Piston Engines Lec 1-3 PDF
Document Details
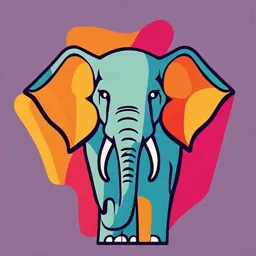
Uploaded by GreatestFarce
Tags
Summary
This document provides an introduction to piston engines, focusing on fundamental concepts and principles of operation. It details different types of engines, including four- and two-stroke cycle engines, and discusses aspects such as operating principles, valve operation, and classifications.
Full Transcript
Piston Engine Fundamentals DT011-1 Aircraft Engines 1 16.1 Fundamentals Four-Stroke and Two-Stroke Cycle Engines......................... 2 Introduction................................................. 2 Fundamenta...
Piston Engine Fundamentals DT011-1 Aircraft Engines 1 16.1 Fundamentals Four-Stroke and Two-Stroke Cycle Engines......................... 2 Introduction................................................. 2 Fundamentals of Engine Parameters............................. 5 Operating Principle........................................... 6 Valve Operation............................................ 11 Two-Stroke Cycle Systems.................................... 15 Principles of Diesel Engine Operation........................... 18 Classification of Reciprocating Engines.......................... 21 Designation of Reciprocating Engines........................... 26 Firing Order and Ignition Interval............................... 28 16.Contents - I Piston Engine Fundamentals Four-Stroke and Two-Stroke Cycle Engines The engine (or: power-plant) being discussed in this Subject is of a basis recipro- cating type. It includes a number of fundamental accessories which contribute to Introduction its operation. These accessories, carefully designed for the particular engine, in- clude the: Internal Combustion Engines carburettor/fuel injection system An engine may be defined as a machine in which heat energy released from burn- ignition coil and distributor ing fuel is transformed into mechanical energy, this burning is known as combus- battery tion. spark plugs Originally, the word engine had a wide variety of meanings. At one time, war and starter torture machines were known as engines. generator Even today, we call fire-fighting equipment fire engines. In this case, the term re- A failure of any one or a pronounced variation in their operation may cause loss of ferred to the steam engine that operated the pumps. Today, the word is usually power, poor operation, or even engine failure. limited to steam-, air-, gas- and oil-operated power units. Engines are classified according to the following: The internal combustion engine can burn fuels, such as gasoline, diesel oil, kero- the source of power: gas, oil or steam sene, fuel oil, alcohol and even coal dust. Two principal types of internal combus- the place where heat is released, whether inside or outside the cylinder, i.e. tion engines are the gasoline and diesel engines. The gasoline engine burns internal or external combustion engines gasoline and uses an electric spark to ignite the fuel. The diesel engine uses a the movement of the main working parts: reciprocating, rotary or turbine heavier petroleum product, reasonably enough, called diesel oil. In this type of en- the general arrangement of some characteristic features, such as vertical, gine, ignition is produced by heat from compression. Engines are driven by the horizontal, inclined or radical cylinders. combustion of liquid or gas fuels. They convert the chemical energy of the fuel into mechanical energy, producing rotary motion. The combustion pressure moves the The most common in the general aviation are the reciprocating internal combus- piston and the connecting rod transmits this power to the crankshaft. To achieve tion engines. greater transmission capacity several cylinders are incorporated into one engine. Engines that burn fuel in the chambers of the cylinders are known as internal com- The engine also requires many auxiliary systems, such as ignition, fuel supply, bustion engines. Those that burn fuel outside the cylinders, such as steam en- cooling, lubrication and exhaust systems. gines, are classified as external combustion engines. Note: In the following, only internal combustion engines will be discussed. C Piston Engine Fundamental Engine Working Parts The figure shows in a cut-away view an example of one of a four stroke cycle in- ternal combustion cylinder. To understand the working principles of internal com- Figure 1: Four-stroke Engine bustion engines it is necessary to know the names of a few parts. The basic combustion and gas expansion chamber is called the cylinder. It is es- sential that the cylinder is closed at one end by the cylinder head; the other end of the cylinder is open. The piston, equipped with rings, is close fitting and moves up and down or back and forth (reciprocating) in the cylinder. The rings are fitted into grooves around the piston to form a gastight seal between the piston and cylinder wall, normally two of these rings provide the lubricating oil film between the cylin- der wall and the piston. The open end of the cylinder is directly above the crankshaft (crank); it is support- ed by, and rotates freely in, suitable bearings. In order to convert the reciprocating motion of the piston into rotating motion, the piston is connected to the crankshaft by a connecting rod. This rod is fastened to the piston by the piston pin and to a bearing surface on the crankshaft by a split bearing. This bearing surface is re- ferred to as crankshaft throw, crankshaft journal, or crankpin. Each movement of the piston from top to bottom or bottom to top is called a stroke. One complete revolution of the crankshaft occurs with two strokes of the piston: one up and one down or, in the horizontal engine, one in and one out. When the piston is at the top of its stroke, it is said to be at top dead center (TDC); when it is at the bottom of its stroke; it is said to be at bottom dead center (BDC). Every engine must have an intake valve to admit the fuel-air mixture and an ex- haust valve to permit the gases in the cylinder to escape. An example of the intake valve is shown in the figure. The exhaust valve is similar. During the 4 strokes, the valves open and close with a specific timing controlled by the camshaft. The valves are held closed by coil springs, the opening impulse is given by the camshaft and transmitted via the push rods and the rocker, after the opening the coil spring closes the valve automatically. The cylinder head also includes the spark plug for igniting the mixture. Many engines have their cylinders lying in a horizontal or radial (circular) position, but the operation is the same, and the piston positions are identified the same way. Piston Engine Rotary Internal Combustion Engines (Wankel Engine) Figure 3: Wankel Engine Principle The