Biomolecules: Water, Carbohydrates and Lipids PDF
Document Details
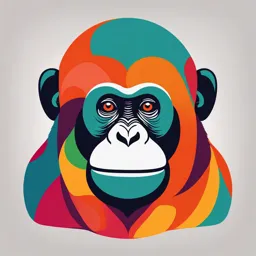
Uploaded by AwesomeNephrite1580
NEIS - Port Said
Tags
Summary
This document provides detailed information on water, carbohydrates, and lipids. It explores the properties of water and its importance in living organisms, describes the functions of different types of carbohydrates, and explains their chemical structures. The document is likely a study guide or textbook.
Full Transcript
BIOMOLECULES WATER, CARBOHYDRATES AND LIPIDS NEIS-PORT SAID PROSPERITIES OF WATER Water is a polar solvent. Water is regarded as the ‘general solvent’ or ‘universal solvent’ due to the polarity of its molecules. For example, when sodium chloride (NaCl) dissolves in water, it produ...
BIOMOLECULES WATER, CARBOHYDRATES AND LIPIDS NEIS-PORT SAID PROSPERITIES OF WATER Water is a polar solvent. Water is regarded as the ‘general solvent’ or ‘universal solvent’ due to the polarity of its molecules. For example, when sodium chloride (NaCl) dissolves in water, it produces positive sodium ions and negative chlorine ions. The positive oxygen atoms in water attract the negative chlorine ions, and the negative hydrogen atoms attract the positive sodium ions. Water has the ability to ionize molecules, which are necessary for life. For example, the pancreas secretes sodium bicarbonate (NaHCO3). This compound ionizes in water into positive hydrogen ions and negative bicarbonate ions, which makes the medium alkaline and thus suitable for the enzymes’ work. PROSPERITIES OF WATER Water has high specific heat. Specific heat is the amount of heat required to increase the temperature of one gram of matter by 1 degree Celsius. Water has the highest specific heat on Earth due to the hydrogen bonds between its molecules. As a result of having high specific heat, water needs a great amount of energy to increase its temperature and loses a great amount of energy when its temperature decreases. This helps living organisms to have a constant temperature which is essential for the vital processes occurring within their bodies. Water has low viscosity and high surface tension. Surface tension is the cohesion of the molecules on the surface of a fluid to occupy the least possible volume. Viscosity is the resistance of a fluid to flowing. Water has low viscosity and high surface tension due to the hydrogen bonds between its molecules; these conditions are suitable for life. PROSPERITIES OF WATER Water density decreases under 4◦C. Water expands when its temperature becomes less than 4◦C (instead of shrinking). This decreases its density and makes it float. In frozen lakes, we find ice on the surface, while we find liquid water underneath. This property is because of the hydrogen bonds between water molecules. The freezing point of water decreases if it has substances dissolved in it. This property is very important for living organisms, as it prevents the water in the cells of from freezing when exposed to temperatures less than 0◦C. PROSPERITIES OF WATER Water can turn into vapor in temperatures lower than boiling point (100◦C). Water vapor formed on the surfaces of oceans is carried by convection currents to cold layers in the atmosphere. This changes into clouds which provide living organisms with rain and water. Water rise in capillary tubes. Water has the ability to rise in capillary tubes without being pumped and in opposition to external forces such as gravity. This property helps water transport from trees’ roots to all of its parts. Melting: Solid matter changes its state to liquid. Freezing: Opposite process of melting is called freezing. Liquid matter loses heat and changes its state to solid. Boiling: Liquid matters gain heat and change their states to gas. Condensation: Opposite process of boiling is called condensation. Gas molecules lose heat and change its phase to liquid. During phase change, temperature of matters stay constant. Graphs of phase change are given below. Water importance to living organisms. 1. It is an essential constituent of living cell. No living thing can resist drying. 2. By its Solvent Action. 3. It Acts as a Medium for Various Physical Processes. 4. Hydrolysis. 5. Dehydration and Condensation. 6. Ionizing Medium. 7. It Acts as a Vehicle for Various Physiological Processes. 8. Heat Regulation. 9. Respiratory Function. Functions of Carbohydrates Carbohydrates along with being the chief energy source, in many animals, they are instant source of energy. Glucose is broken down by glycolysis/ Krebs’s cycle to yield ATP. Serve as energy stores, fuels, and metabolic intermediates. It is stored as glycogen in animals and starch in plants. Stored carbohydrates acts as energy source instead of proteins. They form structural and protective components, like in cell wall of plants and microorganisms. Structural elements in the cell walls of bacteria (peptidoglycan), plants (cellulose) and animals (chitin). Carbohydrates are intermediates in biosynthesis of fats and proteins. Carbohydrates aid in regulation of nerve tissue and is the energy source for brain. Carbohydrates gets associated with lipids and proteins to form surface antigens, receptor molecules, vitamins and antibiotics. Formation of the structural framework of RNA and DNA (ribonucleic acid and deoxyribonucleic acid). They are linked to many proteins and lipids. Such linked carbohydrates are important in cell-cell communication and in interactions between cells and other elements in the cellular environment. Carbohydrates those are rich in fiber content help to prevent constipation. Chemical Formula of Sugar The sugar formula consists of the C (i.e., carbon), H (i.e., hydrogen), and O (i.e., Oxygen) atoms. The General Chemical formula of sugar is Cn H2n On where “n” refers to the number of atoms of that element present in the carbohydrate. In the straight-chain form, the carbon atoms are linked in a linear fashion, while in the cyclic form, they are connected to form a ring, which is more common in nature. Sugars are also named according to their number of carbons: some of the most common types are trioses (three carbons), pentose (five carbons), and hexoses (six carbons). The “D” represents the configuration of the hydroxy-group on the fifth carbon atom in the molecule, if glucose is drawn as a Fisher projection. Specifically, the hydroxy-sidechain will be on the right-hand-side of the projection. Ring forms of sugars The sole chemical difference between alpha and beta glucose is the orientation of the -OH (hydroxyl) and -H (hydrogen) groups on carbon 1. Isomers One of two or more compounds that have the same chemical formula but different arrangements of the atoms within the molecules and that may have different physical/chemical properties. Disaccharides Disaccharides (di- = “two”) form when two monosaccharides join together via a dehydration reaction, also known as a condensation reaction or dehydration synthesis. In this process, the hydroxyl group of one monosaccharide combines with the hydrogen of another, releasing a molecule of water and forming a covalent bond known as a glycosidic linkage. Each carbon atom in a monosaccharide is given a number, starting with the terminal carbon closest to the carbonyl group (when the sugar is in its linear form). This numbering is shown for glucose and fructose, above Common disaccharides include lactose, maltose, and sucrose. Lactose is a disaccharide consisting of glucose and galactose and is found naturally in milk. Many people can't digest lactose as adults, resulting in lactose intolerance (which you or your friends may be all too familiar with). Maltose, or malt sugar, is a disaccharide made up of two glucose molecules. The most common disaccharide is sucrose (table sugar), which is made of glucose and fructose. Polysaccharides A long chain of monosaccharides linked by glycosidic bonds is known as a polysaccharide (poly- = “many”). The chain may be branched or unbranched and may contain different types of monosaccharides. Starch, glycogen, cellulose, and chitin are some major examples of polysaccharides important in living organisms. Storage Polysaccharides --- Starch Starch is the stored form of sugars in plants and is made up of a mixture of two polysaccharides, amylose and amylopectin (both polymers of glucose). Plants are able to synthesize glucose using light energy gathered in photosynthesis, and the excess glucose, beyond the plant’s immediate energy needs, is stored as starch in different plant parts, including roots and seeds. The starch in the seeds provides food for the embryo as it germinates and can also serve as a food source for humans and animals, who will break it down into glucose monomers using digestive enzymes. Amylose consists entirely of unbranched chains of glucose monomers connected by 1-4 linkages. Amylopectin is a branched polysaccharide. Although most of its monomers are connected by 1-4 linkages, additional 1-6 linkages occur periodically and result in branch points. Starch is the major source of energy stored as a carbohydrate in plants. It is composed of two substances: amylose, which is a linear polysaccharide, and amylopectin, which is a branched polysaccharide. Both the forms of starch are polymers of α-D-glucose. Amylose is a polysaccharide consisting of α-1,4- glycosidic bonds; amylopectin is a polysaccharide consisting of α-1,4 linkages and branch point α-1,6- glycosidic bonds that occur every 20 to 25 glucose units. Both amylose and amylopectin are polymers of glucose, they are not linked together but are separate types of molecules. Glycogen is the storage form of glucose in humans and other vertebrates and is made up of monomers of glucose. Glycogen is the animal equivalent of starch and is a highly branched molecule usually stored in liver and muscle cells. Whenever blood glucose levels decrease, glycogen is broken down to release glucose in a process known as glycogenolysis. Glycogen is structurally quite similar to amylopectin, although glycogen is more highly branched (8– 12 glucose units between branches) and the branches are shorter Structural Polysaccharides --- Cellulose Cellulose is the most abundant natural biopolymer. The cell wall of plants is mostly made of cellulose; this provides structural support to the cell. Wood and paper are mostly cellulosic in nature. Cellulose is made up of glucose monomers that are linked by β 1-4 glycosidic bonds. Every other glucose monomer in cellulose is flipped over, and the monomers are packed tightly as extended long chains. This gives cellulose its rigidity and high tensile strength—which is so important to plant cells. While the