Gas Exchange, Transport, and Circulation in Plants and Animals (Part 1) - January 2025 - Our Lady of Fatima University PDF
Document Details
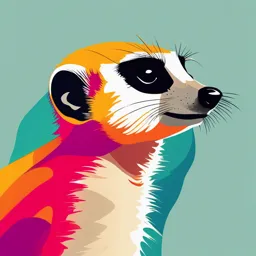
Uploaded by InvulnerableMotif3109
Our Lady of Fatima University
2025
Daniel Lance R. Nevado
Tags
Summary
This document is a lecture note/presentation from Our Lady of Fatima University. It covers the topic of gas exchange, transport, and circulation in plants and animals. The document is likely to contain lectures from the 2025 January session detailing the processes of gas exchange and animal physiology for biological science.
Full Transcript
WEEK 1: Gas Exchange, Transport, and Circulation in Plants and Animals (Part 1) January 2025 | Prepared by: Daniel Lance R. Nevado Learning Objectives: 1. Compare the structures or organs involved in gas exchange in plants and animals. 2. State factors that affect gas exchange. 3....
WEEK 1: Gas Exchange, Transport, and Circulation in Plants and Animals (Part 1) January 2025 | Prepared by: Daniel Lance R. Nevado Learning Objectives: 1. Compare the structures or organs involved in gas exchange in plants and animals. 2. State factors that affect gas exchange. 3. Explain the breathing mechanisms in vertebrates. 4. Trace the pathway of air in a mammalian respiratory system through the organs and their roles. 5. Explain the coordination of the respiratory system with the circulatory system in the transport of gases to the body tissues. Gas Exchange across Respiratory Surfaces One of the major physiological challenges facing all multicellular animals is obtaining sufficient oxygen (O2) and disposing of excess carbon dioxide (CO2). 4 Major Types of Gas Exchange Systems 1. Body surface (Invertebrates, adult amphibians) 2. Gills (Invertebrates, fish, larval amphibians) 3. Tracheae (Invertebrates) 4. Lungs (almost ALL amphibians, reptiles, Figure 1. Elephant seals are respiratory Champions birds, and mammals) Diving to great depths, elephant seals can hold their breath for over 2 hr, descend and ascend rapidly in the water, and endure repeated dives without suffering any apparent respiratory distress. Gas Exchange involves diffusion across membranes Because plasma membranes must In this case, oxygen from air dissolves be surrounded by water to be in a thin layer of fluid that covers the stable, the external environment in respiratory surfaces, such as in the gas exchange is always aqueous. lungs. The diffusion process is passive, driven only by the difference in O2 and CO2 concentrations on the two sides of the membranes and their relative solubilities in the plasma membrane. Fick’s Law of Diffusion for a dissolved gas, rate of diffusion (R) is directly proportional to the pressure difference (∆p), and the area (A) over which the diffusion occurs. R is inversely proportional to the distance (d) across which the diffusion must occur. Diffusion constant (D) accounts for the size of molecule, membrane permeability, and temperature. Figure 2. Different Gas Exchange Systems in animals a. Gases diffuse directly into single-celled organisms. b. Most amphibians and many other animals respire across their skin. Most amphibians also exchange gases via lungs. c. Echinoderms have protruding papulae, which provide an increased respiratory surface area. d. Insects respire through an extensive tracheal system. e. The gills of fishes provide a very large respiratory surface area and countercurrent exchange f. The alveoli in mammalian lungs provide a large respiratory surface area but do not permit countercurrent exchange. Inhaled fresh air contains some CO2, but levels are higher in the lungs, so more CO2 is exhaled than inhaled; similarly, O2 levels are higher in fresh air, leading to an influx of O2. Gills, Cutaneous Respiration, and Tracheal Systems Gills are specialized extensions of tissue that project into water. They can be simple, as in the papulae of echinoderms, or complex, as in the highly convoluted gills of fish. External gills are found in fish and amphibian larvae Figure 3. Some amphibians have External gills are not enclosed within external gills External gills are used by a body structures. aquatic amphibians, both larvae and some species Disadvantage: they must that live their entire lives in water such as this axolotl constantly be moved to ensure (Ambystoma mexicanum), contact with fresh water having to extract oxygen from the water. high oxygen content. Branchial chambers