Wankel engine principle is a internal combustion engine which uses a rotary design piston instead of a reciprocating one. The engine works after the four- stroke cycle principle and the piston has the rough shade of a triangle (epitro- choid). The rotary piston rotates around the center driveshaft (eccentric shaft) which is supported and fixed with bearings. The energy of the combustion is directly trans- ferred into a rotary motion on the eccenter shaft. The eccenter shaft is the center of gravity and the oscillating masses are reduced compared to a traditional piston engine, because of that the engine has a very sta- ble and smooth running with few vibrations. Figure 2: Four-Stroke Rotary Engine (Wankel principle) C Piston Engine Fundamentals of Engine Parameters Piston Displacement Piston displacement is defined as the total volume of air/fuel mixture an engine can Efficiencies draw in during one complete engine cycle; it is normally stated in cubic centime- ters, liters or cubic inches. In a piston engine, this is the volume that is swept as Volumetric Efficiency the pistons are moved from top dead center to bottom dead center. In a standard Volumetric efficiency (VE) is used to describe the am bount of fuel/air in the cylin- piston engine (an Otto or Diesel engine), displacement is calculated by multiplying der in relation to regular atmospheric air. If the cylinder is filled with fuel/air at at- the number of cylinders in the engine with the area of a piston and the length of mospheric pressure, then the engine is said to have 100% volumetric efficiency the stroke. (this means that the volume is totally filled with air at ambient pressure). On the With circular pistons, displacement can be calculated from the bore diameter and other hand, super chargers and turbo chargers increase the pressure entering the stroke using the following formula: cylinder, giving the engine a volumetric efficiency greater than 100%. However, if the cylinder is pulling in a vacuum, then the engine has less than 100% volumetric PD = A L N efficiency. Normally aspirated engines typically run between 80% and 100% VE. The higher the volumetric efficiency, the more power the engine can produce. where: Thermal Efficiency A = the area of the piston head in square inches L = the length of the stroke in inches Getting more fuel/air into the cylinder means more fuel energy is available to make power. Unfortunately, not all of the fuel’s energy gets converted to rotating energy. N = the number of cylinders in the engine. Of the 19,000 to 20,000 BTU "British Thermal Unit" per pound of gasoline, typically less than 33% ends up as useable horsepower. Compression Ratio The compression ratio is a single number that can be used to predict the perform- Note: One British Thermal Unit (BTU) is the amount of heat required to raise the ance of any internal-combustion engine. It is a ratio between the volume of a com- temperature of one pound (0.4 kg) of water by one degree Fahrenheit. bustion chamber and cylinder, when the piston is at the bottom of its stroke and Mechanical Efficiency the volume when the piston is at the top of its stroke. The higher the compression ratio, the more mechanical energy an engine can squeeze from its air-fuel mixture. Volumetric efficiency identifies how much fuel/air gets into the cylinder and thermal efficiency identifies how much of the fuel gets converted to useable power, but Higher compression ratios, however, also make detonation more likely. The ratio some of this power is robbed by the engine’s moving parts. It takes power to over- is calculated by the following formula: come the friction between parts and to run engine accessories such as the water pump. Depending on how much fuel gets into the cylinder and depending on how A L N + Vc -------------------------------------- much of the fuel gets converted to workable power, some of this power is used by Vc the engine to run itself. The left-over power is what could be measured on a dy- namometer (bench test). The difference between what is measured on the dyno where and the workable power in the cylinder is the mechanical efficiency (ME). A = the area of the piston head in square inches L = the length of the stroke in inches N = the number of cylinders in the engine Vc = volume of the combustion chamber (including head gasket). 16.1 - 5 Piston Engine Operating Principle The operations of reciprocating engines are based on the principles formulated by Dr. N. A. Otto in 1876. His principles are simply that if a mixture of fuel and air is compressed and then the fuel burnt in a confined space, the resulting pressure and consequent expansion would produce power. In the Otto process or cycle, air is the working medium or gas, although the vaporised fuel does add some mass to the working gas. The air contents only 20%, or 1/5, oxygen, while the other 80% is mainly nitrogen and other inert gases. It is the oxygen that combines chemically with the fuel in the combustion process which, in turn, supplies heat to the working medium - the 80% of the air that does not enter into the combustion process plus the products of combustion. The working medium acts just like steam in a steam engine. Not just the products of combustion, but the whole working medium or gases exert a force on the piston. Since fuel is a hydrocarbon, the products of combustion are carbon dioxide, water vapour, carbon monoxide, and some molecular hydrogen. There is a sequence of events, or a cycle, necessary for the practical application of these principles These events must be: 1. admission of a fuel-air mixture into a closed space 2. compression of this mixture to less volume 3. combustion of the fuel to produce expansion of the confined gases and power 4. exhaustion of the gases from the confined space before admission of a new fuel-air mixture. In a reciprocating engine, this cycle of events is accomplished in either two or four strokes of the piston. Note: The rotary engine (Wankel) works after the same principle but all 4 strokes happen in one rotation without empty strokes. 