Renal Physiology PDF
Document Details
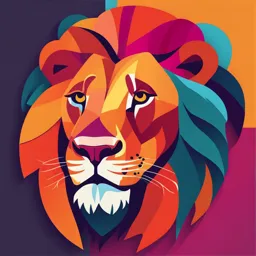
Uploaded by BrilliantMinneapolis
FH JOANNEUM
2015
Tags
Summary
These notes cover Renal Physiology, including the components of the urinary system, kidney functions, what urine is, urinalysis, etc. They contain images and diagrams.
Full Transcript
Renal Physiology Figure 26.1 An Introduction to the Urinary System Components of the Suprarenal Urinary System gland Kidney...
Renal Physiology Figure 26.1 An Introduction to the Urinary System Components of the Suprarenal Urinary System gland Kidney Renal artery Produces urine and vein Ureter Inferior Transports urine toward vena cava the urinary bladder Urinary Bladder Aorta Temporarily stores urine prior to elimination Urethra Conducts urine to exterior; in males, transports semen as well © 2015 Pearson Education, Inc. 2 Kidney Functions The Kidneys are the primary organs of the Urinary System At less than 1% of body weight, they receive 20- 25% of total cardiac output! Functions: Maintain blood volume Maintain fluid & electrolyte composition – Water – Ions Maintain acid/base balance Eliminate metabolic waste, toxic substances Vitamin D activation: hormone involved in Ca2+, PO43- absorption in small intestine Hormone production – EPO – red blood cell production – Renin – water/salt conservation What is Urine? Urine: protein free filtrate of the blood regulated by the kidneys Normally contains: excess water, ions, nitrogenous waste, small soluble compounds Composition changes as a function of blood/kidney regulation Should NOT contain: blood cells, large proteins (presence of these could indicate infection or damage to the kidneys) Urinalysis Urinalysis: analysis of urine sample using reagent strips (dipsticks) used to test properties and components – clear, color should be yellow-amber – odorless – pH=6-7 – specific gravity 1.001 1.030 – all reagents negative if NOT: leukocytes urinary tract infection blood infection, kidney stone glucose hyperglycemia, diabetes ketones starvation, ketoacidosis protein infection, heart failure, kidney disease, nephron dysfunction bilirubin hemolysis, liver disease urobilinogen liver disease Nephron tubules D i s t a l c o n v o l u t e d Proximal convoluted t u b tubule u l e 6 Loop of Henle a T C Renal corpuscleoh li n lc k e d c e ta is c n Nephron The Nephron is the BLOOD IN working unit of the kidney that produces urine NEPHRON Over 1 million Remove waste nephrons in each Regulate fluid kidney Regulate ions Blood into Nephron Regulate acid/base gets regulated, filtered, balanced balanced blood returned to circulation BLOOD OUT URINE OUT Nephron retains (regulated, filtered) (waste) excess, waste, toxins removed in urine BLOOD to BODY Nephron Nephron contains specialized blood vessels and tubules that regulate exchange of materials to/from the blood: Afferent Arteriole Renal Corpuscle: – Glomerulus – Bowman’s Capsule Efferent Arteriole Proximal Convoluted tubule (PCT) Peri-tubular capillaries & vasa recta Loop of Henle – Descending Limb (thin) – Ascending Limb (thick) Distal Convoluted tubule (DCT) Collecting Duct urine Cortical Radiate Veins blood to body Renal Blood Flow & Filtration Renal Perfusion: blood flow through the kidneys, including local regulation of the nephron. Efferent arteriole – receive 20-25% of Juxtaglomerular cardiac output Complex Macula densa – affected by systemic blood pressure Juxtaglomerular cells – regulated by local blood flow and resistance factors: autoregulation sympathetic nervous Afferent arteriole system hormones Renal Blood Flow & Filtration Renal Blood Flow: – receive 20-25% of cardiac output – 1000 – 1200mL per minute Renal Perfusion = blood flow to the kidneys, Efferent arteriole determined by mean arterial Juxtaglomerular pressure Complex – 1000 – 1200 mL/min Macula densa – 45% hematocrit = 600ml/min plasma Glomerular Filtration Juxtaglomerular cells Rate – plasma filtration/minute – 120ml/min Filtration fraction = filtrate/total plasma perfusion per minute Afferent – 120ml/600ml = 20% arteriole – nearly all is Reabsorbed back into the blood, 1-2ml per minute left Glomerular Filtration Rate The rate of glomerular filtration (GFR) is important clinically for assessing kidney health, kidney disease and kidney failure The total rate of filtration at the glomerular membrane