protect gills of some invertebrates Another disadvantage of external gills: thin epithelium for gas exchange can be easily damaged. Branchial chambers provide a means of pumping water past stationary gills. The internal mantle cavity of mollusks opens to the outside and contains the gills. In crustaceans, the branchial chamber lies between the bulk of the body and the hard exoskeleton of the animal. Figure 4. Body plans of a Bivalve and a Decapod crustaceans Gills of bony fishes are covered by the operculum The gills of bony fishes are located between the oral cavity (buccal cavity), and the opercular cavities which serves as pumps to alternately move water into the mouth, through the gills, and out of the fish. Figure 5. How most bony fishes respire. The gills are suspended between the buccal (mouth) cavity and the opercular cavity. Respiration occurs in two stages. The oral valve in the mouth is opened and the jaw is depressed, drawing water into the buccal cavity while the opercular cavity is closed. The oral valve is closed and the operculum is opened, drawing water through the gills to the outside. There are between three (3) and seven (7) gill arches on each side of fish’s head. Each gill arch is composed of two rows of gill filaments, and each gill filament contains thin membranous plates or lamellae. Water flows past the lamellae in one direction only. Figure 6. Structure of a fish gill Water passes from the gill arch over the filaments (from left to right in the diagram). Water always passes the lamellae in a direction opposite to the direction of blood flow through the lamellae. The success of the gill’s operation critically depends on this countercurrent flow of water and blood. Countercurrent flow – blood flows opposite to the direction of water movement in the lamella. Maintains a positive oxygen gradient along the entire pathway for diffusion Ensures that the oxygen concentration gradients remains between blood and water throughout the length of the gill lamellae Figure 7. Countercurrent exchange. This process allows for the most efficient blood oxygenation. When blood and water flow in opposite directions (a), the initial oxygen (O2) concentration difference between water and blood is small, but is sufficient for O2 to diffuse from water to blood. As more O2 diffuses into the blood, raising the blood’s O2 concentration, the blood encounters water with ever-higher O2 concentrations. At every point, the O2 concentration is higher in the water, so that diffusion continues. In this example, blood attains an O2 concentration of 85%. When blood and water flow in the same direction (b), O2 can diffuse from the water into the blood rapidly at first, but the diffusion rate slows as more O2 diffuses from the water into the blood, until finally the concentrations of O2 in water and blood are equal. In this example, blood’s O2 concentration cannot exceed 50%. Cutaneous respiration requires constant moisture Cutaneous respiration – the process of exchanging oxygen and carbon dioxide across the skin In amphibians, cutaneous supplements and sometimes replaces the action of lungs (such as in plethodontid salamanders “lungless salamanders”) Some aquatic reptiles have the ability to respire cutaneously such as soft-shelled turtles. They can remain submerged and inactive in river sediment for hours without having to ventilate their lungs. Figure 8. Plethodontid salamanders and soft-shelled turtles Tracheal systems are found in arthropods Tracheae– small, branched cuticle-lined air ducts (found in most terrestrial arthropods). The arthropods have no single respiratory organ. The trachea branched into very small tracheoles which are series of tubes that transmit gases throughout the body. Spiracle – specialized opening in the exoskeleton where air passes. In most terrestrial arthropods, spiracle can Figure 9. Tracheae and tracheoles be opened and closed by valves. Tracheae and tracheoles are connected to the exterior by openings called spiracles and carry oxygen to all parts of a Advantage: prevent water loss by closing terrestrial insect’s body. the spiracles for adaptation that facilitated the invasion of land by arthropods Lungs Despite the high efficiency of gills as respiratory organs in aquatic environments, gills were replaced in terrestrial animals for two principal reasons: 1. Air is less supportive than water 2. Water evaporates The fine membranous lamellae of Terrestrial