β 1-4 linkage cannot be broken down by human digestive enzymes, herbivores such as cows, koalas, buffalos, and horses are able, with the help of the specialized flora in their stomach, to digest plant material that is rich in cellulose and use it as a food source. In these animals, certain species of bacteria and protists reside in the rumen (part of the digestive system of herbivores) and secrete the enzyme cellulase. The appendix of grazing animals also contains bacteria that digest cellulose, giving it an important role in the digestive systems of ruminants. Animal source Plant source Fatty Acids Fatty acids are merely carboxylic acids with long hydrocarbon chains Ester bond Phospholipids Like triglycerides, phospholipids have a glycerol backbone. But unlike triglycerides, phospholipids only have two fatty acid molecules attached to the glycerol backbone, while the third carbon of the glycerol backbone is bonded to a phosphate group. Phospholipids are amphipathic molecules. This means that they have a hydrophilic, polar phosphate head and two hydrophobic fatty acid tails. A liposome is a synthetic spherical-shaped vesicle that is composed of one or more phospholipid bilayers, which closely resembles the structure of cell membranes. The ability of liposomes to encapsulate hydrophilic or lipophilic drugs have allowed these vesicles to become useful drug delivery systems. The Fundamental Structure of Proteins Proteins are the polymers of α (20 type) amino acids and they are connected to each other by a peptide bond or peptide linkage. The elements that make up proteins are carbon , hydrogen , oxygen as well as nitrogen. Types of R-groups Positively charged side chain. Negatively charged side chain. Polar, uncharged side chain. Hydrophobic side chain. Special cases like SH group The hydrogen bonded to the Nitrogen atom and the Carbonyl oxygen Tertiary Structure of Protein The tertiary structure of a protein consists of the way a polypeptide is formed of a complex molecular shape. This is caused by R-group interactions such as disulfide (covalent) bonds, hydrogen bonds (broken by high Temp. or adding alcohol, …..), ionic bonds (adding acid or alkali) , and hydrophobic interactions. A protein becomes denatured when its normal shape gets deformed because some of these bonds are broken. Protein quaternary structure Protein quaternary structure is the fourth classification level of protein structure. Protein quaternary structure refers to the structure of proteins which are themselves composed of two or more smaller protein chains. Collagen Collagen is fibrous protein composed of 3 chains bonded by hydrogen bonds. The chains are wound together to form a triple helix. Since glycine is the smallest of all the amino acids, it allows the chain to form a tight configuration, and and it can withstand stress. Every third amino acid is glycine. Collagen is the primary building block of your body's skin, muscles, bones, tendons and ligaments, and other connective tissues. It's also found in your organs, blood vessels and intestinal lining. The hemoglobin is a globular protein made up of four polypeptide chains: two alpha chains of 141 amino acid residues each and two beta chains of 146 amino acid residues each. each of which has a single covalently bound heme group. Each of the four heme groups is made up of an iron Fe2+. Oxygen binds to ferrous iron in heme. There is another way to group sugars as well: whether they are a reducing sugar or a non-reducing sugar. A reducing sugar is a sugar that has a free aldehyde or ketone that can act as a reducing agent. A non- reducing sugar does not have a free aldehyde or ketone, so it cannot act as a reducing agent. CELLS AND VIRUSES NIES-PORT SAID The shorter the wavelength of the light (or other form of electromagnetic radiation) being used, the better the resolution. This is because when light interacts with an object, it can either be absorbed, reflected, refracted, or diffracted. Why is a specimen placed in a vacuum in an electron microscope? Without a vacuum, the electrons would strike and be scattered by the atoms / particles of the air components. Cells are divided into two main classes, initially defined by whether they contain a nucleus. Prokaryotic cells (bacteria) lack a nuclear envelope; eukaryotic cells have a nucleus in which the genetic material is separated from the cytoplasm. Both prokaryotic and eukaryotic cells have structures in common. All cells have a plasma membrane, ribosomes, cytoplasm, and DNA. The plasma membrane, or cell membrane, is the phospholipid layer that surrounds the cell and protects it from the outside environment. Ribosomes are the non-membrane bound organelles where proteins are made, a process called protein synthesis. The cytoplasm is all the contents of the cell inside the cell membrane, not including the nucleus. Structure of a prokaryotic cell. Structure of a eukaryotic cell Prokaryotic Eukaryotic Cell size (1 – 10um) larger (10 – 100um) Cell arrangement unicellular multicellular True membrane-bound No Yes nucleus DNA structure DNA is circular and is linear and complexed with neither associated with "histones," before histones nor organized into organization into a number chromosomes. of chromosomes Membrane-bound organelles do not contain Contain membrane-bound organelles such as the mitochondria, golgi apparatus, lysosomes, peroxisomes and ER Ribosome size 70S 80S Cytoskeleton No Yes Sexual reproduction Asexually Most Sexually Cell division Binary fission Mitosis Ribosomes coordinates the interaction between mRNA and tRNA and Protect mRNA from nucleases. Cell wall (Plant cell) The plant cell wall is generally arranged in 3 layers and composed of carbohydrates, like pectin, cellulose, hemicellulose. The three major layers are: Primary Cell Wall The Middle Lamella (an interface between the other neighboring cells and glues them together) The Secondary Cell Wall The cell wall of plant cells is permeable to all substances. The main function of the cell wall is to provide structural strength and support (rigid and regular shape). It allows cells to develop turgor pressure, which is the pressure of the cell contents against the cell wall. Plasmodesmata Plasmodesmata are cytoplasmic bridges that connect adjacent plant cells. The plasmodesmata is a channel through the plant cell wall that allows molecules and substances to move in and out of the cell. Cell membrane The cell membrane consists of a lipid bilayer that is semipermeable (Selective). The cell membrane regulates the transport of materials entering and exiting the cell. Most plasma membranes consist of approximately 50% lipid and 50% protein by weight, with the carbohydrate portions of glycolipids and glycoproteins constituting 5 to 10% of the membrane mass. Endoplasmic reticulum (ER) The transportation system of the cell Endoplasmic reticulum is an organelle found in both eukaryotic animal and plant cells.. The endoplasmic reticulum (ER) is a network of membrane-enclosed tubules and sacs (cisternae) that extends from the nuclear membrane throughout the cytoplasm. There are two distinct sub-compartments of the ER – the rough and the smooth ER. SER RER Structure Tubular structure Flat sacs which are and does not have embedded with ribosomes on its membrane-bound surface ribosomes on the outer surface Function Synthesis and Synthesis and transport lipids. transport proteins to Golgi or other parts and may in vesicles. Golgi apparatus, Golgi body, is a membrane-bound organelle found in eukaryotic cells (cells with clearly defined nuclei) that is made up of a series of flattened stacked pouches called cisternae. It is located in the cytoplasm next to the endoplasmic reticulum and near the cell nucleus. The Golgi apparatus, or Golgi complex, functions as a factory in which proteins received from the ER are further processed and sorted for transport to their eventual destinations: lysosomes, the plasma membrane, or secretion. The Golgi apparatus is responsible for transporting, modifying, and packaging proteins and lipids into vesicles for delivery to targeted destinations. Lysosome Lysosomes are sphere-shaped sacs filled with hydrolytic enzymes that have the capability to break down many types of biomolecules. Enzymes of the lysosomes are synthesized in the rough endoplasmic reticulum and exported to the Golgi apparatus transported from the Golgi apparatus to lysosomes in small vesicles, which fuse with larger acidic vesicles What are the functions of lysosome? 1. Intracellular digestion 2. Removal of dead cells (autolysis) 3. Destruction of old organelles (autophagy) 4. Help in fertilization (sperms head or acrosome secrete some lysosomal enzymes which help in the penetration of sperm into vitelline layer of ovum). Mitochondria Mitochondria are surrounded by a double-membrane system, consisting of inner and outer mitochondrial membranes separated by an intermembrane space. The inner membrane forms numerous folds (cristae), which extend into the interior (or matrix) of the organelle and has highly selective permeability. Containing DNA and ribosomes. Chloroplast They are double-membrane organelle with the presence of outer, inner and intermembrane space. The inner membrane surrounds a large space called the stroma, which is analogous to the mitochondrial matrix and contains many metabolic enzymes. Stroma is the fluid-filled internal space of the chloroplasts which encircle the grana and the thylakoids composed of membranes that contain photosynthetic chlorophyll. like the mitochondrion, the chloroplast has its own Chloroplasts produce energy through photosynthesis genome and genetic system. The stroma therefore and oxygen-release processes, which sustain plant growth and crop yield. As such, chloroplasts are also contains a special set of ribosomes, RNAs, and responsible for the biosynthesis of active compounds the chloroplast DNA. such as amino acids, phytohormones, nucleotides, vitamins, lipids, and secondary metabolites Ribosomes Ribosomes can be found floating within the cytoplasm or attached to the endoplasmic reticulum. A ribosome is made out of RNA and proteins, and each ribosome consists of two separate RNA-protein complexes, known as the small and large subunits. Ribosomes receive information from the cell nucleus and construction materials from the cytoplasm. Ribosomes translate information encoded in messenger ribonucleic acid (mRNA). They link together specific amino acids to form polypeptides and they export these to the cytoplasm. (Protein synthesis) Nucleus The nuclear envelope is made up of two lipid bilayer membranes, an inner nuclear membrane and an outer nuclear