16.1 - 6 Piston Engine Figure 4: Four-Stroke Cycle Working Principle 16.1 - 7 Piston Engine Four-Stroke Cycle 1st Stroke = Intake - Suction or Induction The four-stroke engine has a crank mechanism which incorporates pistons, con- At the start of this stroke, the piston is at the top of the cylinder, and there is only necting rod and crankshaft. The crank mechanism is contained inside the engine a small space left between it and the cylinder head. This position, TDC, is control- casing which is divided into cylinder head, cylinder and crankcase. The vertical led by the crankshaft and connecting rod. A charge of fuel-air mixture must enter motion of the piston is converted into rotary motion via the connecting rod. Input this space. As the piston starts to move downward, the inlet valve is opened by the and output of fresh mixture and exhaust gases are via valves which are actuated camshaft. The exhaust valve remains closed. As the piston moves downward, a by a camshaft. The camshaft is driven at half crankshaft speed from the crank- partial vacuum is created in the space above the cylinder. Vacuum always intro- shaft, via timing wheels, timing chains or toothed belts. duces a potential suction effect. It is commonly said that the piston draws or sucks To produce a combustible fuel-air mixture, a carburettor or fuel injection system is the mixture into the cylinder. required. To achieve combustion an electric or electronic ignition system is also Atmospheric pressure pushes the fuel-air mixture through the inlet valve port into required. In the four-stroke cycle engine each upstroke and downstroke or back- the combustion chamber. After the piston has reached the bottom (BDC) of its and-forth movement of the piston is identified. These strokes are called intake, travel, controlled by the crankshaft and connecting rod, the space above the cylin- compression, power, and exhaust. The figure illustrates in schematic form the po- der is filled with a fuel-air mixture. Further movement of the crankshaft moves the sitions of some of the moving parts of the engine for each of these strokes. piston upward, and at the same time the inlet valve closes by spring action to trap The arrows along the sides of the cylinder are to show: the mixture in the cylinder. If the fuel were ignited at this point, it would burn, gen- the piston travel erating heat and causing an expansion of the gases. The resulting force would be the direction of rotation on the crankshaft for each of the four strokes. applied inside the cylinder and against the piston, but it is at the bottom of travel. Obviously, the development of pressure at this point is wasted. Remember that it takes two revolutions of the crankshaft to complete the four strokes, and that the stroke is the distance travelled by the piston from the top Figure 5: 1st Stroke dead center (TDC) position to the bottom dead center (BDC) position or back. The dead center is the top and bottom position where the piston changes direction. 16.1 - 8 Piston Engine 2nd Stroke = Compression 3rd Stroke = Power (Working or Expansion) As the crankshaft continues to rotate, the piston moves upward, reaching its ex- As the piston approaches top dead center, a spark occurs at the spark plug. Be- treme upward position. During this travel, the fuel-air mixture is compressed into a cause of the heat of the spark, the fuel is ignited and burns rapidly, or in other much smaller volume. Compressing the fuel-air mixture raises its temperature and words, combustion takes place. In this process, chemical energy is converted to pressure, thereby generating much more power than it would if the mixture is burnt heat. This combustion raises the temperature of all the gases in the combustion at atmospheric pressure only. Compressing or squeezing the fuel-air mixture in a chamber. Increasing the temperature of the gases causes an increase of pressure tightly enclosed chamber before ignition is very important. This is true if we wish and development of a force onto the piston. This pressure is applied equally on all to get the most heat of the fuel into work. At this TDC position on compression, the parts of the combustion chamber and piston head. Since the piston is free to move crankshaft has made one complete revolution, or 360°. downward, the force actually pushes it to the bottom of its travel. The connecting rod transfers this mechanical energy to the crankshaft, rotating it and doing the work. A flywheel mounted on the end of the crankshaft helps to keep the crank- shaft turning until power is again produced. The work of the crankshaft may be har- nessed through gears and shafts to drive equipment, such as the wheels of a vehicle. Figure 6: 2nd Stroke Figure 7: 3rd Stroke = Power (Work or Expansion) 16.1 - 9 Piston Engine 4th Stroke = Exhaust 1st Stroke After the fuel has burnt, it is necessary to clear the combustion chamber of the hot The exhaust valve is closed, the inlet valve open. The piston moves from TDC to gases to make room for a new charge of fuel-air mixture. The working gas must BDC and the volume above the piston is increased. As a result of the vacuum pro- be changed, since a new charge of oxygen is needed to support combustion of the duced, the fuel-air mixture is sucked in. The vacuum pressure is approx. 0.1 to 0.2 fuel. Just as the power stroke is nearly finished, the exhaust valve is opened by bar. the camshaft and the exhaust gases start to escape. As the piston moves upward on the last stroke of the cycle, the gases are pushed out of the cylinder or scav- 2nd Stroke enged to the atmosphere. At the end of the piston’s upward travel on the exhaust Both valves are closed and the piston moves from BDC to TDC. As the volume is stroke, all of the gases are practically removed from the cylinder. The exhaust reduced, the fuel-air mixture is compressed. The pressure increases from 10 to 16 valve is closed by spring action, and the camshaft again opens the inlet valve pre- bar, the temperature reaches approx. 400 - 500 °C. paratory to repeating the events of a cycle. The crankshaft has now gone all the way around again, another 360°, making a 3rd Stroke total of 720° of rotation. The four strokes are repeated over and over again, with a Both valves are closed, ignition occurs just before TDC and the piston is forced in different stroke taking place in each of the cylinders at any one time. The intake the BDC direction by the combustion of the mixture. Combustion is initiated by a valve and exhaust valve open once for each cycle, and they must be in time with spark at the plug. Since there is an interval between the spark and a flame being the stroke of the pistons. produced (approx. one millisecond) the ignition must be advanced 0 - 40° before TDC, so that the full combustion pressure of 40 - 60 bar is available shortly after Figure 8: 4th Stroke TDC has been reached. 4th Stroke The inlet valve is closed, the exhaust valve is opened. The piston moves from BDC to TDC and forces the exhaust gases out of the cylinder through the valve. The exhaust pressure is approx. two bar, and the temperature up to 1,000 °C. The spent gases are expelled from the cylinder at very high speed. 