depends on net filtration pressure properties of the glomerular membrane, such as pore size, filtration slit size Total for the entire system, daily average GFR = 115- 125 mL/min 11 GFR Regulation GFR can be altered by: Plasma protein concentration ( affects Pop) Hydration level (affects Pglom and PBC) Urinary tract obstruction (PBC) Mean Arterial Blood Pressure (MAP affects Pglom) MAP is the most variable and will cause the biggest changes, so there are 2 ways the kidneys compensate for MAP 1)Autoregulation – Myogenic mechanism – Tubuloglomerular feedback 2)Sympathetic nervous System 12 Afferent & Efferent Arteriole Changes to the afferent and efferent arteriole can affect renal perfusion (RPF) and glomerular filtration rate (GFR) –Afferent arteriole pressure toward the glomerulus constriction of afferent arteriole = decreased Pgc, decreased GFR –Efferent arteriole pressure leaving the glomerulus constriction of efferent arteriole = increased Pgc, increased GFR Any afferent and/or efferent arteriole constriction lower total renal perfusion (RPF) due to decreased overall bloodflow 13 Fig. 14-9, p. 515 Autoregulation Autoregulation is the main L u m e way kidneys maintain n o f B o w m constant GFR as mean a n ’ s c a p systemic arterial blood s u l e pressure (MAP) changes If GFR is too high: afferent Efferent arteriole arteriole vasoconstriction If GFR is too low: afferent arteriole vasodilation 2 ways this happens: Myogenic Mechanism G A fl fo m e re r e u n tl a r tc a e rp i l o l a e r i e s Tubuloglomerular feedback 14 Glomerulus Afferent arteriole Glomerular Efferent arteriole capillary blood pressure Net filtration Vasoconstriction pressure (decreases blood flow into the glomerulus) GFR (a) Arteriolar vasoconstriction decreases the GFR 15 Fig. 14-10a, p. 515 Glomerulus Afferent arteriole Glomerular Efferent arteriole capillary blood pressure Net filtration pressure Vasodilation (increases blood flow into the glomerulus) GFR (b) Arteriolar vasodilation increases the GFR 16 Fig. 14-10b, p. 515 Autoregulation: Myogenic – Myogenic L u m Mechanism: afferent e n o f B o w arteriole m a n ’ s c automatically a p s u l e constricts when stretched by Efferent increased MAP arteriole – Increased Pglom → afferent arteriole G l vasoconstrictio o m e r u l a r c a p i l l a r i e s n, decreases Pglom to balance Afferent arteriole GFR 17 Autoregulation: Tubuloglomerular Feedback – Tubuloglomerular L u m feedback: occurs at e n o f B o w juxtaglomerular apparatus m a n ’ s – ATP and adenosine released c a p s u l e by DCT cells (macula densa) if GFR is too high, causes Efferent afferent arteriole arteriole vasoconstriction (granular cells) – Increased Pglom → macula densa sense high salt and fluid flow G A – Macula densa → release ATP fl fo m e re r e u n tl a r tc a e rp i l o l a e r i e s and adenosine granular cells – Afferent arteriole vasoconstriction decreases Pglom to balance GFR Macula densa 18 Juxtaglomerular Apparatus Endothelial Lumen of cell Bowman’s capsule Efferent arteriole Smooth Distal muscle tubule cell Efferent Bowman’s arteriole capsule Juxtaglomerular Podocyte Afferent apparatus arteriole Glomerular Mesangial capillaries cell Macula densa Granular cells Distal tubule Juxtaglomerular Afferent apparatus arteriole 19 Fig. 14-11, p. 516 Sympathetic GFR Regulation Sympathetic Nervous System L u m e stimulation will decrease n o f B o w m GFR to decrease urine a n ’ s c a p volume and retain fluids s u l e (increase blood volume) SNS → afferent arteriole Efferent arteriole vasoconstriction Decreases Pglom, decreases GFR SNS → mesangial cells and podocyte cell contraction Decreases size of filtration slits, G A fl fo m e re r e u n tl a r tc a e rp i l o l a e r i e decreases GFR s 20 RAAS Pathway: Renin Release RAAS Pathway – NET effect: increase systemic arterial pressure, increase sodium reabsorption – Renin released by JG (granular) cells of afferent arteriole when: afferent arteriole senses decreased blood pressure (less stretch) macula densa in DCT sense decreased sodium chloride SNS stimulates β- adrenergic receptors on JG cells release of prostaglandins RAAS Pathway RAAS Pathway – Renin: cleaves angiotensinogen angiotensinogen produced by liver – Angiotensinogen converted to angiotensin I – Angiotensin I converted to angiotensin II ACE enzyme from pulmonary