membrane. These membranes are connected to each other by nuclear pores. passage of substances). The outer nuclear membrane is continuous with the endoplasmic reticulum. The nucleoplasm is a gel-like substance inside the nucleus of a cell. The primary components of the nucleoplasm are chromatin, protein fibers called fibrils, and water. Chromatin is made from DNA and protein molecules that code genetic information for protein synthesis. The nucleus houses the nucleolus. The nucleolus is not membrane bound synthesizes the parts that make up the ribosomes. Centrioles Centrosomes are structures found inside of animal cells. They are made from two centrioles. Centrioles are microtubule rings. Each centriole is a cylinder of microtubules, typically consisting of a ring of 27 microtubules (arranged as 9 triplets). The main purpose of a centrosome is to organize microtubules and provide structure for the cell, as well as work to pull chromatids apart during cell division. Microtubules Microtubules are composed of alpha- and beta-tubulin subunits assembled into linear protofilaments. A single microtubule contains 10 to 15 protofilaments (13 in mammalian cells) that wind together to form a wide hollow cylinder. The microtubule-organizing center (MTOC) is a structure found in eukaryotic cells from which microtubules emerge. MTOCs have two main functions: the organization of eukaryotic flagella and cilia and the organization of the mitotic and meiotic spindle apparatus, which separate the chromosomes during cell division. Sap Vacuoles Sap vacuoles are little sac-like structures found in cells that are filled with fluid and used for material storage (cell sap). It creates a sealed compartment within the cell that is filled with water, dissolved inorganic and organic molecules (enzymes). Their job is to store resources and sustain the cell mechanically. It also keeps the cell's Turgor pressure constant. Microvilli Microvilli are tiny finger-like projections found on the surface of certain cells. Their purpose is to increase the surface area of the cell's apical surface, resulting in more effective absorption or secretion of substances. These microvilli are predominantly present in cells lining the small intestine, kidney tubules, and certain cells in the respiratory and reproductive systems. By significantly expanding the surface area of the cell membrane, microvilli enhance the cell's capacity to exchange materials with its surroundings. CELL MEMBRANES NEIS – PORT SAID Cell membrane vs Plasma membrane The plasma membrane, also called the Some main differences between the cell membrane, is the membrane two are the fact that the plasma found in all cells that separates the membrane encloses the organelles, interior of the cell from the outside whereas the cell membrane encloses environment. In bacterial and plant the entire cell. Moreover, the cell cells, a cell wall is attached to the membrane can protect the cell from plasma membrane on its outside outside viruses and bacteria, the surface. plasma membrane, however, cannot do this. The fluid mosaic structure of the membrane The fluid mosaic model describes the structure of the plasma membrane as a mosaic of components —including phospholipids, cholesterol, proteins, and carbohydrates—that gives the membrane a fluid character Cell membrane fluidity (CMF) is describing the freedom of movement of protein and lipid constituents within the cell membrane. The following is a principle of the fluid mosaic model of cell membrane structure: Phospholipids form a bilayer that contains proteins that change position in this bilayer. The fluid mosaic structure of the membrane Factors influence cell membrane fluidity Lipids containing unsaturated fatty acids similarly increase 1. Temperature, membrane fluidity because the presence of double bonds 2. Cholesterol, introduces kinks in the fatty acid chains, making them more difficult to pack together. 3. Kind of fatty acids in the phospholipids It is predicted that anything that will increase membrane viscosity will decrease fluidity. Examples include, decreasing temperature, increasing pressure, increasing cholesterol content, and altering environmental pH and ionic strength. The fluid mosaic structure of the membrane The fluid mosaic model was first proposed by S.J. Singer and Garth L. Nicolson in 1972 to explain the structure of the plasma membrane. Plasma membranes range from 5 to 10 nm in thickness. For comparison, human red blood cells, visible via light microscopy, are approximately 8 µm wide, or approximately 1,000 times wider than a plasma membrane. The proportions of proteins, lipids, and carbohydrates in the plasma membrane vary with cell type. The fluid mosaic structure of the membrane Phospholipid approximately 50% of the mass of most cell membranes 1. amphiphilic or dual-loving, phospholipid molecules. The hydrophilic (head) or water-loving areas of these molecules are in contact with the aqueous fluid both inside and outside the cell. 2. A phospholipid molecule consists of a three-carbon glycerol backbone with two fatty acid molecules attached to carbons 1 and 2, and a phosphate-containing group attached to the third carbon. This arrangement gives the overall molecule an area described as its head (hydrophilic) (the phosphate-containing group), which has a polar character or negative charge, and an area called the tail (the fatty acids), which has no charge (hydrophobic). The fluid mosaic structure of the membrane Phospholipid 3. The hydrophilic regions of the phospholipids tend to form hydrogen bonds with water and other polar molecules on both the exterior and interior of the cell. 4.. In contrast, the middle of the cell membrane is hydrophobic and will not interact with water. Therefore, phospholipids form an excellent lipid bilayer cell membrane that separates watery media or fluid within the cell from the fluid outside of the cell. The fluid mosaic structure of the membrane Phospholipid --- Importance The lipid bilayer acts as a barrier to the passage of molecules and ions into and out of the cell. However, an important function of the cell membrane is to allow selective passage of certain substances into and out of cells.. The fluid mosaic structure of the membrane Cholesterol Cholesterol is a steroidal lipid that is present in all animal tissues and plays important roles in membrane structure, lipid metabolism, and the production of bile acids, vitamin D, and steroid hormones. Cholesterol is situated in the cell membrane in between the phospholipids. The rigid rings of the cholesterol molecule interact with the hydrophobic tails of the phospholipids. The higher the cholesterol content in membranes, the lower its fluidity, and vice versa. Provide structure and support to the membrane. Acts as a permeability barrier for the membrane. The fluid mosaic structure of the membrane Proteins Proteins make up the second major component of plasma membranes. 50% ?? 1. Integral proteins (some specialized types are called integrins) are, as their name suggests, integrated completely into the membrane structure, and this type of protein has a hydrophilic region or regions, and one or several mildly hydrophobic regions. This arrangement of regions of the protein tends to orient the protein alongside the phospholipids, with the hydrophobic region of the protein adjacent to the tails of the phospholipids and the hydrophilic region or regions of the protein protruding from the membrane and in contact with the cytosol or extracellular fluid. The fluid mosaic structure of the membrane Proteins Proteins make up the second major component of plasma membranes. 2. Peripheral proteins are not embedded in the phospholipid bilayer and do not extend into its hydrophobic core. They are proteins that associate directly with the lipid membrane (electrostatic interactions) or indirectly via interactions with integral membrane proteins.The peripheral membrane proteins function to conduct and help maintain the cytoskeleton (attached to protein tubules) of the cell, as well as contain the extracellular matrix of the tissue. The fluid mosaic structure of the membrane Proteins -- functions transport enzymatic activity triggering signals attachment (anchoring the cell in a particular location) recognition of molecules (receptors) cytoskeleton The fluid mosaic structure of the membrane Carbohydrates Carbohydrates are the third major component of plasma membranes. They are always found on the exterior surface of cells and are bound either to proteins (forming glycoproteins) or to lipids (forming glycolipids). These carbohydrate chains may consist of 2–60 monosaccharide units and may be either straight or branched. The fluid mosaic structure of the membrane Carbohydrates They enable cells to recognize another cell as familiar or foreign, which is called cell-cell recognition. They also help cells attach to and bind other cells, which is called cell adhesion. Involved in the fine tuning of cell signalling as reaction molecules in the front line to various extrinsic stimulants. Transport across the membrane Passive transport across cell membranes A. Diffusion 1. Simple Diffusion Molecules and ions move freely in gases and liquids, each type of these particles tends to spread out evenly within the space available. Some molecules and ions are able to pass through cell membranes --> The membrane is permeable. Some substances cannot pass through cell membranes --> The membrane is partially permeable. faster than simple diffusion Water can pass through biological membranes via two pathways: simple diffusion through the lipid bilayer, or water-selective facilitated diffusion through aquaporins (AQPs). In human kidneys, nine aquaporins (AQPs), including AQP1–8 and AQP11, have been found and are differentially expressed along the renal tubules and collecting ducts with distinct and critical roles in the regulation of body water homeostasis and urine concentration. Passive transport across cell membranes ---B. Osmosis Diluted solution (R) has a higher water potential (tendency of water to move) and a concentrated solution (L) has a low water potential. Passive transport across cell membranes ---B. Osmosis Passive transport across cell membranes ---B. Osmosis In a hypotonic solution, the extracellular fluid has a higher water potential (low osmolarity) than the fluid inside the cell so water enters the cell. In a hypertonic solution, the extracellular fluid has a lower water potential (higher osmolarity) than the fluid inside; water leaves the cell. In an isotonic solution the extracellular fluid has the same water potential as the cell; there will be net water movement