16.1 - 10 Piston Engine The following diagram shows the changes of pressure during the four strokes of Valve Operation the complete working cycle in one cylinder. According to the firing order of an en- gine, this working cycle occurs in each cylinder separately. To compare all working During our discussion of the four-stroke cycle, we assumed for convenience of ex- planation that the valves opened and closed on the dead center positions of the cycles of the cylinders in an engine, it is possible to measure the pressure pattern on a special test rig, while the engine is running, using a piezo-electric indicator piston. In actual operation, to improve engine operation and economy, the valves which produces an electrical tension in relation to the combustion chamber pres- are timed to open earlier and close later than the times described in the previous discussion. sure. Any marked deviation from the normal pattern of pressures suggests that the engine’s settings like mixture proportions, ignition timing or compression, are in- The engines are operated at relatively high speeds. And, to get the exhaust out of correct. the cylinder and a new charge in during the short time available, it is necessary to increase the number of degrees of crankshaft rotation during which the valves re- Figure 9: Complete Four-stroke Cycle Indicator Diagram main open. Valve Action The intake and exhaust valves on the engine are commonly opened by the cam- shaft and operating mechanism and closed by spring action. In this system, the camshaft is geared and timed to the crankshaft so that the valves will be operated at the right times. As the camshaft turns, the raised portion of the cam, called the lobe, raises the cam follower (valve lifter or tappet), which in turn pushes the valve off its seat. In the particular engine illustrated, the cam action of opening the valves is through push rods and rocker arms. The operation of the valves varies with different types of engines, but the principle of timing is the same. When the valve (intake or exhaust) is pushed off its seat, the valve spring is com- pressed. As soon as the lobe has cleared the tappet, the spring closes the valve. There are two cams for each cylinder, one for operating the intake valve and one for the exhaust valve. The lobes are not in the same position on the camshaft; if they were, both valves would be operated at the same time. Stress Distribution and Temperature During the Four-Stroke Cycle The figure show the formation of the working diagram to explain the pressure or stress distribution for the piston in a cylinder during the four strokes. The general technology uses the method of diagrams to demonstrate or compare different working cycles. 16.1 - 11 Piston Engine Figure 10: Cam Lobes, Lifters and Push Rod To explain the different degrees within the revolution of the crankshaft it is neces- sary to know the geometric figure of a circle and its partition in degrees. One full revolution stands for 360°. Therefore two revolutions are 2 x 360° = 720°. Half rev- olution is 180° and a quarter revolution is 90°. The camshaft must turn one-half as fast as the crankshaft in order to open each valve only once during two crankshaft revolutions; therefore, the camshaft gear is twice as large (has twice the number of teeth) as the driving crankshaft gear. That is, each valve must open once during every four strokes of the piston, because it is necessary to admit air and fuel once and to permit exhaust once. Figure 11: Timing Diagram with Respect to Crankshaft Rotation (I) 16.1 - 12 Piston Engine Valve Timing Figure 12: Valve Timing Diagram The correct valve timing is obtained by providing the proper rotating position be- tween the camshaft and crankshaft. Where the piston is near BDC and TDC the movement of the piston is very slight for several degrees of movement of the crankshaft. The solid lines show the positions of the connecting rod and piston TDC and BDC. The dotted lines indicate the position of the connecting rod and piston at approx. 30° before and after TDC and BDC. The piston movement is involved with all four events of the four-stroke cycle. Since little piston movement occurs near the top and bottom dead center positions, there is little work accomplished for that portion of connecting rod travel. This is called the rock position. Partly for this reason, the opening and closing of the valves do not have to coincide exactly with the beginning and ending of the four strokes of the cycle. The figure shows when the valves open and close for a typical engine. Remember the exact timing for a particular engine may be somewhat different from that in the illustration. The intake valves opens 5° of crankshaft rotation be- fore the piston has reached the TDC and closes 45° of crankshaft rotation after the piston has already passed BDC and moves upwards. Every half revolution of the crankshaft stands for 1 piston stroke. The intake valve is open during 230° of crankshaft rotation. The exhaust valve opens 45° before the piston has reached BDC and closes 5° after the piston has passed TDC. The exhaust valve is open during 230° of crank- shaft rotation. The exhaust valve opens before the piston reaches the end of the power stroke so that the pressure remaining in the cylinder will cause the hot gas- es to rush out on their own accord. If the valve did not open at this time, the remaining pressure would impede the up- ward movement of the piston during the exhaust stroke. Even though some of the force of the expanding gases is wasted, the gases rushing out the exhaust port create a turbulence which aids in scavenging the exhaust. This, together with the freedom afforded the piston in beginning the exhaust stroke, results in greater power output. Since both valves are open and closed the same amount of degrees during the crankshaft rotation and the four strokes, this diagram is a symmetrical valve timing diagram. 16.1 - 13 Piston Engine Figure 13: Timing Diagram with Respect to Crankshaft Rotation (II) 16.1 - 14 Piston Engine The early opening of the exhaust valve carries off some of the excess heat, thus Two-Stroke Cycle Systems aiding in cooling the engine. When the piston moves upward on the exhaust stroke, considerable momentum is imparted to the exhaust. But, if the valve closes The four events of the Otto cycle take place in a two-stroke engine, but the cycle is completed in one revolution of the crank. In both, the four-stroke and the two- at top dead center, a small amount of the gas will be trapped in the combustion chamber, and the amount of incoming fuel-air mixture will be somewhat less as stroke cycle, fuel and air are drawn into the engine, compressed, fired, and the ex- well as diluted. haust gases scavenged. The figure illustrates the principle of operation of a two- stroke cycle engine. The air current created by the exhausting gases is sufficient to cause a new fuel- The engine has no valves in the top of the cylinder and the inlet from the carburet- air charge to start moving into the cylinder if the intake valve is open. For this rea- son, the intake valve opens slightly before TDC. Further, by closing the exhaust tor opens into the