and renal endothelium – Angiotensin II stimulates aldosterone release NaCl reabsorption at distal convoluted tubule and collecting duct increases systemic vasoconstriction, increases efferent arteriole vasoconstriction also: stimulates thirst and water reabsorption via ADH increase – increased blood volume and increased blood pressure Fig 37-10 Nephron Functions Major functions of the Nephron: – Glomerular Filtration – Tubular Reabsorption – Tubular Secretion – Excretion Basic process of Nephron Filtration Glomerular Filtration: separation of blood cells, proteins from solutes/fluid what stays in blood: proteins, blood cells blood in from afferent what goes into arteriole to glomerulus filtrate enters Bowman’s Filtrate: any capsule molecules smaller filtered blood leaves at than 3nm; water, efferent arteriole filtrate moves into PCT glucose, amino acids, nitrogenous waste The Filtration Membrane filtration slits The filtration membrane is 3 podocytes layers: – 1) glomerular epithelium: » fenestrated capillaries; blocks red and white blood capillary cells – 2) basement membrane » blocks proteins, other solutes can pass – 3) podocyte filtration slits; glomerular capillaries » blocks macromolecules » layer of anionic proteins podocytes blocks negatively charged proteins Mesangial cells: regulate blood flow, clear debris via phagocytosis Glomerular Filtration Rate Glomerular Filtration Rate (GFR): rate at which fluid enters the tubules from the glomerular capillaries used to evaluate kidney function, assess the severity and course of renal disease varies with age, body size, gender Women: 90 - 100 mL/min OR 130 - 145 L/day Men: 115 - 125 mL/min OR 165 – 180 L/day National Kidney Foundation, 2017 Clinical Estimation of GFR Clinical Estimation of GFR uses the principles of plasma clearance to track filtration of certain molecules – plasma clearance: rate at which a molecule is removed from the plasma and excreted in the urine (measured in mL/min) For GFR estimation, the ideal molecule should be: – stable plasma concentration – freely filtered at glomerulus – NOT reabsorbed, secreted, synthesized or metabolized by the kidney GFR = [Umol x V] / Pmol Examples: inulin GFR = glomerular filtration U= concentration of molecule in urine (mg/mL) creatinine V= urine flow rate (mL/min) P=plasma concentration Creatinine Creatinine: breakdown product of creatine, muscle metabolism simple, routine, inexpensive method Does it pass the clearance requirements? – stable plasma concentration ✔yes – freely filtered at glomerulus ✔yes – NOT reabsorbed, secreted, synthesized or metabolized by the kidney ** some creatinine secreted into PCT leads to about 10-20% higher excretion over and above filtration – may overestimate GFR by 10-20% Estimation of GFR: Creatinine Clearance GFR = [Ucr x V] / Pcr Creatinine Clearance ~ GFR Normal value, women: 95 ± 20mL/min Normal value, men: 120 ± 25mL/min Measure: 24 hour urine collection for volume & creatinine concentration plasma creatinine: measured from venous blood ***Be wary of values that are far out of range*** – ERROR? incomplete urine collection by patient? – ERROR? muscle mass? declines with age, muscle wasting, malnutrition increases with high meat diets Estimation of GFR: Plasma Creatinine Plasma Creatinine: rate of creatinine production by muscle is constant creatinine should leave the plasma as it is cleared by kidneys – decrease in GFR will lead to increased plasma creatinine accumulation women: 0.6 – 1.0 mg/dL men: 0.8 – 1.3 mg/dL age, gender, weight dependent** Blood Urea Nitrogen (BUN) Blood Urea Nitrogen: formed by liver metabolism of amino acids, conversion of ammonia into urea simple, routine, inexpensive method Does it pass the clearance requirements? – stable plasma concentration ** varies with high protein diet, muscle breakdown – freely filtered at glomerulus ✔yes – NOT reabsorbed, secreted, synthesized or metabolized by the kidney ** about 50% reabsorbed by PCT underestimates GFR reabsorption is passive and increases with sodium and water reabsorption (ex: during volume depletion) Glomerular Filtration Rate: Regulation GFR Depends on: 1) Total Surface Area of glomerulus 2) Permeability of Filtration Membrane 3) NFP: Net Filtration Pressure Surface Area & Permeability of Filtration Membrane High surface area due to tightly packed