into or out the cell. Osmosis & plant cell Osmosis & plant cell If a plant cell is placed in high water potential or a dilute solution, water will enter the plant cell through its partially permeable cell surface membrane by osmosis, as the dilute solution has a higher water potential than the plant cell As water enters the vacuole of the plant cell, the volume of the plant cell increases (turgor pressure). The expanding protoplast (living part of the cell inside the cell wall) pushes against the cell wall and pressure builds up inside the cell – the inelastic cell wall prevents the cell from bursting. The pressure created by the cell wall also stops too much water entering and this also helps to prevent the cell from bursting When a plant cell is fully inflated with water and has become rigid and firm, it is described as fully turgid This turgidity is important for plants as the effect of all the cells in a plant being firm is to provide support and strength for the plant – making the plant stand upright with its leaves held out to catch sunlight If plants do not receive enough water the cells cannot remain rigid and firm (turgid) and the plant wilts Osmosis & plant cell If a plant cell is placed in a solution with a lower water potential than the plant cell (such as a concentrated sucrose solution), water will leave the plant cell through its partially permeable cell surface membrane by osmosis As water leaves the vacuole of the plant cell, the volume of the plant cell decreases The protoplast gradually shrinks and no longer exerts pressure on the cell wall As the protoplast continues to shrink, it begins to pull away from the cell wall This process is known as plasmolysis – the plant cell is plasmolysed. What are the factors affecting the rate of Osmosis? The factors affecting the rate of osmosis include: 1.Pressure. 2.Temperature. 3.Surface Area. 4.Water Potential. 5.Concentration gradient. Water Potential (Ψ) Water Potential (Ψ) = zero in pure water ΨS = solute potential ΨP = pressure potential Psi Ψ = ΨS + ΨP The formula for calculating water potential If the solute concentration of a solution increases, the potential for the water in that solution to undergo osmosis decreases to be negative and zero in pure water. Solute potential (Ψs) decreases with increasing solute concentration; a decrease in Ψs causes a decrease in the total water potential. Solutes reduce water potential (resulting in a negative Ψw) by consuming some of the potential energy available in the water. Solute molecules can dissolve in water because water molecules can bind to them via hydrogen bonds 100% pure water has Water Potential (Ψ) Ψ = 0, which is the highest possible water ΨS = solute potential potential, so all solutions ΨP = pressure potential Psi Ψ = ΨS + ΨP have Ψ < 0, and you cannot get Ψ > 0 The formula for calculating water potential The higher the pressure potential (Ψp), the more potential energy in a system: a positive Ψp increases Ψtotal, while a negative Ψp decreases Ψtotal. If water enters into a cell, hydrostatic pressure develops and the cell becomes turgid. This magnitude of increase in water potential in a turgid cell is called pressure potential. In the plasmolyzed cell, plant cell loses water, pressure potential lowers and water potential then becomes equal to osmotic potential. (negative). The Solute potential is negative and pressure potential is positive. In a fully turgid cell, the solute potential is equal to pressure potential and consequently water potential is zero. II. Active transport across cell membranes II. Active transport across cell membranes The active transport is done using membrane (transporter) proteins / pumps in the cell membrane. These use energy (this energy conform the protein to help in transport) from the breakdown of ATP to move the ions into the cell. The carrier proteins are ATPases. Each carrier protein is specific to just one type of ion or molecule. Cells contain many different carrier proteins in their membranes. II. Active transport across cell membranes Active transport is not the same as facilitated diffusion. Both active transport and facilitated diffusion do use proteins to assist in transport. However, active transport works against the concentration gradient, moving substances from areas of low concentration to areas of high concentration. In addition, the types of proteins that they use are different. (See below.) Active transport uses carrier proteins, not channel proteins. These carrier proteins are different than the ones seen in facilitated diffusion, as they need ATP in order to change conformation. Channel proteins are not used in active transport because substances can only move through them along the concentration gradient. III. Endocytosis and exocytosis (bulk transport) Macromolecules are too large to move with membrane proteins and must be transported across membranes in vesicles. Exocytosis the transport of macromolecules out of a cell in a vesicle the object is surrounded by a membrane inside the cell to form a vesicle the vesicle is moved to the cell membrane. the membrane of the vesicle fuses with the cell membrane, expelling its contents outside the cell. III. Endocytosis and exocytosis (bulk transport) Endocytosis the transport of macromolecules into a cell in a vesicle the cell puts out extensions around the object (infolding of the plasma membrane) to be engulfed. the membrane fuses together around the object, forming a vesicle. there are 2 types of endocytosis: phagocytosis (cell eating) and pinocytosis (cell drinking). GAS EXCHANGE NEIS – PORT SAID 24/25 Gas exchange In all organisms gaseous exchange, the supply of oxygen to and removal of carbon dioxide from cells, depends ultimately on the rate at which these gases diffuse in the dissolved state. The area of a respiratory surface is directly proportional to the rate of gas exchange across that surface. That is, as the surface area increases, the rate of gas exchange also increases. This is because a greater surface area allows more gas particles to cross per unit of time. In human, during gas exchange oxygen moves from the lungs to the bloodstream. At the same time carbon dioxide passes from the blood to the lungs. This happens in the lungs between the alveoli and a network of tiny blood vessels called capillaries, which are located in the walls of the alveoli. Features of Gas Exchange Surfaces The surfaces where gas exchange occurs in an organism are highly varied Different organisms have evolved mechanisms for getting the gases to the gas exchange surface depending on size, habitat etc. All gas exchange surfaces have features in common These features allow the maximum number of molecules to be exchanged across the surface in the shortest period of time They include: Large surface area to allow faster diffusion of gases across the surface Thin walls to ensure diffusion distances remain short Good ventilation with air so that diffusion gradients can be maintained Good blood supply to maintain a high concentration gradient so diffusion occurs faster Methods of respiration Methods of respiration Respire through the plasma membrane The unicellular organism such as amoeba takes oxygen from air, water, and surrounding with the help of the surface of the cells. The animals take oxygen and then diffuse that oxygen into energy and carbon dioxide. The generated carbon dioxide is exhaled by the use of a plasma membrane. Methods of respiration Through skin Some animals such as tapeworms and earthworms use their skin for the respiration process. Furthermore, because their skins are very thin, soft, and moistened which helps in exchanging gases. The skin of the animals contains many blood cells that are spread on their skin and the blood cells present are known as capillaries that help in the respiration process. Methods of respiration Tracheal system The body of insects contains a special fine tube for the exchange of gases known as the trachea that helps in the respiration process of the insects. The air containing oxygen enters into the trachea and reaches the trachea tube that sends the oxygen to cells of the body that helps the insects in breaking their food. Methods of respiration Through gills Aquatic animals take oxygen with the help of gills. Gills are the special muscles provided in the aquatic animals that extract the dissolved oxygen from the water and send it to the cells of the aquatic animals. Methods of respiration Through lungs Respiration in animals such as humans occurs with the help of the lungs. The animals take oxygen from the air by using their lungs that send the usable oxygen to the cells of the animals to break down the taken food to obtain the energy. The provided oxygen is mixed with the glucose that gives sugar, water, and energy that gives strength for working. The lungs are the body part of humans that is used to extract oxygen from the air and exhale carbon dioxide. The lungs are present in the chest cavity and work as a filter in the human body for the extraction of oxygen. Human Gas Exchange Respiratory tree (multiple-branched bronchi) The trachea branches into the right and left primary bronchi at the carina. The bronchi continue to branch into bronchial a tree. The main function of the bronchi, like other conducting zone structures, is to provide a passageway for air to move into and out of each lung. In addition, the mucous membrane traps debris and pathogens. A bronchiole branches from the tertiary bronchi. The terminal bronchioles join a respiratory bronchiole, the smallest type of bronchiole, which then leads to an alveolar duct, opening into a cluster of alveoli. An alveolus is one of the many small, grape-like sacs that are attached to the alveolar ducts. CAPILLARIES are blood vessels in the walls of the alveoli. The walls of the alveoli share a membrane with the capillaries. That's how close they are. This lets oxygen and carbon dioxide diffuse Transfer of oxygen Gas exchange takes place in the millions of alveoli in the lungs and the capillaries that envelop them. As shown below, inhaled oxygen moves from the alveoli to the blood in the capillaries, and carbon dioxide moves from the blood in the capillaries to the air in the alveoli. Three processes are essential for the transfer of oxygen from the outside air to the blood flowing through the lungs: ventilation, diffusion, and perfusion. Ventilation is the process by which air moves in and out of the lungs. Diffusion is the spontaneous movement of gases, without the use of any energy or effort by the body, between the alveoli and the capillaries in the lungs. Perfusion is the process by which the cardiovascular