crankcase through a slot. The path of the fuel charge into the valve after TDC on the intake stroke, the incoming charge under pressure helps to cylinder is through the transfer port in the side of the cylinder; opposite to this is the exhaust port. In the starting process of the engine, it is assumed that as the push the remaining exhaust out and also aids in cooling. Thus, both, the early opening and late closing of the exhaust valve result in better scavenging and cool- crank is turned the piston is moved upward. ing of the cylinder. When this happens, both ports, transfer and exhaust, are covered, and at the As the piston goes downward on the intake stroke, the decrease in pressure in the same time a partial vacuum is developed in the crankcase. A fuel-air mixture en- ters the crankcase because a pressure difference is created at the check valve. cylinder enables the atmospheric pressure to impart considerable momentum to the incoming mixture. With the intake valve remaining open until 45° past BDC, the Then as the piston moves down, it uncovers the two ports. The downward moving inward rush of the new charge is allowed a longer time interval, permitting more piston slightly compresses the mixture in the crankcase and forces the fuel charge through the transfer port and into the cylinder. charge to enter the cylinder. As cranking continues, the piston moves upward, closing the ports and compress- If the piston moves slowly, the quantity of mixture that enters will be such that its pressure inside the cylinder will be equal to that outside. At high speed, the piston ing the fuel-air in the cylinder. At the same time, a new charge is drawn into the reaches the end of its downward travel before a complete charge has had time to crankcase. Near TDC, the spark plug ignites the fuel to produce the power stroke. enter, thereby causing less initial pressure and resulting in less power output per As the piston then approaches BDC on the power stroke, the exhaust port is again stroke. uncovered, and because the exhaust is expanding, it is driven out through the port. Consequently, the valve is allowed to remain open during the rock position at BDC Further movement of the piston again uncovers the transfer port, and the new compressed fuel-air charge that entered the crankcase during the compression and somewhat beyond, since very little work is accomplished by the piston, to al- low as much fuel-air charge as possible to enter the cylinder. stroke is forced upward into the top of the cylinder. Also compression is realised until the piston is beyond the rock position; therefore, The task of the deflector on the piston is to direct the fuel-air mixture upward and having a more complete charge of fuel in the cylinder outweighs the small loss in away from the exhaust port. The incoming mixture aids in scavenging the exhaust gases. As the piston continues to move, more of the fuel-air mixture enters the cyl- compression that would be gained by having the intake closed at BDC. inder, and eventually the ports are closed and the events of compression accom- As it is shown, this is a typical valve timing diagram, such as would probably be panied by intake in the crankcase, power, and exhaust are repeated. found in a manufacturer’s service manual. This diagram shows a period of 10° of crankshaft rotation when the piston is in the top rock position at the end of the ex- haust stroke. Both valves are open; this is known as valve overlap. The amount of valve overlap is expressed in terms of degrees of crankshaft rota- tion while both valves are open. This will vary according to the design of the par- ticular engine. Valve overlap occurs only at the end of the exhaust stroke and at the beginning of the intake stroke, when the piston is in TDC position. 16.1 - 15 Piston Engine Figure 14: Two-stroke Cycle Engine Working Principle 1st Stroke Detail 1 - Piston moves up from BDC to TDC After the piston has closed the transfer port, a partial vacuum of 0.2 to 0.4 bar builds up in the crankcase as a result of the overall volume increasing. This is called the pre-induction phase. The top edge of the piston seals first the transfer- port then the exhaust outlet and compresses the fresh mixture which is ignited just before TDC is reached. Detail 2 - Crankcase fills up with fuel/air mixture When the piston exposes the inlet port, the actual inward flow of fuel-air mixture for the next working cycle commences. Fresh mixture is sucked in through the un- covered inlet port. 2nd Stroke Detail 3 - Piston moves down from TDC towards BDC The ignited mixture forces the piston downwards. The inlet port is closed by the bottom edge of the piston; the fresh mixture in the crankcase is pre-compressed. After the piston has closed the inlet port, pre-compression of the fuel-air mixture to approx. 0.3 - 0.6 bar commences. Detail 4 - Fuel/air mixture enters combustion chamber At the end of the power stroke the exhaust port is uncovered. Most of the spent gases flow into the exhaust pipe. The transfer port is opened, the pre-compressed fresh mixture flows from the crankcase into the cylinder and forces the remaining spent gases into the open exhaust outlet. In the two-stroke cycle petrol engine the gases are exchanged via ports in the cyl- inder wall, which are covered or uncovered by the piston. Timing mechanisms and valves are not needed. Engine lubrication is achieved by adding oil to the fuel. 16.1 - 16 Piston Engine Figure 15: Two-stroke Cylinder Case with Transfer Ports There are different kinds of scavenging processes. Since every downward stroke of the piston is a power stroke, it would seem that the two-stroke cycle engine is twice as efficient as the four-stroke engine. This is not true because of losses in three different ways: some of the charge goes out the exhaust port with the exhaust the transfer port is open for such a short time that a small amount of fuel-air mixture is admitted when the piston moves upward, there is no compression until the ports are covered. Also, operating temperatures are higher since combustion occurs at every revolu- tion. Consequently, two-stroke cycle gasoline engines are not used to a great ex- tent. Because of the design of the two-stroke cycle engine, the lubrication system is entirely different from that of a four-stroke cycle engine. The main difference between the four-stroke cycle and the two-stroke cycle en- gines is the control of the gas exchange. The two-stroke petrol engine works with- out valves, while the piston takes over that task to control the gas exchange. Therefore this engine does not have so many moving parts like camshaft and the complete valve train assembly with the valve mechanism. This makes a simple en- gine construction possible. Furthermore the two-stroke system uses the room above the piston and