and a half times one and a half, right? And get to a number, but most of these risks are gonna be ranges. You have an elevated 50 to 60% chance. You have a two to 3% chance. Does this make sense? We start to identify risks based on probabilities of the population. If you had this factor plus this factor plus this factor, how do those three together handle risk? And so many of our cancer risks assessments are really complicated questionnaires with a wide range of how that answer influences your overall risk. And we'll get into that when we get to cancer. All of these are polygenic. Everything that we're going to be talking about in terms of the more complicated pieces that don't fit this perfect picture, lots of time on cancer, lots of time on congenital anomalies. That particular area, that wide spectrum of outcomes we're gonna get is going to be about complex interactions. The problem with all of this is you can have de novo mutation. Something new that is never seen before in a family. Genetics when it is new is very different from genetics when it's familial. And as we said with our rearrangements, if something is present within a family, it does imply a potential for viability. Something that is new can be more catastrophic. Because the family having means that there are people with it throughout the family and it's fine. When we have affected individuals being born to unaffected individuals, this is what we call truly de novo. There is no family history. It's not a recessive trait suddenly appearing, but literally there's no clear pattern in the multiple generations we're studying. That is a true de novo. That being said, the de novo that you observe doesn't have to be completely separate from other things that are inherited. So teasing this out is really about what am I looking at now and how is that going to influence the future? So I have something that's new. Is it in the same realm of something we've seen before? Is it completely new? Do I have no information on this gene whatsoever in this entire disorder? Well, that's gonna be identifying a new link between a gene and a particular risk. And this is how we identified many of our moderate penetrance genes. We saw potential for correlations and then just tried to find families to see if it held up. Every year we get more information on this. Every year we find out about new changes or different pathogenic variants that correlate with specific phenotypes from congenital anomalies through cancer every single year. This is a field that is constantly evolving. So why do we still, yeah, go for it. Can you still be considered de novo if the parents are the carriers and the patient is the first incidence? Only if the generation of it came from parents having a gametogenic event. Then it's, but if being a carrier and it's just the first time that two carriers came together and had that 25% chance, that's not truly de novo. It might be new to the family, but it's not truly de novo in terms of mutation development. Does that make sense? So why do we study single gene disorder still is where I was going with that? Because those more simple inheritance patterns can give us a lot more information about the more complex ones. Identifying more clean, clear cut relationships gives us a much better picture of when we deviate from clear cut. It also gives us the concept of being able to look at what we call associated genes. I have this definitive phenotype of X, but when I have X, sometimes I also have Y. Can give us a better picture of what Y is even doing. And many of our advancements in understanding how certain genes were playing in development, especially for some of our deletion syndromes, this information really helped with that. I see this condition when I have this, and when I also lose this, it seems to correlate with this more severe version. Huh, what happens if I just lose that? And oh, look, now we can understand this new phenotype we hadn't really thought about before. Remember, a lot of what we know is based on who walked in the clinic and who we were able to study. Now we're at a place where we're doing more microarrays, more sequencing of anyone who comes in with a tumor, right? Anyone who comes in with an unclear pattern of inheritance, pediatric cases get microarray as standard practice now, whereas 10 years ago they weren't. We didn't have panels that we could quickly do to study specific gene variants in breast cancer until like 10 years ago. My sense of time is a little off, it might be a little bit longer than 10 years at this point where we had panels. But we can run RNA transcriptome analysis very easily and very inexpensively on every tumor that walks into a clinic. That gives you a tremendous capacity to study and treat. And it also helped us see where a full risk existed and where partial risks existed, where we had association of one gene to another and we were better able to link specific phenotypes with outcomes. So there's my cancer. All final products, all final outcomes are the result of complex interactions. You can mitigate risk. You can also increase it. So this is my little spiel I did before and so I put it in words for you. Environment is the most complex piece because when you are talking about people, we have two things we have to think about. What environment is the person in and what are the different biochemical environments that the cell populations are in? And how would those different biochemical environments influence those cell types? Make sense? All right, so that is our complex inheritance piece and we're gonna go back to regulation. We're gonna take a quick little side step.