system pumps blood throughout the lungs. Specialized cells and structures What are the 4 histological layers of the trachea? respiratory mucosa, submucosa, cartilage and/or muscular layer 1- mucosa The tracheal mucosa is composed of ciliated pseudostratified columnar and goblet cells. Goblet cells produce mucus that contains immunoglobulin A (IgA), lysozymes, lactoferrin, and peroxidases.The cilia function to propel the dust away from the lung. Specialized cells and structures What are the 4 histological layers of the trachea? respiratory mucosa, submucosa, cartilage and/or muscular layer 2- submucosa The second histological layer is the submucosa. It consists of connective tissue that contains mucus glands, smooth muscle, vessels, nerves and lymphatics 3- cartilage Trachea is made up of 16 to 20 rings of cartilage. C-shaped cartilaginous rings provide a rigid structure to the trachea. This rigidity prevents the trachea from collapsing during gas exchange and keeps the airways open. Because these rings are C-shaped, it allows the trachea to adapt to the changes in the esophagus during swallowing The respiratory mucosa and submucosa are 4- muscular layer adapted to warm and moisten the air, and to trap particles in mucous. The trachealis smooth muscle found in the posterior wall allows the trachea to contract and decrease its diameter. Bronchioles feature sparsely ciliated simple columnar epithelium and secretory club cells (Clara) but lack cartilage, goblet cells, and glands. Fewer cilia and the diameter of bronchioles ranges from 0.5 mm to 1 mm. Adaptations of the alveoli Thin walls - alveolar walls are one cell thick providing gases with a short diffusion distance. Moist walls - gases dissolve in the moisture helping them to pass across the gas exchange surface. Permeable walls - allow gases to pass through. They give the lungs a really big surface area. Extensive blood supply: They have a lot of tiny blood vessels called capillaries. It ensures that the blood rich in oxygen is carried away from the lungs and the blood rich in carbon dioxide is carried to the lungs. Large diffusion gradient: Breathing guarantees that the concentration of oxygen in the alveoli is greater than the concentration in capillaries to ensure the movement of oxygen from the alveoli to the blood. The diffusion of carbon dioxide occurs in the reverse direction. The elastic fibers allow the alveoli to stretch as they are filled with air during inhalation. They then spring back during exhalation in order to expel the carbon dioxide-rich air. Alveolar macrophages play an important role in scavenging microbes. The rate of gas diffusion The rate of gas diffusion through the alveolar-capillary membrane is determined by several factors, including (1) the pressure difference of each gas between both sides of the membrane, (2) the solubility of the gas, (3) the surface area of the membrane, (4) the distance through which the gas must diffuse, and (5) the molecular weight of the gas Solubility and molecular weight are two important factors that determine the diffusion coefficient of a gas. If the diffusion coefficient for oxygen is 1, the relative diffusion coefficients for different gases in the body fluid are as follows: carbon dioxide, 20.3; carbon monoxide, 0.81; nitrogen, 0.53; and helium, 0.95. Therefore, carbon dioxide diffuses more rapidly than oxygen across membranes. Gas exchange between the air in the alveoli and the blood For effective gas exchange to occur, alveoli must be ventilated and perfused. Ventilation (V) refers to the flow of air into and out of the alveoli, while perfusion (Q) refers to the flow of blood to alveolar capillaries. Gas exchange in the alveoli occurs primarily by diffusion. Traveling from the alveoli to capillary blood, gases must pass through alveolar surfactant, alveolar epithelium, basement membrane, and capillary endothelium. Oxygen. alveolar epithelium. Hb. Cross cell capillary endothelium membrane RBCs Effects of tar and carcinogens in tobacco smoke on the gas exchange system There are many harmful chemicals in cigarettes that are linked to disease, e.g. nicotine: narrows blood vessels and increases heart rate, leading to increased blood pressure causes high blood pressure that leads to blood clots forming in the arteries, potentially resulting in heart attack or stroke carbon monoxide: binds irreversibly to haemoglobin, reducing the capacity of blood to carry oxygen puts more strain on the breathing system, as breathing frequency and depth need to increase to supply the same amount of oxygen means that the circulatory system needs to pump blood faster, raising blood pressure and increasing the risk of coronary heart disease and stroke tar: is a carcinogen linked to increased chances of cancerous cells developing in the lungs contributes to COPD, which occurs when chronic bronchitis and emphysema occur together Effects of tar and carcinogens in tobacco smoke on the gas exchange system tar: is a carcinogen linked to increased chances of lung cancer. contributes to COPD, which occurs when chronic bronchitis and emphysema occur together. Chronic obstructive pulmonary disease (COPD) is a common lung disease causing restricted airflow and breathing problems. It is sometimes called emphysema or chronic bronchitis. In people with COPD, the lungs can get damaged or clogged with phlegm. lung cancer Lung cancer occurs when gene mutations cause unusual cell growth in the lungs. Smoking is the number one cause of lung cancer. Lung cancer starts in the trachea, the main airway (bronchus) or the lung tissue. Cancer that starts in the lung is called primary lung cancer. Metastatic lung cancer means that the cancer has spread from where it started in the lung to other parts of the body. Lung cancer doesn't always cause symptoms in its early stages. Symptoms can include a cough that won't go away, coughing up blood and breathlessness. Lung cancer diagnosis often starts with an imaging test to look at the lungs. chest x-ray, CT scan and PET-CT scan to diagnose lung cancer. You might also have a bronchoscopy and biopsy. Emphysema Emphysema is a lung disease that results from damage to the walls of the alveoli in lungs, leading to fewer and larger air sacs instead of many tiny ones which become inflamed. This reduces the surface area of the lungs and, in turn, the amount of oxygen that reaches your bloodstream. This makes it harder for your lungs to move oxygen in and carbon dioxide out. This causes the air sacs to lose their shape and become less elastic as a result of destruction of elastin (inflammatory phagocytes enzymes) , or abnormalities in elastic fiber assembly causing the bronchioles to collapse and loss of lung elastic recoil leads to difficulty in exhalation it makes it hard to get fresh air in and out of your lungs. This reduces the surface area of the lungs and, in turn, the amount of oxygen that reaches your bloodstream. causing the bronchioles to collapse and loss of lung elastic recoil leads to difficulty in exhalation less elastic as a result of destruction of elastin Chronic bronchitis Chronic bronchitis is thought to be caused by overproduction and hypersecretion of mucus by goblet cells, mucus trap bacteria which inflame and then swell the airways linings combined with poor ciliary function. The airways become clogged also by debris and this further increases the irritation. Debris blocks the bronchioles leading to difficulty in breathing and no or less gas exchange. The smooth muscle in the airway becomes thicker and narrows the breathing tubes (airways). Exhaust Gases Diesel engine exhaust gases contain several harmful substances. The main pollutants are carbon monoxide (CO), hydrocarbons (HC), particulate matter (PM), and nitrous gases such as nitrogen monoxide (NO) and nitrogen dioxide (NO2) (together abbreviated NOx). Soot is a mass of impure carbon particles resulting from the incomplete combustion of hydrocarbons. Soot is considered a hazardous substance with carcinogenic properties. Among these diesel emission components, particulate matter has been a serious concern for human health due to its direct and broad impact on the respiratory organs. In earlier times, health professionals associated PM10 (diameter < 10 μm) with chronic lung disease, lung cancer, influenza, asthma, and increased mortality rate. However, recent scientific studies suggest that these correlations be more closely linked with fine particles (PM2.5) and ultra-fine particles (PM0.1). Plant leaf structure Upper epidermis This is a single layer of cells containing few or no chloroplasts. The cells are quite transparent and permit most of the light that strikes them to pass through to the underlying cells. The upper surface is covered with a waxy, waterproof cuticle, which serves to reduce water loss from the leaf. Palisade layer - mesophyll This consists of one or more layers of cylindrical cells oriented with their long axis perpendicular to the plane of the leaf. The cells are filled with chloroplasts (usually several dozen of them) and carry on most of the photosynthesis in the leaf. Plant leaf structure Spongy layer - mesophyll its cells are irregular in shape and loosely packed. Although they contain a few chloroplasts, their main function seems to be the temporary storage of sugars and amino acids synthesized in the palisade layer. They also aid in the exchange of gases between the leaf and the environment. During the day, these cells give off oxygen and water vapor to the air spaces that surround them. They also pick up carbon dioxide from the air spaces. The air spaces are interconnected and eventually open to the outside through pores called stomata (sing., stoma). Plant leaf structure Lower epidermis Typically, most of the stomata (thousands per square centimeter) are located in the lower epidermis. Although most of the cells of the lower epidermis similar to those of the upper epidermis, each stoma is surrounded by two sausage-shaped cells called guard cells having chloroplasts. The guard cells regulate the opening and closing of the stomata. Thus they control the exchange of gases between the leaf and the surrounding atmosphere. Xerophytes Xerophyte, any plant adapted to life in a dry or physiologically dry habitat (salt marsh, saline soil, or acid bog) by means of mechanisms to prevent water loss or to store available water. Succulents (plants that store water) such as cacti and agaves have thick, fleshy stems or leaves. Adaptations Very few stomata Sunken stomata Hairs surrounding stomata Needle-shaped or small leaves Thickened waxy cuticle Halophytes Hydrophytes Plants adapted to living in water are