organisms constantly lose gills lack structural strength and rely water to the atmosphere. on water for their support. Gills would provide an enormous When a fish is out of water, it will surface area for water loss, soon suffocates because its gills potentially causing dehydration. collapse into a mass of tissue. The lungs minimizes evaporation by Unlike gills, tracheae and lungs can moving air through a branched tubular remain open because the body itself passage. provides the necessary structural The tracheal system of arthropods also support uses internal tubes to minimize evaporation Breathing of air takes advantage of Amphibians and reptiles breath in partial pressures of gases different ways Partial pressure – the pressure The lungs of amphibians are formed as contributed by gas to the total saclike outpouchings of the gut. atmospheric pressure according to its fraction of the total molecules present. Dry air contains: Nitrogen – 78.09% Oxygen – 20.95% Argon – 0.93% Carbon dioxide – 0.03% 760 mm Hg (1.0 atm) is the barometric pressure of the air at sea level Amphibians force air into their lungs, filling their oral cavity with air and pushes air into their lungs. This is called positive pressure breathing Reptiles expand their rib cages by muscular contraction which creates a lower pressure inside the lungs compared with the atmosphere. This kind of ventilation is called negative pressure breathing because of the air being “pulled in” by the animal Figure 10. Amphibian Lungs Each lung of this frog is an outpouching of the gut and is filled with air by the creation of a positive pressure in the buccal cavity. a. The buccal cavity is expanded and air flows through the open nostrils. b. The nostrils are closed and the buccal cavity is compressed, thus creating the positive pressure that fills the lungs. The amphibian lung lacks the structures present in the lungs of other terrestrial vertebrates that provide an enormous surface area for gas exchange, and so are not as efficient as the lungs of other vertebrates. Mammalian lungs have greatly increased surface area Pathway of air: Mouth and Nose → pharynx → larynx (voice box) → glottis → trachea (windpipe) → right and left bronchi → bronchioles → alveoli The alveoli are surrounded by an extensive capillary network where all gas exchange between the air and blood takes place In humans, each lung has about 300 million alveoli, and the total surface area available for diffusion can be as much as 80 m2. Figure 11. The Human Respiratory System and the structure of the mammalian lung The lungs of mammals have an enormous surface area because of the millions of alveoli that cluster at the ends of the bronchioles. This provides for efficient gas exchange with the blood. Mammalian lungs have greatly increased surface area Pathway of air: Mouth and Nose → pharynx → larynx (voice box) → glottis → trachea (windpipe) → right and left bronchi → bronchioles → alveoli The alveoli are surrounded by an extensive capillary network where all gas exchange between the air and blood takes place In humans, each lung has about 300 million alveoli, and the total surface area available for diffusion can be as much as 80 m2. Figure 11. The Human Respiratory System and the structure of the mammalian lung The lungs of mammals have an enormous surface area because of the millions of alveoli that cluster at the ends of the bronchioles. This provides for efficient gas exchange with the blood. The respiratory system of birds is a highly efficient flow-through system The avian respiratory system has the most efficient respiration of all terrestrial vertebrates. The bird lung channels air through tiny vessels called parabronchi, where gas exchange occurs. Air flows through the parabronchi in one direction only. The unidirectional flow of air is achieved through the action of anterior and posterior air sacs unique to birds. Figure 12. How a bird breathes. Birds have a system of air sacs, divided into an anterior group and posterior group, that extend between the internal organs and into the bones. Figure 12. How a bird breathes. Breathing occurs in two cycles. Cycle 1: Inhaled air (shown in red) is drawn from the trachea into the posterior air sacs (shown expanding as it fills with air) and then is exhaled into the lungs (posterior air sacs deflate). Cycle 2: Air is drawn from the lungs into the anterior air sacs, which expand, and then is exhaled from these air sacs