glomerular capillaries glomerular mesangial cells nearby can contract to reduce total surface area available for filtration Permeability due to fenestrations of glomerular capillaries 1000 times more permeable than normal body capillaries contraction of podocytes to decrease permeability? Glomerular Filtration Pressure Glomerular Filtration is regulated by various pressures at the glomerular membrane, where capillary blood pressure is the main driving force: Pglom Glomerular capillary blood pressure = +55mmHg – systemic blood pressure – Elevated glomerular capillary BP due to diameter of afferent arteriole (large) vs. efferent arteriole (smaller) Pop Plasma-colloid osmotic pressure = -30mmHg – high protein level in glomerular capillaries vs. Bowman’s capsule PBC Bowman’s capsule hydrostatic pressure = - 15mmHg – Fluid in Bowman’s capsule – The sum of these pressures is the: NET glomerular filtration pressure = +10mmHg 35 Reabsorption Tubular Reabsorption: returns necessary molecules from the tubules back to the blood through active Peritubular capillary and passive transport – Returned to blood: nutrients; glucose, filtrate enters PCT amino acids, water, substances selected to be returned to peritubular capillaries ions filtrate moves into Loop of Henle Na/K ATPase Na+/K+ ATPase sets up gradient across the tubule cell maintains Na+ concentration gradient (high in tubule lumen, low in tubule cell) maintains electrical gradient (inside of tubule cell is negative) Then… the gradient is used to drive movement of molecules Na+ transporters move Na+ from tubule lumen across apical membrane – Examples: » Na+/Glucose cotransporter » Na+/H+ exchanger » Na+ channel Fig 1.2 Rennke & Denker, 2014 Na+ Linked Processes The movement of many other molecules are dependent upon: Na+ gradients are linked to movement of: molecules that are directly linked to co-transporters, require Na+ to be reabsorbed (“piggy-back” molecules) molecules that are directly linked to exhangers, require Na+ to be secreted (“swap” molecules) molecules that require electrical gradients set up by Na+ osmolarity water follows Na+ other molecules become more concentrated as Na+ and water are removed from the filtrate Fig 1.2 Rennke & Denker, 2014 Nutrient Tmax – when transporters are used, the amount of nutrient that can be reabsorbed is limited by the number of transporters in the membrane Tmax = mg/min , how much a solute can be transported every minute substances stay in filtrate, excreted when transporters are “full”/saturated – Glucose = normally A LOT of transporters, all should be reabsorbed Filtrate Nucleus Interstitial Peri- in tubule fluid Diabetes lumen is abnormal case, tubular when Tmax of glucose capillary Tubule cell is reached glycosuria – Unrecognized Na solutes? = no transporters, no 3Na 3Na reabsorption 2K 2K Glucose K Amino acids Some ions Vitamins Tmax & Renal Threshold When active transporters or passive carriers are used to d move molecules, there is a Movement of glucose re lte limit to the amount they can Fi (mg/min) transport. Reabsorbed Tmax is the maximum amount of ed movement across the et membrane available for a given cr Ex molecule due to number of transport or carrier molecules Renal Threshold: When Plasma concentration of glucose plasma concentration of (mg/100 ml) substance exceeds ability of carries then it will be “excess” that stays in filtrate, lost in urine Renal Threshold Levels The Renal Threshold sets the amount of a given substance in the blood and in the urine. 2 typical examples: Glucose: renal threshold is very high compared to normal glucose intake – glucose is entirely reabsorbed – exception: uncontrolled diabetes mellitus, excessive blood sugar levels Phosphate: renal threshold is equal to normal PO4 intake – excess PO4 is quickly eliminated – PTH adjusts the renal threshold for phosphate Diluting and Concentrating Urine – kidneys adjust the amount of water and ions in the blood by adjusting the amount of water and ions lost in the urine via loop of Henle & vasa recta interactions – dehydration need to compensate with concentrated, small volume of urine – overhydrated need to compensate with dilute, large volume of urine Medullary Osmotic Gradient – The medulla contains a large osmotic gradient from 300mOSm in the cortex to 1200 mOsm in the inner medulla 300 300 400 