called hydrophytes. Roots of hydrophytes are poorly developed and have air spaces to get oxygen from mud Stems lack much support as water provides buoyancy for the plant PROTEINS AND ENZYMES NEIS-PORT SAID The Fundamental Structure of Proteins Proteins are the polymers of α (20 type) amino acids and they are connected to each other by a peptide bond or peptide linkage. The elements that make up proteins are carbon , hydrogen , oxygen as well as nitrogen. Types of R-groups Positively charged side chain. Negatively charged side chain. Polar, uncharged side chain. Hydrophobic side chain. Special cases like SH group Peptide bonds are covalent bonds and so involve the sharing of electrons In order to form a peptide bond : 1. A hydroxyl (-OH) is lost from the carboxylic group of one amino acid 2. A hydrogen atom is lost from the amine group of another amino acid 3. The remaining carbon atom (with the double-bonded oxygen) from the first amino acid bonds to the nitrogen atom of the second amino acid This is a condensation reaction so water is released During hydrolysis reactions, the addition of water breaks the peptide bonds resulting in polypeptides being broken down to amino acids Primary Structure of Protein The sequence of amino acids bonded by covalent peptide bonds is the primary structure of a protein The DNA of a cell determines the primary structure of a protein by instructing the cell to add certain amino acids in specific quantities in a certain sequence. This affects the shape and therefore the function of the protein. The primary structure is specific for each protein (one alteration in the sequence off amino acids can affect the function of the protein). Secondary Structure of Protein Secondary structure: it is linking of the amino acids by hydrogen bonds between the oxygen of the –CO– group of one amino acid and the hydrogen of the –NH– group of the amino acid four places ahead of it. The hydrogen bonded to the Nitrogen atom and the Carbonyl oxygen There are two shapes that can form within proteins due to the hydrogen bonds: o α-helix o β-pleated sheet The α-helix shape occurs when the hydrogen bonds form between every fourth peptide bond (between the oxygen of the carboxyl group and the hydrogen of the amine group). The β-pleated sheet shape forms when the protein folds so that two parts of the polypeptide chain are parallel to each other enabling hydrogen bonds to form between parallel peptide bonds. The hydrogen bonds can be broken by high temperatures and pH changes. Tertiary Structure of Protein Additional bonds forming between the R groups (side chains) The tertiary structure of a protein consists of the way a polypeptide is formed of a complex molecular shape. This is caused by R-group interactions such as disulfide (covalent) bonds, hydrogen bonds (broken by high Temp. or adding alcohol, …..), ionic bonds (adding acid or alkali) , and hydrophobic interactions. The additional bonds are: o Hydrogen (these are between R groups) weak bond o Disulphide (only occurs between cysteine amino acids) Strong covalent o Ionic (occurs between charged R groups) broken by change in pH o Weak hydrophobic interactions (between non-polar weak bond A protein becomes denatured when its normal shape gets deformed because some of these bonds are broken. Protein quaternary structure Protein quaternary structure is the fourth classification level of protein structure. Protein quaternary structure refers to the structure of proteins which are themselves composed of two or more smaller protein chains. Occurs in proteins that have more than one polypeptide chain working together as a functional macromolecule, for example, haemoglobin The same bonds responsible for maintaining the tertiary structure of a protein will also be involved in forming the quaternary structure. Each polypeptide chain in the quaternary structure is referred to as a subunit of the protein. Collagen Collagen is fibrous protein composed of 3 chains bonded by hydrogen bonds. The chains are wound together to form a triple helix. Since glycine is the smallest of all the amino acids, it allows the chain to form a tight configuration, and it can withstand stress. Every third amino acid is glycine. Collagen is the primary building block of your body's skin, muscles, bones, tendons and ligaments, and other connective tissues. It's also found in your organs, blood vessels and intestinal lining. The hemoglobin is a globular protein made up of four polypeptide chains: two alpha chains of 141 amino acid residues each and two beta chains of 146 amino acid residues each. each of which has a single covalently bound heme group. Each of the four heme groups is made up of an iron Fe2+. Oxygen binds to ferrous iron in heme. Biuret protein assay is based on the principle that copper ions (Cu2+) react with peptide bonds in proteins, forming a complex. It is a chemical test used for detecting the presence of at least two peptide bonds in a molecule. In the presence of peptides, a copper(II) ion forms mauve-colored coordination complexes in an alkaline solution. The reaction in the biuret test is a colorimetric reaction where the result is indicated by a color change from blue to purple or violet. In an alkaline environment, the cupric (Cu+2) ions in the biuret reagent bind to the nitrogen atoms in the peptide bonds of proteins forming a violet-colored copper coordination complex. The formation of purple color indicates the presence of peptide bonds in the sample. The intensity of the developed purple color is directly proportional to the concentration of peptide bonds present in the solution. Enzymes All enzymes are proteins but all proteins are not enzymes. Without enzymes, most metabolic reactions would take much longer and would not be fast enough to sustain life. Enzymes are very efficient catalysts for biochemical reactions. Enzymes are mainly globular proteins. Enzymes bind with chemical reactants called substrates. The preference of an enzyme for one specific substrate is defined as the specificity The substrate binds to the active site of the enzymes leading to an enzyme–substrate complex. After releasing the product, the enzyme is again ready to bind the next substrate molecule. The active site consists of amino acid residues (side chains or R groups) that form temporary bonds with the substrate, the binding site, and residues that catalyze a reaction of that substrate. Enzymes and activation energy Enzymes work by lowering the activation energy of chemical reactions. Activation energy is the energy needed to start a chemical reaction. Enzymes work by binding to reactant molecules and holding them in such a way that the chemical bond-breaking and bond- forming processes take place more readily. Mode of action of enzymes Lock and Key Model Induced Fit Model Vs. Lock and Key A German scientist, Emil Fischer postulated the lock and key model in 1894 to explain the enzyme’s mode of action. This model assumes that the active site of the enzyme and the substrate fit perfectly into one another such that each possesses specific predetermined complementary geometric shapes and sizes. In this model, enzymes are depicted as highly specific. They must bind to specific substrates before they catalyze chemical reactions. Mode of action of enzymes Induced Fit Model Vs. Lock and Key Induced Fit Model This model proposes that the initial interaction between enzyme and substrate is relatively weak, but that these weak interactions rapidly induce conformational changes in the enzyme that strengthen the binding which maximizes the catalyzing action. Measuring Enzyme Activity The progress of enzyme-catalysed reactions can be investigated by: Measuring the rate of formation of a product Measuring the rate of disappearance of a substrate Factors affecting enzyme action 1-Temperature Enzymes have a specific optimum temperature – the temperature at which they catalyse a reaction at the maximum rate Lower temperatures either prevent reactions from proceeding or slow them down: Molecules move relatively slow Less frequent enzyme-substrate complex formation Substrate and enzyme collide with less energy, making it less likely for bonds to be formed or broken (stopping the reaction from occurring) Higher temperatures speed up reactions: Molecules move more quickly More frequent enzyme-substrate complex formation Substrate and enzyme collide with more energy, making it more likely for bonds to be formed or broken (allowing the reaction to occur) Factors affecting enzyme action 1-Temperature However, as temperatures continue to increase, the rate at which an enzyme catalyses a reaction drops sharply, as the enzyme begins to denature: High temperatures causes the hydrogen bonds between amino acids to break, changing the conformation of the enzyme Bonds (eg. hydrogen bonds) holding the enzyme molecule in its precise shape start to break This causes the tertiary structure of the protein (ie. the enzyme) to change This permanently damages the active site, preventing the substrate from binding Denaturation (irreversible) has occurred if the substrate can no longer bind Very few human enzymes can function at temperatures above 50°C This is because humans maintain a body temperature of about 37°C, therefore even temperatures exceeding 40°C will cause the denaturation of enzymes. However in some bacteria, the enzyme optimum temperature may reach 90oc (structure of the enzyme may be stabilized with strong covalent bonds). On the other hand, some plant enzymes have lower optimum temperature, depending on their habitat. Factors affecting enzyme action 2- pH Factors affecting enzyme action 3- Enzyme Concentration Factors affecting enzyme action 4- Substrate Concentration Factors affecting enzyme action 5- Enzyme Inhibitors (reversible) (irreversible) Enzymes which use the induced fit mechanism can be less affected by competitive inhibitors. Enzymes which use the induced fit mechanism can be less affected by competitive inhibitors. This is because the inhibitor does not induce the same change in the active site as the substrate does. End-product inhibition (-ve feedback) is when the final product inhibits an enzyme involved in the initial reactions. At the end of the metabolic pathway, the final product may be able to inhibit the enzyme responsible for catalysing the initial reaction. This causes the whole metabolic pathway to stop The rate of Enzyme activity Maximum rate (velocity) Vmax Vmax & the Michaelis-Menten Constant The Michaelis-Menten model describes the kinetics of such enzyme catalysed reactions In this model, two values are used to describe an enzyme catalysed reaction, the maximal rate