Final Physics Exam Revision Grade 11 PDF
Document Details
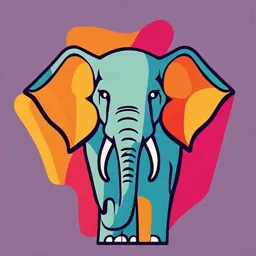
Uploaded by StatelyProtagonist7823
Tags
Related
- Chapter 9 Light - Reflection and Refraction PDF
- Class 10 Science: Light Reflection and Refraction Revision Notes PDF
- CBSE Class 10 Science Light Reflection & Refraction Notes PDF
- Physics Lecture: Light and Optics in Arabic
- Physics Class 10th PDF: Reflection, Refraction, Electricity, & Magnetism
- Light Physics Cheat Sheet PDF
Summary
This document is a physics study guide for grade 11 students, covering topics such as reflection and refraction of light, mirrors, and lenses. It includes explanations of key concepts, diagrams, and practice questions to help students prepare for their final exams. The guide aims to help revise the topics covered in the exam.
Full Transcript
Final Physics Exam Revision - Grade 11 Prepared by Physics and chemistry department The Exam content: Topic 22: Reflection and Refraction of Light. 22.1&2 The Nature of light, Reflection Pp. 723-725 22.2 Refraction of Light Pp. 726-727 22.3 The law of Refraction Pp. 728-732 22.4 Dispersion and Pri...
Final Physics Exam Revision - Grade 11 Prepared by Physics and chemistry department The Exam content: Topic 22: Reflection and Refraction of Light. 22.1&2 The Nature of light, Reflection Pp. 723-725 22.2 Refraction of Light Pp. 726-727 22.3 The law of Refraction Pp. 728-732 22.4 Dispersion and Prisms Pp. 733-734 22.5 The Rainbow Pg 736 22.6 Huygens' Principle 22.7 Total Internal Reflection Pp. 736-7340 Topic 23: Mirrors and Lenses. 23.1 Flat Mirrors and Introducing 23.2 Images Formed by Spherical Mirrors Pp. 750 - 755 23.2 Images Formed by Spherical Mirrors 23.3 Images Formed by Refraction Pp. 755 - 762 23.5 Thin Lenses Pp. 764 - 771 Exam Study Resources: 1. Final Physical Science Exam Revision Presentation. 2. The Booklet on Classera (contains practice questions) 3. Serway, Raymond A., and Vuille, Chris. College Physics, 11th Edition, Cengage Learning Topic 22: Reflection and Refraction of Light 22.1 The Nature of Light Particle Theory of Light (Newton): – Light modeled as a stream of particles emitted by a source that stimulated the sense of sight on entering the eye. – Provided simple explanations for reflection and refraction. Wave Theory of Light (Huygens): – During Newton’s lifetime, however, Wave Theory was proposed. – He showed that a wave theory of light could also explain the laws of reflection and refraction. Electromagnetic Wave Theory (Maxwell) Light identified as high-frequency electromagnetic waves. Predicted the speed of light to be consistent with experimental measurements. c= 3 x 108 m/s Photoelectric Effect (Hertz): Clean metal surfaces emit charges when exposed to ultraviolet light. This phenomenon could not be explained by classical wave theory. Explained the photoelectric effect: energy from a single photon is transferred to an electron, behaving like a particle. Einstein’s Photon Theory: Light consists of particles called photons. The energy of a photon is proportional to its frequency: E = h · f where H : Planck’s constant, F : is the frequency of the light. Photon: A quantum of light with particle-like properties. The Dual Nature of Light Light exhibits wave-like behavior in: – Propagation, reflection, refraction, and interference. Light exhibits particle-like behavior in: – The photoelectric effect and energy transfer. Reflection Reflection happens when a wave bounces off a surface. You see your reflection in a mirror because light waves bounce off the smooth surface and back to your eyes. Sound can also reflect; that’s how echoes are created. Refraction When a wave enters a new medium (like light going from air to water), it bends.This bending is called refraction. You’ve seen this happen when you put a straw in a glass of water and the straw looks bent or broken at the water's surface. That’s because light travels slower in water than in air, causing the wave to change direction. Diffraction Diffraction is when waves bend around obstacles or spread out after passing through a small opening. If you’ve ever heard someone talking from around the corner, that’s because sound waves are diffracting around the wall. Interference When two waves meet, they interact with each other. This is called interference, and it can happen in two ways: Constructive interference: When waves add together, their energies combine, and the wave gets bigger. This is like when two people in a stadium cheer at the same time, making the sound louder. Destructive interference: When waves cancel each other out, their energies reduce, and the wave gets smaller or disappears. It’s like when noise-canceling headphones stop outside