The osmotic gradient: 600 The osmolality (solute concentration) of the 900 medullary interstitial fluid progressively 1200 increases from the 300 mOsm of normal body fluid to 1200 mOsm at the deepest part of the medulla. The Loop of Henle creates the gradient countercurrent multipliers: flow of filtrate down, then Water leaves the up the loop of Henle descending limb Interstitial fluid osmolality creates fluid flow and exchange. Descending limb is H2O NaCI Start permeable to water, here Osmolality of filtrate Salt is pumped out water leaves and filtrate in descending limb of the ascending limb becomes concentrated inside the loop of Henle Ascending limb is NOT permeable water, Osmolality of pumps out NaCl without filtrate entering the allowing water to ascending limb balance Vasa Recta Vasa recta preserve the gradient. preserve the The vasa recta are highly permeable to water and solutes. Countercurrent exchanges occur between each section of gradient the vasa recta and its surrounding fluid. As a result: The blood within the vasa recta remains nearly isosmotic to the surrounding fluid. The vasa recta are able to reabsorb water and solutes into the general circulation without undoing the osmotic gradient created by the countercurrent multiplier. blood in vasa recta Blood from To vein equilibrates with the 300 efferent arteriole medulla gradient and NaCI 300 325 NaCI maintain the osmotic H2O 300 H2O 400 400 differences The counter- current flow countercurrent NaCI H2 O 400 NaCI H2O of fluid moves through two adjacent parallel exchange: vasa recta 600 600 sections of vasa recta. blood flow turns up and NaCI NaCI 600 around the loops of H2O H2O henle, exchanging NaCl 900 900 NaCI NaCI and water along with H2O 900 H2O the established gradient 1200 Vasa recta 1200 Filtrate changes in Loop of Henle Reabsorption of water, then NaCl occurs through the Loop 300 300 100 of Henle 100 Descending limb: permeable to 300 water, filtrate loses water as it H2O NaCI moves further and further down H2O NaCI the medulla concentration 400 200 H2O NaCI gradient Filtrate concentrated, water H2O NaCI 600 400 returned to blood H2O Ascending limb: NOT NaCI permeable to water, actively H2O 900 700 pumps out NaCl, filtrate loses H2O NaCl as it moves up the medulla concentration gradient Nephron loop 1200 Filtrate iso-osmotic, NaCl returned to medulla and blood © 2016 Pearson Education, Inc. As water and solutes are reabsorbed, the loop first concentrates the filtrate, then dilutes it. 300 300 100 Cortex 100 300 300 1 Filtrate entering the 5 Filtrate is at its most dilute nephron loop is isosmotic H2O NaCI as it leaves the nephron loop. to both blood plasma and At 100 mOsm, it is hypo- cortical interstitial fluid. osmotic to the interstitial fluid. Osmolality of interstitial fluid (mOsm) H2O NaCI 400 400 200 H2O NaCI 4 Na and Cl– are pumped out Outer of the filtrate. This increases the medulla H2O NaCI interstitial fluid osmolality. 600 600 400 2 Water moves out of the H2O filtrate in the descending limb down its osmotic gradient. NaCI This concentrates the filtrate. H2O 900 900 700 H2O 3 Filtrate reaches its highest concentration at the bend of the Inner loop. medulla Nephron loop 1200 1200 © 2016 Pearson Education, Inc. Collecting Ducts use the medulla Gradient The Loop of Henle establishes and maintains the medulla gradient Filtrate is dilute when it reaches the DCT (100mOsm) Collecting Duct can remove water from 300 the urine if needed: Hydration state: 100mOsm, 300 dilute urine excreted 400 Dehydration state: urine needs can be concentrated up to 600 1200mOsm requires ADH: aquaporin 900 channels Low Blood Pressure states: 1200 urine output reduced to retain blood volume uses aldosterone: Na+ channels © 2016 Pearson Education, Inc. Collecting Ducts without water channels Collecting duct is NOT Collecting duct Descending limb permeable to water without 300 of nephron loop 100 hormonal mechanisms in place, DCT 100 filtrate is “blind” to medulla Cortex 300 100 300 Osmolality of interstitial fluid (mOsm) gradient NaCI H2O NaCI 600 400 600 Hydration state: 100mOsm, Outer medulla 100 dilute large volume of urine 900 NaCI excreted 700 900 H2O Urea Inner Diuretics: prescribed for high medulla 1200 100 1200 blood pressure, block hormonal Active transport Large volume of dilute urine mechanisms, block