or maximal velocity (Vmax) and the Michaelis-Menten constant (Km) These values are derived from the reaction rate at different substrate concentrations. The two important values deduced are the Vmax (maximum rate of reaction at saturating substrate concentrations) and the Km, which is the substrate concentration at ½Vmax (also known as the Michaelis-Menten constant). The Michaelis-Menten constant is the substrate concentration at which the enzyme works at half its maximum rate At this point, half of the active sites of the enzyme are occupied by substrate molecules The higher the affinity of the enzyme for the substrate, the lower the substrate concentration needed for this to occur This is why the Michaelis-Menten constant is a measure of the affinity of an enzyme for its substrate. Vmax gives information about the maximum rate of reaction that is possible, while Km measures the affinity. Km is an inverse measure of the substrate's affinity for the enzyme ; an enzyme with a high Km has a low affinity for its substrate and an enzyme with a low Km has a high affinity for its substrate The presence of competitive inhibitor the Km will increase but the value of Vmax does not change The presence of noncompetitive inhibitor on the other hand will reduce the Vmax but Km will remain the same since the substrate binding site is not occupied by the inhibitor Immobilising enzymes Immobilised enzymes are enzymes that have been bound to an inert, stationary and insoluble material such as alginate The substrate is then passed over the immobilised enzyme and the product is collected This allows enzymes to be held in place throughout the reaction, following which they are easily separated from the products and may be used again. Milk is a valuable source of nutrients containing protein, fat and the carbohydrate Lactose Some people are lactose intolerant Lactose is a disaccharide that is broken down into glucose and galactose Enzymes in industry and medicine Pectinase enzyme removes the soluble pectin from the juice and amylases are used to remove the starch from the juice that causes the unwanted haze in the juice and also is responsible for the gel formation in the juice during storage. Pectinase also increases press capacity which increases juice production yield. amylase and cellulase in cotton fabrics -The textile industry amylases are used to remove starch-based size for improved and uniform wet processing. Amylase is a hydrolytic enzyme which catalyzes the breakdown of dietary starch to short chain sugars, dextrin and maltose. - Cellulases are widely used in the textile industry for the manufacture and finishing of cellulose-containing materials.... In the detergent industry cellulases are used to provide cleaning and fabric-care benefits such as the brightening of colour in faded garments by removing fuzz. lactase and managing lactose intolerance Adenosine deaminase and ADA deficiency Adenosine deaminase (ADA) deficiency is a disorder that affects the immune system. Specifically, ADA deficiency impairs the development and function of immune cells called lymphocytes. CLASSIFICATION & BIODIVERSITY NEIS-PORT SAID 24/25 CLASSIFICATION Scientific taxonomy used to group and categorize organisms into groups such as genus or species. These groups are known as taxa (singular: taxon). It is based on structural or functional similarities or evolutionary history. CLASSIFICATION Organisms are put into three-domain system. The three domains of life are conceptualized as Archaea(extremophiles),the typical Bacteria, and Eukaryote (comprising the nuclei-bearing eukaryotes). Archaea are a group of micro-organisms that are similar to, but evolutionarily distinct from bacteria. Many archaea have been found living in extreme environments, for example at Some Archaea produce methane and cannot survive in high pressures, salt concentrations or the presence of oxygen and have many unusual enzymes. temperatures. Many people think that eukaryotes are all multicellular, but this is not the case. While prokaryotes are always unicellular organisms, eukaryotes can be either unicellular or multicellular. For example, most protists are single-celled eukaryotes! Each human cell has about 1.8 meters of DNA if completely stretched out; however, when wound about histones, this length is reduced to about 90 micrometers (0.09 mm) of 30 nm diameter chromatin fibers. PHYLOGENETIC TREE A phylogenetic tree is made up of several branches—it’s called a tree for a reason! Each branch represents a lineage, and the tips of the branches represent the taxa being examined. These two trees deliver the same information in different ways. Take a closer look at the branch points and you will see that the two trees show the same relationships between taxa. VIRUSES Viruses are microorganisms (ranging in size from 20-300 nm) visible only with the electron microscope. Viruses are not included in the three domain classification system since they are acellular i.e. they do not have cellular structures as they are not surrounded by a partially permeable membrane containing cytoplasm and ribosomes. All viruses are parasitic because they can only reproduce by infecting and taking over living cells. The virus DNA or RNA takes over the protein synthesizing machinery of the host cell with the help of energy released from the respiration in the host cells, which then helps to make new virus particles (the host cells make copies of virus nucleic acids and make their protein). THE BIOLOGICAL SPECIES CONCEPT The biological species concept defines a species taxon as a group of organisms that can successfully interbreed and produce fertile offspring. not according to similarity of appearance. Although appearance is helpful in identifying species, it does not define species. Western meadowlarks (Sturnella neglecta) and Eastern meadowlarks (Sturnella magna) look almost identical to one another, yet do not interbreed with each other — thus, they are separate species according to this definition. Organisms may look different and yet be the same species. For example, look at these ants. You might think that they are distantly related species. In fact, they are sisters—two ants of the species Pheidole barbata, fulfilling different roles in the same colony. SPECIES VS SUBSPECIES In biological classification, subspecies (pl.: subspecies) is a rank below species, used for populations that live in different areas and vary in size, shape, or other physical characteristics (morphology), but that can successfully interbreed. Compare genes (nucleotides) or proteins (amino acids) sequences across species In a protein / gene sequence analysis, for example, the more amino acids/nucleotides that match up, the more closely related the two species will be. Molecular homology is the similarity amongst organisms at the molecular level due to shared ancestry. How closely two organisms are related can be determined by the degree of similarity between the base sequence in their nucleic acids. Additionally, similarity of amino acid sequences in their proteins also help to determine the degree of relatedness. The sequence of amino acids in the haemoglobin molecule is very similar in chimpanzees and humans. This indicates that humans and chimpanzees are more closely related than humans and cats. Just for example !!!! Comparing amino acids sequences ENVIRONMENT Environment is sum total of all the living and non-living elements and their effects on living organism life. BIODIVERSITY Biodiversity — short for biological diversity — is the variety of all living things and their interactions. Why Is Biodiversity Important? Many basic needs humans obtain from biodiversity such as food, fuel, shelter, and medicine. Ecosystems provide crucial services such as pollination, seed dispersal, climate regulation, water purification, nutrient cycling, and control of agricultural pests. Other potential benefits not yet recognized. BIODIVERSITY LEVELS LEVELS OF ORGANIZATION Fish Population or community ? BASIC DEFINITIONS An individual is one organism and is also one type of organism (e.g., human, cat, moose, palm tree, gray whale, tapeworm, or cow in our example). A species is a class of living organism whose members have the same main characteristics and are able to breed with each other. Endemic species are those that are restricted to a geographical area and do not occur naturally in any other part of the world. A population is defined as a group of individuals of the same species living and interbreeding within a given area. Community is a group or association of populations of two or more different species occupying the same geographical area at the same time. entire set of interacting species populations An ecosystem consists of a community of organisms together with their physical environment. Biome: a grouping of ecosystems on a given continent that is similar in vegetation structure, physiognomy, features of the environment and characteristics of their animal communities. Difference Community Ecosystem Several communities, with all their BASIC DEFINITIONS A group of living organisms living living and nonliving components Definition and interacting only with each and their interactions, form an other forms a community. ecosystem. A community is a part of an The ecosystem comprises several Level ecosystem; therefore, it’s on a communities; therefore, the smaller level. ecosystem occupies a larger level. An ecosystem includes both living A community is only composed of Components (biotic) and non-living (abiotic) living or biotic components. components. In an ecosystem, the interactions In a community, interactions happen both between living Interactions happen only between living components and living and organisms or biotic components. nonliving components. All birds, animals, plants, micro- A pond, a forest, a meadow, etc., Example organisms, etc., living in a forest are examples of an ecosystem. form a community. Species richness measures the number of different species while species evenness tells whether the ecosystem has a Species richness is a dominant species or has similar measure of the number abundances of all species. of different types of species in an ecosystem. Species evenness is a measure of the relative abundance of each species. BIODIVERSITY COMPONENTS Three major components of biodiversity are species diversity, ecological diversity, genetic diversity, and functional diversity. Species Diversity? Species diversity is the number of species and abundance of each species that live in a specific location. Why? GENETIC DIVERSITY Genetic diversity is the variation of genes that exist within a species