sounds by producing sound waves that cancel them out! 22.2 Reflection and Refraction Light travels in straight lines in a uniform medium until it encounters a boundary. Ray Approximation: Light is represented as a straight line, called a ray, along its direction of travel. Reflection of Light: Types of Reflection Specular Reflection: – Occurs on smooth, mirror-like surfaces. – Reflected rays are parallel, preserving the beam’s structure. – Example: Reflection from calm water or a polished metal surface. Diffuse Reflection: – Occurs on rough surfaces. – Rays scatter in multiple directions. – Example: Light reflecting off a dry road. Real-World Example: Wet vs. Dry Roads Dry road: Diffuse reflection scatters light, making the road visible. Wet road: Specular reflection directs light away from the observer, making the road harder to see. Law of Reflection The angle of incidence, θi , equals the angle of reflection, θr θi = θ r Both angles are measured relative to the normal, a line perpendicular to the surface at the point of incidence. 22.3 The Law of Refraction Refraction occurs when light passes from one medium to another, causing the light to bend due to a change in its speed. Properties: – The incident ray, reflected ray, refracted ray, and the normal at the point of incidence all lie in the same plane. – The bending of the light ray occurs due to a change in its speed as it moves between media with different optical densities. Refraction Scenarios Light Speed Changes: From fast to slow medium (e.g., air to glass): – θ2 < θ1: The refracted ray bends toward the normal. From slow to fast medium (e.g., glass to air): – θ2 > θ1: The refracted ray bends away from the normal. Effects of Speed Changes (a) Light bending toward the normal (high to low speed medium). (b) Light bending away from the normal (low to high speed medium). Index of Refraction (n) The index of refraction is the ratio of the speed of light in a vacuum (c) to the speed of light in the medium (v) The index of refraction is a measure of how much a medium slows down light compared to a vacuum. Defined as: where: – c = speed of light in vacuum, – v = speed of light in the medium. It is a dimensionless number. Laws of Refraction (Snell’s Law): The angle of refraction depends on the properties of the two media and on the angle of incidence, through the relationship θ1: Angle of incidence (measured from the normal). θ2: Angle of refraction (measured from the normal). n1: Index of refraction for the first medium. n2: Index of refraction for the second medium. If a ray travels from point A to B through a refracting surface, the same path will be followed in reverse if the light originates from B. Frequency (f): Frequency remains constant when light passes from one medium to another. Ensures continuous wave motion across the boundary. Wavelength (λ): Wavelength changes depending on the medium. It is related to the speed of light in the medium. 22.4 Total Internal Reflection: Occurs when light travels from a medium with a higher index of refraction (n1) to a medium with a lower index of refraction (n2). If the angle of incidence exceeds a certain limit, called the critical angle (θc), all light reflects back into the first medium. Beyond the critical angle, no refraction occurs, and the reflection behaves as though from a perfect Mirror. Critical Angle (θc) The angle at which the refracted ray travels parallel to the boundary between the two media. Determined by Snell’s Law: Fiber Optics Thin strands of glass or plastic guide light through successive internal reflections. Light remains confined within the fibers, even around curves, enabling efficient transmission over long distances. Fiber Optics in Communications: Telecommunications: Fiber optics transmit high-speed data for internet, television, and telephone services. Advantages: – Immune to electromagnetic interference. – Minimal signal loss over long distances. 22.5 Huygens’ Principle: - Huygens’ Principle states that every point on a wave front can be considered a point source for spherical secondary waves, called wavelets. - After a short time interval, the new wave front is the surface tangent to these wavelets. This principle can be used to predict the future position of the wave front. It explains the propagation of light as a series of spherical wavelets originating from each point on the wave front. Each point on the wave front emits spherical secondary waves called wavelets. These wavelets travel at the same speed as the primary wave. The new wave front at a later time is tangent to all of these wavelets. Wave Front: A wave front is a surface connecting all points of a wave that have the same phase and amplitude. For example, a wave front could be a surface that passes through the crests of all the light waves at a given moment. There are two main types of wave fronts: Plane wave fronts: Straight lines where the waves travel in parallel directions. Spherical wave fronts: Curved surfaces where the waves radiate from a point source. Wave Front: The surface through which all points of the wave are in phase. Wavelets: Spherical secondary waves emanating from each point on a wave front, used to construct the future wave front. 