medullary Passive transport gradient formation, dilute, large volumes of urine excreted © 2016 Pearson Education, Inc. Collecting Ducts with Aquaporins Collecting duct H2O Descending limb 300 of nephron loop 100 150 H2O ADH: hormone that causes DCT 300 aquaporins to be inserted into Cortex Osmolality of interstitial fluid (mOsm) 300 100 300 300 collecting duct, water is NaCI H2O reabsorbed as filtrate moves H2O 400 through the medulla gradient H2O NaCI 600 400 600 600 Outer Normal state: water is reabsorbed medulla H2O from collecting duct to peritubular 900 NaCI 700 Urea 900 900 capillaries up to 1200mOsm H2O maximum H2O Urea Inner H2O medulla 1200 1200 99% of water initially filtered 1200 returned to blood Small volume of Urea contributes to the concentrated urine osmotic gradient. ADH small volume per day water lost in increases its recycling. urine NOTE: alcohol directly blocks ADH release © 2016 Pearson Education, Inc. Secretion Tubular Secretion: selectively adding to the filtrate by removing molecules from the blood Peritubular capillary – Excess in blood, needs excretion: metabolic waste (creatinine, etc), plasma protein bound any substances that have not drugs, acid (H+) or base been able to filter out, or have (HCO3-), ions (K+), urea been reabsorbed passively will be removed from the blood and put into the filtrate Tubular Secretion: K+ – K+ secretion is regulated to maintain electrical gradients, especially in the heart, and maintain Na/K pump activity in the kidneys – Secretion occurs in the DCT and Collecting Duct Na/K Pump, moves K+ into tubules K+ leaky channels in DCT and collecting duct lumen – Secretion stimulated by aldosterone Tubular Secretion: H+ – H+ secretion is regulated in order to maintain acid/base balance – Normally causes urine to be H+ acidic pH = 6 – occurs in mainly in PCT and HCO3– Collecting Duct H+ ATPase pumps H+ H+ H+/K+ ATPase pumps HCO3– Na+/H+ cotransporters; H+ antiporters HCO3– – Balanced with HCO3- formation and reabsorption to blood Proximal Convoluted Tubule Reabsorption: 50% of sodium 50% of water – aquaporins in apical and basolateral membrane – paracellular movement across “leaky” tight junctions Na+-linked Cotransporters – Na+-glucose – Na+-phosphate – Na+-amino acids – Na+-organic solutes Osmolarity linked passive diffusion – urea – potassium – calcium Secretion: Na+-linked H+ exchange – Na+-H+ exchanger– sodium reabsorption, H+ Fig 1.2 secretion Rennke & Denker, 2014 Micturition Bladder fills progressively until its wall tension (pressure) rises above threshold. This elicits a nervous reflex called the micturition reflex that empties the bladder or at least causes a conscious desire to urinate. Micturition reflex is completely autonomic spinal cord but it can be Inhibited or facilitated by Brain centers Brain centers include – Pontine facilitative and inhibitory centers – Several cerebral cortex mainly inhibitory centers Urinary Bladder and Its Innervations Blood vessels and have little to do with bladder contraction. Some sensory nerve fibers also pass by way of the sympathetic nerves and may be important in the sensation of fullness and, in some instances, pain. Ureterorenal reflex Ganglion cells 40 to 60 mm Hg (Hypogastric Nerves) low-resistance electrical Mot N pathways (Pelvic Nerves)Sen N Sacral Plexus Skeletal motor fiber Somatic nerve fiber Brain control of Micturition Micturition reflex is the basic cause of micturition but higher centers exert final control. Higher centers keep the micturition reflex partially inhibited except when micturition is desired Higher centers can prevent micturition even if micturition reflex occurs by continual tonic contraction of external urethral sphincter until convenient time presents. When time is convenient cortical centers can facilitate sacral micturition centers to initiate a reflex and inhibit the external sphincter so urination can occur Voluntary urination Voluntarily contracts abdominal muscle which increases pressure in the bladder More urine flows to bladder neck and posterior urethra thus stretching their wall This stimulates stretch receptors which excites micturition reflex and simultaneously inhibits external urethral sphincter Ordinarily all urine will be emptied with rarely more than 5-10 ml left in bladder. Adult Micturition Reflex Diagram Hemodialysis The End