the room below the piston to fulfill the tasks of intake, com- pression, ignition and exhaust. The piston works in that case as a pump. The crankcase is completely closed and gastight. The two-stroke petrol engine does not need an oil change, because the oil is mixed together with the petrol and sep- Because three different kinds of channels or ducts are necessary for the gas flow, arator in the crankcase from the petrol for lubrication, while the petrol vapourises this engine is also referred to as a three-duct two-stroke engine. The most simple when it comes into contract with the hot engine parts. Later the oil will be burnt to- design of three-duct two-stroke engine with deflector piston has an inlet, an ex- gether with the petrol and fresh oil takes over the lubrication. The problem with the haust and a transfer duct. The inlet duct leads from the carburettor to the crank- two-stroke engine is the high fuel consumption. case. The exhaust duct leads to the exhaust pipe. The transfer duct connects the crankcase to the cylinder. The ducts terminate at slotted ports in the cylinder wall. The two-stroke engine uses an ’open’ gas flow principle, which means that both, the exhaust and transfer ports are open at the same time for most of the duration of the gas transfer process. In a four-stroke engine, on the other hand, the gas flow process is a closed one, apart from the valve overlap period when both, the inlet and the exhaust valve are open. As a result, some mixing of the fresh mixture and the burnt gases, with a loss of fresh mixture, is unavoidable in a two-stroke engine. The scavenging process largely determines the extent of these losses. 16.1 - 17 Piston Engine Principles of Diesel Engine Operation Four-Stroke Cycle in a Diesel Engine The diesel engine is mechanically similar to a gasoline engine, but it is of heavier Intake Stroke construction. Both depend for operation upon the four fundamentals of air, fuel, compression, and ignition. Examination of the four-stroke and two-stroke cycles On the downward stroke of the piston, with the exhaust valve still open, air is ad- will also reveal definite similarity between the gasoline and diesel engines. Intake, mitted into the cylinder through the intake valve. Atmospheric pressure forces the compression, power, and exhaust take place in the same order. air into the cylinder space vacated by the piston. The exhaust valve, like that in the gasoline engine, closes approx. 13° past TDC. Most diesel engines operate on the four-stroke cycle principle. The pistons move up and down in the cylinders, and connecting rods are fastened to a crankshaft Compression Stroke that transforms reciprocating motion into rotary motion. When air or any gas is compressed, its temperature raises. Compression starts at The valves (intake and exhaust) are also located in the cylinder head. There is little approx. 38° past BDC. Both valves are closed, and the volume in the cylinder difference in external appearance between the gasoline and diesel engines, and above the piston is decreasing. Piston movement compresses the trapped air to both types extract energy from burning fuel inside a cylinder. The main difference approx. 40 bar and raises the temperature of the compressed air to approximately between the two engines is in how the fuel is ignited. The fuel in a gasoline engine 800 °C. This heat is sufficient to ignite the diesel fuel spontaneously as it is inject- is ignited with a hot spark, the Diesel engine instead ignites the gas/air mixture ed. No electric spark is required. only with the compression temperature, in the start phase a glow plug raises the temperature in the cylinder head till the self ignition temperature is reached. Power Stroke Near the end of the compression stroke, approx. 10° - 20° before TDC, the diesel fuel is injected into the hot, compressed air, the fuel injection continuing until ap- prox. 16° past TDC. The heat starts an immediate combustion of the fuel, which is in an atomised form. The increased pressure exerts a strong force on the combus- tion chamber walls, but the piston is driven downward on the power stroke. The normal combustion temperature is approximately 1.700 °C. Exhaust Stroke At approx. 38° before BDC on the power stroke, the exhaust valve opens and re- mains so until after TDC. The remaining force of the expanding gases starts the exhaust of the gases, which is further continued as the piston moves upward. The events in both, gasoline and diesel engines are the same, even to the scavenging of the exhaust gases by the inrush of new air on the intake stroke. 16.1 - 18 Piston Engine Figure 16: Working of a Four-stroke Diesel Engine 16.1 - 19 Piston Engine Speed and Power Control Figure 17: Two-stroke Cycle Engine Since ignition is by heat of compression, the time at which the fuel in a fuel-air mix- ture would ignite cannot be timed accurately in a diesel engine. Instead, air only is compressed, and the fuel is injected at the proper time to produce power. This in- jection process has another result, a slower burning with more even cylinder pres- sures than the more rapidly burning gasoline in a gasoline engine. Also, in the diesel engine a full charge of air enters the cylinder on each intake stroke. Be- cause the quantity of air is practically constant, only the amount of fuel injected de- termines the power output and the speed. As long as the amount of fuel injected is below the amount established by the manufacturer, there is always enough air in the cylinder to insure complete combustion. Two-Stroke Diesel Engine In the case of the two-stroke cycle diesel engine, the operation is the same as for the same type of gasoline engine, except that the diesel engine uses a blower to force in the new air charge. An injection of diesel fuel is made each time the piston reaches TDC. Just before BDC, ports are opened or uncovered on the side of the cylinder. Fresh air is blown in and forces the remaining exhaust gases out the ex- haust ports (valves) in the cylinder head. The blower is used to pre-compress the air. The crankcase is used as an oil sump, as in a four-stroke engine. There are no scavenging losses, since the spent gases are only scavenged with air. The in- take of air is controlled by the piston as it opens and closes the ports. The outflow of spent gases is controlled by one or more exhaust valves. 