population. Genes are what make up our unique DNA code, carrying information that determines certain features or characteristics about you. These are called traits. Every individual in a species is unique because of these differences in traits. Genetic diversity is the biological variation that occurs within species. It makes it possible for species to adapt when the environment changes. ASSESSING SPECIES DIVERSITY (COLLECTING AND MAKING SPECIES LIST & SAMPLING) Estimating the Size of a Population Measuring all the different levels of biodiversity within an ecosystem could be very time consuming Finding out which species live in an ecosystem and the size of the populations requires the identification and cataloguing of all organisms present to build a species list This is possible for areas that are very small or where the species are very large like trees However, for larger and more complex ecosystems like rainforests, it is simply impossible to find, identify and count every organism that exists there When this is the case different samples of the area can be taken and used to make an estimate for the total species numbers in the area ASSESSING SPECIES DIVERSITY (COLLECTING AND MAKING SPECIES LIST & SAMPLING) Sampling Sampling is a method of investigating the abundance and distribution of species and populations There are two different types of sampling: Random Systematic In random sampling the positions of the sampling points are completely random or due to chance This method is beneficial because it means there will be no bias by the person that is carrying out the sampling that may affect the results. In systematic sampling the positions of the sampling points are chosen by the person carrying out the sampling There is a possibility that the person choosing could show bias towards or against certain areas Individuals may deliberately place the quadrats in areas with the least species as these will be easier and quicker to count This is unrepresentative of the whole area When a sampling area is reasonably uniform or has no clear pattern to the way the species are distributed then random sampling is the best choice PRACTICAL QUANTITATIVE ECOLOGY Random sampling -frame quadrat Systematic sampling -belt transect. -line transect. Mark-release-recapture (for animals and insects) The distribution of a species describes how it is spread throughout the ecosystem The abundance of a species is the number of individuals of that species The distribution and abundance of non-motile or slow-moving species in an area can be assessed using two different practical methods: Frame Quadrats Belt Transects PRACTICAL QUANTITATIVE ECOLOGY a. Random sampling. simple random sampling, all samples within the region have an equal chance of being selected.. Advantages Simplicity Requires little prior knowledge of the population Disadvantages Lower accuracy Higher cost Lower efficiency Samples may be clustered spatially Samples may not be representative of the feature attribute(s) PRACTICAL QUANTITATIVE ECOLOGY a. Random sampling. When to use random sampling. Random sampling is usually carried out when the area under study is fairly uniform, very large, and or there is limited time available. The frame is placed on the ground (or on whatever is being investigated) and the animals, and/ or plants inside it counted, measured, or collected, depending on what the survey is for. Steps of random sampling Step 1: Define the population. Start by deciding on the population that you want to study... Step 2: Decide on the sample size. Next, you need to decide how large your sample size will be.... Step 3: Randomly select your sample.... Step 4: Collect data from your sample. RANDOM SAMPLING --- PITFALL TRAP PRACTICAL QUANTITATIVE ECOLOGY b. Systematic sampling.. c. Mark-release andPRACTICAL QUANTITATIVE ECOLOGY recapture Lincoln Index.. PRACTICAL QUANTITATIVE ECOLOGY SPECIES ABUNDANCE INTERACTIONS We need to analyze the relationships between the abundance of species with abiotic or biotic factors !! Spearman's rank correlation Spearman's rank correlation measures the strength and direction of association between two ranked variables (Species abundance and Environmental factors). The calculated value range between -1 to 1. +ve -ve No Step 3 calculate d squared SPEARMAN'S RANK CORRELATION COEFFICIENT An ecologist collected Prawn and Mullet from 6 different Location along Mitterrandian sea representing different degrees of temperature. Location No. Prawn No. Mullet Temp. ºC Egypt 15 10 20 Libya 38 15 12 Tunisia 6 50 11 Morocoo 7 4 14 Spain 8 7 14 France 30 60 14 The standard deviation reflects variability within a sample, while the standard error estimates the variability across samples of a population. What is biodiversity and why is it different in different Places? Biodiversity refers to the number of biological species that exist in a given region. High biodiversity means that a region supports a wide variety of species, while low biodiversity implies that an area supports only a few. The reasons for variances in biodiversity are complex, but they include both natural and man-made causes. Natural or human-induced factors that directly or indirectly cause a change in biodiversity are referred to as drivers. Direct drivers that explicitly influence ecosystem processes. include land use change, climate change, invasive species, overexploitation, and pollution. There are many factors that can affect biodiversity, including: habitat destruction and fragmentation, over-exploitation of resources, introduction of invasive species, climate change, pollution, and disease. Coral reefs are believed by many to have the highest biodiversity of any ecosystem on the planet—even more than a tropical rainforest. Occupying less than one percent of the ocean floor, coral reefs are home to more than 25% of all marine life. Why is that important? A highly biodiverse ecosystem, one with many different species, is often more resilient to changing conditions and can better withstand significant disturbances. Each species plays its own function in a coral reef ecosystem. Some are herbivores and specialize in eating different kinds of algae, keeping corals from being smothered by their potentially deadly competitors. Others, like sharks, groupers, and other predatory fish, keep populations of smaller fish and other organisms in balance. https://coral.org/en/coral-reefs-101/why-care-about- reefs/biodiversity/#:~:text=Coral%20reefs%20are%20believed%20by,25%25%20of%20all%20marine%20life. On the other hand environments in which abiotic factors make it difficult for organisms to survive tend to have lower biodiversity The biodiversity, or variety of life in the desert, is based upon organisms that are specially adapted to survive without a ready supply of water. Whether hot or cold, the biodiversity of the desert is very low. Plants and animals living in the desert need special adaptations to survive in the harsh environment. Plants tend to be tough and wiry with small or no leaves, water-resistant cuticles, and often spines to deter herbivory. Some annual plants germinate, bloom and die in the course of a few weeks after rainfall, while other long-lived plants survive for years and have deep root systems able to tap underground moisture. Animals need to keep cool and find enough food and water to survive. Many are nocturnal, and stay in the shade or underground during the heat of the day. They tend to be efficient at conserving water, extracting most of their needs from their food and concentrating their urine. Some animals remain in a state of dormancy for long periods, ready to become active again during the rare rainfall. They then reproduce rapidly while conditions are favorable before returning to dormancy. A narrow niche is specific and limited and a broad niche is less specific and less limited. A species with a broad niche, also called a generalist.A generalist species is able to thrive in a wide variety of environmental conditions and can make use of a variety of different resources (for example, a heterotroph with a varied diet). A specialist species can thrive only in a narrow range of environmental conditions or has a limited diet. A species with a narrow niche, also called a specialist. Specialist Generalist POLLUTION NEIS – PORT SAID 2024/2025 POLLUTION Pollution: the addition of any substance (solid, liquid, or gas) or any form of energy (such as heat, sound, or radioactivity) to the environment at a rate faster than it can be dispersed, diluted, decomposed, recycled, or stored in some harmless form. 1- Water Pollution 1- Water Pollution A fertilizer is a plant nutrient that is added to the soil to promote growth. A pesticide is a substance that kills or controls pests, usually insects. Bacterial Bloom Eutrophication is the process in which a water body becomes overly enriched with nutrients, leading to the plentiful growth of simple plant life. The excessive growth (or bloom) of algae and plankton in a water body are indicators of this process. How do Water Bodies Become Overly Enriched? The availability of nutrients such as nitrogen and phosphorus limits the growth of plant life in an ecosystem. When water bodies are overly enriched with these nutrients, the growth of algae, plankton, and other simple plant life is favoured over the growth of more complex plant life. Phosphates tend to stick to the soil and are transported along with it. Therefore, soil erosion is a major contributor to the phosphorus enrichment of water bodies. Some other phosphorus-rich sources that enrich water bodies with the nutrient include: Fertilizers Untreated sewage Detergents containing phosphorus Industrial discharge of waste. Water Remediation Water remediation refers to the process of treating and purifying water contaminated by pollutants from various sources such as industrial effluents and anthropogenic activities to restore its quality for sustainable use. Sewage treatment (or domestic wastewater treatment, municipal wastewater treatment) is a type of wastewater treatment which aims to remove contaminants from sewage to produce an effluent that is suitable to discharge to the surrounding environment or an intended reuse application, thereby preventing water pollution. Untreated sewage may contain water; nutrients (nitrogen and phosphorus); solids (including organic matter); pathogens (including bacteria, viruses and protozoa); helminthes (intestinal worms and worm-like parasites) ; oils and greases; runoff from streets, parking lots and roofs; heavy metals (including mercury). Step 1: Screening and Pumping Step 5: Secondary Settling The incoming wastewater passes through sc