through the trachea. Passage of air through the lungs is always in the same direction, from posterior to anterior (right to left in this diagram). These cycles are always going on simultaneously; during inhalation, fresh air enters the posterior air sacs at the same time that air from the previous breath that was in the lungs moves into the anterior air sacs. In exhalation, the newer air moves from the posterior air sacs to the lung at the same time that air in the anterior air sacs is exhaled from the body. At the same time, another breath of inhaled air (purple) is moving through cycle 1. The respiratory pigments bind oxygen for transport Hemoglobin is a protein composed of four polypeptide chains and four organic compounds called the heme group. (Used most of the vertebrates) At the center of each heme group is an atom of iron, where oxygen bind. Each hemoglobin molecule can carry four oxygen molecules to form a complex molecule called oxyhemoglobin. Hb + 4O2 → Hb(O2)4 Hemoglobin Oxyhemoglobin Figure 13. The structure of the adult hemoglobin In hemocyanin, the oxygen-binding atom is protein. Hemoglobin consists of four polypeptide chains: two α copper and it is a free protein circulating via chains (blue) and two β chains (gold). Each chain is hemolymph of arthropods and some mollusks. associated with a heme group (white), and each heme group has a central iron atom (red ball), which can bind to a molecule of O2. Carbon dioxide is primarily transported as bicarbonate ion The carbon dioxide is transported by blood to the lungs in three different ways Conversion to hydrogen carbonate ions in red blood cells (72%) Binding to hemoglobin (20%) Dissolving in the plasma (8%) Figure 14. The transport of Carbon dioxide by the blood. Passage into bloodstream. CO2 is transported in three ways: dissolved in plasma, bound to the protein portion of hemoglobin, and as bicarbonate (HCO3–), which forms in red blood cells. The reaction of CO2 with H2O to form H2CO3 (carbonic acid) is catalyzed by the enzyme carbonic anhydrase in red blood cells. Carbon dioxide is primarily transported as bicarbonate In the lungs ion where the oxygen partial pressure is high and the carbon dioxide partial pressure is low, oxygen diffuses into the alveolar blood capillaries from the alveoli while carbon dioxide diffuses out from the alveolar blood capillaries into the alveoli and is expelled in exhaled air. Figure 14. The transport of Carbon dioxide by the blood. Removal from bloodstream. When the blood passes through the pulmonary capillaries, these reactions are reversed so that CO2 gas is formed, which is exhaled. Gas exchange in Plants Stomata is a pore or aperture that penetrates the epidermis of leaves, young branches, and stems of green plants. Plays an important role in the exchange of respiratory gases in plants Guard cells two specialized epidermal cells forming stomata. Bean-shaped cells that contains chlorophyll and are able to carry out photosynthesis Play an important role in the opening and Figure 15. Stomata closing of the stoma. A stoma is the space between two guard cells that regulates the size of the opening. Stomata are evenly distributed within the epidermis of monocots and eudicots, but the patterning is quite different. Gas exchange in Plants Lenticels are spongy areas in the cork surfaces of stem, roots, and other plant parts that allows interchange of gases between internal tissues and the atmosphere through the periderm. Figure 16. Lenticels a. Lenticels, the numerous small, pale, raised areas shown here on cherry tree bark, Prunus cerasifera, allow gas exchange between the external atmosphere and the living tissues immediately beneath the outer bark of woody plants. b. Transverse section through a lenticel in a stem of elderberry, Sambucus canadensis. Pneumatophores are spongy outgrowths from underwater roots which commonly extend several centimeters above water, facilitating oxygen uptake in the roots beneath. Figure 17. How mangroves get oxygen to their submerged parts. The black mangrove, Avicennia germinans, grows in areas that are commonly flooded, and much of each plant is usually submerged. However, modified roots called pneumatophores supply the submerged portions of the plant with oxygen because these roots emerge above the water and have large lenticels. Oxygen diffuses into the roots through the lenticels, passes into the abundant aerenchyma, and moves to the rest of the plant.