16.1 - 20 Piston Engine Classification of Reciprocating Engines Figure 18: Engines Classified according to Cylinder Arrangement Many types of reciprocating engines have been designed since the Wright Broth- ers first used their four-cylinder in-line engine to make aviation history. Conven- tional piston engines are classified according to a variety of characteristics, e.g. cylinder arrangement with respect to the crankshaft cooling method (liquid-cooled, air-cooled) number of strokes per cycle (two-stroke, four-stroke). Actually, all engines are cooled by transferring the excess heat to the surrounding air. In air-cooled engines, this heat transfer is direct from the cylinders to the air. In liquid-cooled engines, the heat is transferred from the cylinders to the coolant, which is then sent through tubing and cooled within a radiator placed in the air- stream. Most aircraft reciprocating engines are air-cooled. The most satisfiying classification, however, is by cylinder arrangement. This is the method usually em- ployed because it is more completely descriptive than the other classifications. Although some engine designs have become obsolete, the types most commonly constructed throughout the history of power plants are mentioned. Aircraft engines may be classified according to their cylinder arrangement with respect to the crankshaft as follows: RADIAL TYPE: – rotary-radial – static-radial – single row – double row – multiple row (or: ’corncob’) IN-LINE TYPE: – upright – inverted V-TYPE: – - upright DOUBLE V (or: fan) TYPE OPPOSED (or: flat) TYPE X-TYPE 16.1 - 21 Piston Engine Radial Engines Figure 20: Static-radial Engines The radial engine consists of a row (or rows) of cylinders arranged radially about a central crankcase. Radial engines have appeared in two forms: the rotary-radial and the static-radial. Rotary-Radial Engines During World War I the rotary-radial engine was used by all of the warring nations. It produced the greatest number of horsepower for its weight. The cylinders of this engine are mounted radially around a small crankcase and rotate with the propel- ler. Fins cut into the outside of the cylinders provide all of the cooling needed as the engine spins around. The torque and gyro effect of this large rotating mass made the aircraft difficult to control. This difficulty, together with problems with car- buration, lubrication and exhaust systems, limited the development of the rotary- radial. Figure 19: Rotary-radial Engine 16.1 - 22 Piston Engine Figure 21: Cross Section of a Double Row Radial Engine 16.1 - 23 Piston Engine Static-Radial Engines Double-Row Engines In the late 1920s the Wright Aeronautical Corporation developed a series of 5-, 7- A double-row radial engine resembles two single-row radial engines combined on and 9-cylinder static-radial engines that changed aviation. The reliability of these a single crankshaft as shown in the figure. The cylinders are arranged radially in engines was far greater than that of any other power plant up to this time. The ra- two rows, and each row has an odd number of cylinders. The usual total number dial engine made the long distance flights possible by which Lindbergh and others of cylinders used is either 14 or 18, which means that the same effect is produced awoke the world to the realisation that the airplane was a practical means of travel. as having either two seven-cylinder engines or two nine-cylinder engines joined on one crankshaft. A two-throw 180° crankshaft is used to permit the cylinders in each Single-Row Engines row to be alternately staggered on the common crankcase. That is, the cylinders A single-row radial engine has an odd number of cylinders extending radially from of the rear row are located directly behind the spaces between the cylinders in the the center-line of the crankshaft. The number of cylinders is usually between five front row. This allows the cylinders in both rows to receive ram air for the neces- and nine. The cylinders are arranged evenly in the same circular plane. All pistons sary cooling. The radial engine has the lowest weight-to-horsepower ratio of all the are connected to a single-throw 360° crankshaft, thus reducing the number of different types of piston engines. It has the disadvantage of greater drag because working parts and also reducing the weight. of the area presented to the air, and it also has some problems in cooling. Never- theless, the dependability and efficiency of the engine has made it the most widely Figure 22: Radial Engine Crankshaft with Connection Rods used type for large aircraft equipped with reciprocating engines. The radial engine was manufactured with as few as three cylinders and as many as 28 but it was in the higher horsepower applications that the radial engine proved most useful. Ra- dial engines were produced by the thousands and powered most of the military and airline aircraft until the birth of the turbine engine for these high power appli- cations. 16.1 - 24 Piston Engine In-Line Engines Figure 23: V-type and Opposed Type Piston Engine An in-line engine generally has an even number of cylinders although some 3- cyl- inder engines have been constructed. This engine may be either liquid-cooled or air-cooled and has only one crankshaft which is located either above or below the cylinders. If the engine is designed to operate with the cylinders below the crank- shaft it is called an ’inverted engine’. The in-line engine has a small frontal area and is better adapted to streamlining. When mounted with the cylinders in an in- verted position it offers the additional advantage of a shorter landing gear and greater pilot’s visibility. The in-line engine has a higher weight-to-horsepower ratio than most other engines. With increase in engine size the air-cooled in-line type offers additional handicaps to proper cooling. Therefore, this type of engine is, to a large degree, confined to low- and medium- horsepower engines used in light aircraft. V-Type Engines In the V-type engines , the cylinders are arranged in two in-line banks (rows) form- ing the letter ’V’ usually set 60° apart (other angles, e.g. 90° or 45°, may exist). Most of the engines have eight or twelve cylinders which are either liquid-cooled or air-cooled. There is always an even number of cylinders in each row. Since the two banks of cylinders are opposite to each other, two sets of connecting rods can operate on the same crankpin, thus reducing the weight per horsepower as com- pared with the in-line engine. The frontal area is only slightly greater than that of the in-line type. Hence, the engine cowling can be streamlined to reduce drag. If the cylinders are mounted above the crankshaft, the engine is known as the ’up- right V-type’. If the cylinders are mounted below the crankshaft, it is known as an ’inverted V-type’ engine. The V-12 engines (V-type engines with 12 cylinders) de- veloped in the World War II era (1939-1945) represent some of the highest horse- powers achieved with reciprocating engines. Today, they are likely to be found only on restored military and racing aircraft. Note: Cooling by air is difficult with V-type engines. 