22.6 Dispersion and Prisms Dispersion is the phenomenon where the index of refraction (n) of a material changes with the wavelength of light. Cause: Shorter wavelengths (e.g., violet light) interact more strongly with a material, bending more, while longer wavelengths (e.g., red light) bend less. Effect: Violet light refracts more than red light when transitioning between air and another medium, leading to the separation of colors. Wavelength Impact: The index of refraction (n) depends on wavelength, so the refraction angle θ2 varies for different colors of light. Light Interaction with a Prism Single Wavelength: When a single wavelength of light enters a prism, it bends at the entry and exit faces. The light emerges at an angle known as the angle of deviation (δ). White Light: When white light (a mixture of all visible wavelengths) enters a prism, it spreads into a visible spectrum due to dispersion. The colors are ordered by decreasing wavelength: 1. Red (longest wavelength, least deviation) 2. Orange 3. Yellow 4. Green 5. Blue 6. Violet (shortest wavelength, most deviation) 22.7 The Rainbow: Dispersion of Light Light splits into its constituent colors (spectrum) when it passes through a medium like water. This process is called dispersion. Violet light deviates the most; red light deviates the least. Formation of a Rainbow A rainbow forms when sunlight interacts with raindrops in the atmosphere. The observer must be positioned between the Sun (at their back) and the rain shower (in front). Path of Light Through a Raindrop 1. Step 1: Light enters a raindrop and is refracted (bent), starting the dispersion process. 2. Step 2: Inside the drop, light reflects off the back surface. 3. Step 3: Light exits the drop, refracted again as it moves from water to air. Topic 23: Mirrors and Lenses Topic 23: Mirrors and Lenses. 23.1 Flat Mirrors: A flat mirror reflects light, causing light rays from an object to diverge and appear to originate from behind the mirror. The object distance (do) is the distance between the object and the mirror. The image distance (di) is the distance between the image and the mirror. To locate an image, trace at least two rays from a point on the object: 1. A ray perpendicular to the mirror reflects directly back. 2. An oblique ray follows the law of reflection and appears to originate from the image point. Image Properties of Flat Mirrors 1. Images formed by flat mirrors are virtual (light does not pass through the image) and upright. 2. The image is located as far behind the mirror as the object is in front (do = di). 3. The height of the image (hi) equals the height of the object (ho). 4. The lateral magnification (M) is given by: Flat mirrors produce an apparent left-right reversal. Key Characteristics Virtual Images: The properties of the image A flat mirror always formed by a flat mirror are: produces a virtual image. The image is virtual. The image appears to be The image is upright. located behind the mirror, but the light rays The image is the same size as do not actually pass the object. through the image point. 23.2 Images Formed by Spherical Mirrors A spherical mirror, as its name implies, has the shape of a segment of a sphere. 23.2.1 Concave Mirrors and the Mirror Equation Concave Mirror: A concave mirror has a curved, inward surface, which reflects light inward to a point. The principal axis is the line from the center of curvature C through the center of the mirror. The center of curvature C is the point at the center of the sphere from which the mirror is a segment. The focal point F is located halfway between the mirror’s surface and the center of curvature. The focal length f is half the radius of curvature R, so the relationship is: Ray Diagrams for Concave Mirrors: 1. Ray parallel to the principal axis reflects through the focal point. 