16.1 - 25 Piston Engine Opposed-Type Engines Designation of Reciprocating Engines The opposed-type engine has two banks of cylinders directly opposite to each oth- Some manufacturers use a code system to indicate the type and characteristics of er with a crankshaft in the center. The pistons of both cylinder banks are connect- the engine, followed by a numerical indication of displacement. ed to the single crankshaft. These can be mounted in either a vertical or horizontal The following letters usually indicate the type or characteristic shown: position. Liquid-cooled and air-cooled versions are currently being produced. The opposed-type engine is most popular for light conventional aircraft and helicopters L left-hand rotation for counter-rotating propeller and is manufactured in sizes from less than 100 HP (74.57 kW) to more than 400 T turbocharged with turbine-operated device HP (298.28 kW). These engines are the most efficient, dependable and economi- V vertical; for helicopter installation with the crankshaft in a vertical position cal types of piston engine available for light aircraft. H horizontal; for helicopter installation with the crankshaft in a horizontal The opposed-type engine is usually mounted with both the cylinders and the position crankshaft horizontal. However, in some helicopter installations the crankshaft is A aerobatic; fuel and oil systems designed for sustained inverted flight vertical. The engine has a low (good) weight-to-horsepower ratio. Because of its I fuel injected; continuous fuel injection system installed flat shape it is very well adapted to streamlining and horizontal installation in the G geared nose section for propeller rpm reduction nacelle. Another advantage is that it is reasonably free from vibration. S supercharged; engine structurally capable of operating with a manifold Summary pressure (MAP) of more than 30 in Hg (1,016.1 hPa) and equipped with either a turbine-driven supercharger or an engine-driven supercharger The radial engine has the disadvantage of a large frontal area which produces a great amount of drag. As airplane speeds increased the need to reduce this drag O opposed cylinders became increasingly important. The in-line engine has little frontal area and thus R radial engine; cylinders arranged radially around the crankshaft. little drag but its weight is a disadvantage. The V-type engine is a good compro- Note: Not all manufacturers follow this system. mise between weight and frontal area, but air-cooling is difficult. The horizontally- V-type engines and inverted in-line engine have such designations as V and I. In opposed air-cooled engine is such a good combination of low weight, small frontal every case, the technician working on an engine must interpret the designation area and ease of cooling that it has become the standard configuration. Today, vir- tually all production of reciprocating engine-powered aircraft use this type of en- correctly and utilise the proper information for service and maintenance. The 3-dig- it numbers in the second part of an engine’s designation indicate displacement to gine. The double-V (fan-type) engines have not been in use for many years. The only piston engines in extensive use at the present time are the opposed and radial the nearest 10 in3. E.g., an engine’s displacement of 471 in³ is indicated as 470’. types. A few V-type and in-line engines may still be found in operation, but these A system of suffix designations has also been set up to provide additional informa- tion about engines. The first suffix letter indicates the type of power section and engines are no longer manufactured for general aircraft use. the rating of the engine. This letter is followed by a number from one to nine, which gives the design type of the nose section. 16.1 - 26 Piston Engine Following the nose section number, there is a letter indicating the type of acces- Figure 25: Engine Plates with Designation Suffixes sory section. After this letter, there is a number which states which type of coun- terweight application is used with the crankshaft. Additionally, this number indicates the mode of vibration, such as ’4’, ’5’ or ’6’. The mode number will be found on the counterweights or dynamic balances on the crankshaft. The final character in the designation suffix may be a letter indicating the type of the mag- neto utilised with the engine. The letter ’D’ indicates a dual magneto. Figure 24: Designation Suffixes 16.1 - 27 Piston Engine Firing Order and Ignition Interval Powerful, high-speed engines have a fairly large number of cylinders. As a result, they run more smoothly and develop their torque more uniformly than single- cyl- inder engines, since the working cycles follow one another at shorter intervals. Furthermore, the working strokes or ignition pulses for the various cylinders are evenly distributed over both revolutions of the crankshaft needed for any single complete working cycle. For this reason, the interval between ignition pulses is 360° on a twin-cylinder engine, 180° on a four-cylinder engine, 120° on a six- cyl- inder engine and 90° on an eight-cylinder engine. ’Firing order’ in multi-cylinder engines refers to the power sequence or order in which the cylinders work. The ignition interval depends on the number of cylinders and gives the interval between power strokes in degrees of crankshaft rotation. The firing order is determined by the manufacturer’s engineers who design the en- gine. The relationship of the cranks, or throws, on the crankshaft and the cams and lobes on the camshaft regulate the desired firing order. The firing of engine cylin- ders and does not run consecutively through the total numbers, as 1-2-3-4 or 1-2- 3-4-5-6, and so on. For normal opposing engines with 4 or 6 cylinders the firing order is 1-3-4-2 for the 4 cylinder and 1-6-3-2-5-3 for the 6 cylinder. It has been found best to use the principle of stepping from the front of the engine The figure shows the firing interval of a two-°cylinder engine. The two pistons move up and down at the same time and are equal positioned in their cylinders. They fulfil the task of the four strokes according to their arrangements. As we have seen before, the piston has to move twice from TDC to BDC and twice from BDC to TDC, to complete one working cycle. The figure shows two pistons in the TDC position. Piston No. 1 will enter the power stroke while piston No. 2 enters the induction stroke. The ignition follows in an in- terval of every full revolution or 360°. If we follow the stroke order, we see piston No. 1 in the exhaust stroke and piston No. 2 in the compression stroke, piston No. 1 in induction while piston No. 2 is in the power stroke and piston No. 1 in com- pression, while No. 2 is in exhaust stroke. 16.1 - 28 Piston Engine Figure 26: Firing Order of Reciprocating Engines (I) 16.1 - 29 Piston Engine Figure 27: Firing Order of Reciprocating Engines (II) 16.1 - 30