2. Ray through the focal point reflects parallel to the axis. 3. Ray through the center of curvature reflects back on itself. Image Formation for a Concave Mirror: The nature, size, orientation, and position of the image formed by a concave mirror depend on The object’s position relative to the principal focus (F) and The center of curvature (C). Case 1: Object at Infinity Ray Diagram: Parallel rays incident on the mirror converge at the focal point. Image: – Position: At the focal point (F). – Nature: Real and inverted. – Size: Highly diminished (point sized). Case 2: Object at the Center of Curvature (C) Ray Diagram: Rays behave as above, but the intersection happens at the same distance from the mirror. Image: – Position: At C. – Nature: Real and inverted. – Size: Same size as the object. Case 3: Object at the Focal Point (F) Ray Diagram: Rays become parallel after reflection and do not meet. Image: – Position: At infinity. – Nature: No image is formed. – Size: Undefined. Case 4: Object Between F and the Pole (P) Ray Diagram: One ray parallel to the prin- cipal axis reflects through the focal point, and another aimed at the pole reflects symmetrically. Image: – Position: Behind the mirror. – Nature: Virtual and Upright. – Size: Magnified. Mirror Equation: The relationship between the object distance p, image distance q, and focal length f is given by the mirror equation: This equation allows calculation of the image distance q if the object distance p and focal length f are Known. Magnification The magnification m produced by the mirror is given by: where h is the image height, h is the object height, and the negative sign indicates an inverted image. 23.2.2 Convex Mirrors and Sign Conventions Convex Mirror is sometimes called a diverging mirror because the rays from any point on the object diverge after reflection, as though they were coming from some point behind the mirror. Image Formation for a Convex Mirror A convex mirror always forms virtual, upright, and diminished images, regardless of the object’s position. Case: Object at Any Position Ray Diagram: – One ray parallel to the principal axis reflects as if it is coming from the focal point (F). – Another ray directed toward the center of curvature (C) reflects back symmetrically. Image: – Position: Behind the mirror, between the pole (P) and the focal point (F). – Nature: Virtual. – Orientation: Upright. – Size: Diminished. Lens Types Convex lenses (thicker at the center): Focus light rays to a point. Concave lenses (thinner at the center): Spread light rays outward. A thin lens has two focal points, one on each side of the lens: For Convex lenses, parallel rays converge at the focal point. For Concave lenses, parallel rays diverge as if they originate from the focal point. Ray Diagrams for Thin Lenses Now consider a ray of light passing through the center of a lens. For a thin lens: A ray passing through the center of the lens is undeflected (Ray 1). A ray parallel to the principal axis passes through (or appears to originate from) the focal point after refraction (Ray 2). The intersection of these rays determines the position of the tip of the image. 23.3.1 Ray Diagrams for Thin Lenses: the following three rays are drawn from the top of the object: Ray 1: Drawn parallel to the principal axis. After being refracted by the lens, this ray passes through (or appears to come from) one of the focal points. Ray 2: Drawn through the center of the lens. This ray continues in a straight line. Ray 3: Drawn through the other focal point and emerges from the lens parallel to the principal axis. Image Formation by Thin Lenses For the Convex lens in Figure a, where the object is outside the front focal point, the ray diagram shows that the image is real and inverted. For the Convex lens, when the real object is inside the front focal point, as in Figure b, the image is virtual and upright. For the Concave lens in Figure c, the image is virtual and upright. 23.4 Lens and Mirror Aberrations Spherical Aberration Rays far from the principal axis focus at different points, causing a blurry image. Significant for spherical mirrors and lenses. Chromatic Aberration Caused by different wavelengths focusing at different points. Convex lenses refract violet light more than red light. Reduced using a combination of Convex and Concave lenses. Practice Question: Problem 1: An object is placed 30 cm in front of a convex mirror with a focal length of 15 cm. Find the image distance. Final Answer: The image distance is -10 cm, meaning the image is virtual and behind the mirror. Practice Question: Problem 2: A photon has a frequency of 6.2×1014 Hz. Using Planck’s constant (h= 6.63 x 10-34 J·s), calculate the energy of the photon. Final Answer: The energy of the photon is 4.11 × 10 − 19 J. Practice Question: Problem 3: A light ray passes from air (with a refractive index of 1.00) into a diamond. The angle of incidence in the air is 40°, and the angle of refraction in the diamond is 15°. Calculate the refractive index of the diamond. Final Answer: The refractive index of diamond is 2.48.