The Eukaryotic Cell Cycle PDF
Document Details
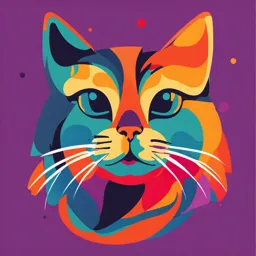
Uploaded by ResilientRocket
Tags
Summary
This document provides information on the eukaryotic cell cycle, including cell division, MPF, and stages of meiosis. It has an overview of cell cycle progression with a detailed description of cell processes.
Full Transcript
The Eukaryotic Cell Cycle Cell division ell division is controlled by: C 1. Nutrients 2. Cell size 3. Cell density 4. Surface attachm...
The Eukaryotic Cell Cycle Cell division ell division is controlled by: C 1. Nutrients 2. Cell size 3. Cell density 4. Surface attachment 5. Growth factors 1. Maturation promoting factor 1) How to identify MPF MPF was discovered in studies usingXenopus eggs: E arly development alternates betweenS phaseandM phasewithout G1 or G2. Early development: Zygote –> blastula –> gastrula Injection of cytoplasm frommitotic cellsinto non-mitotic oocytes induced mitosis. MPF was shown to regulate the entry intomitosis. Characteristics of MPF: M PF activity peaks duringmitosisand is low during interphase. Composed of two key components: 1. Cyclin B: Regulatory subunit. 2. CDK1: Catalytic subunit. Progesterone Treatment and the Xenopus Oocyte Cell Cycle ( a) Stepwise Process of Meiosis and Early Embryonic Development 1. Step 1: Progesterone Treatment or MPF Injection ○ Initial state:Xenopus oocytes are arrested in the G2 phase. ○ Progesterone treatment: Induces the oocytes to entermeiosis I. ○ Activity of MPF (Maturation Promoting Factor): Mimics the effect of progesterone to drive entry into meiosis I. ○ Chromosomal Changes in Metaphase of Meiosis I: Two pairs ofsynapsed homologous chromosomes(blue) align on themeiotic spindle(green). 2. Step 2: First Polar Body Formation ○ Segregation of homologous chromosomes: Homologs are separated, and an asymmetric cell divisionoccurs. ○ First polar body formation: Half the chromosomes are expelled into a small polar body. ○ Transition to Meiosis II: The oocyte immediately enters meiosis II and arrests inmetaphase II. ○ Chromosomal State in Metaphase II: Two chromosomes remain connected to the spindle microtubules. 3. Step 3: Fertilization and Release from Metaphase II Arrest ○ Fertilization by sperm: Triggers release from metaphase II arrest via a rise in intracellularCa²⁺. ○ Completion of Meiosis II: C hromatids segregate, and a second asymmetric cell divisionoccurs. Half the chromatids are expelled into the second polar body. ○ Formation of Zygote: The haploidfemale pronucleusfuses with the haploidsperm pronucleusto form a diploid zygote. 4. Step 4: DNA Replication and First Mitosis ○ The zygote undergoesDNA replicationand enters the first mitotic division. . Step 5: Synchronous Divisions to Form Blastula 5 ○ The first mitosis is followed by11 rapid synchronous divisions. ○ Results in the formation of ablastula. Step 1a: Transfer of Cytoplasm S ource:Cytoplasm is transferred from anegg arrested in metaphase of meiosis II(leftmost cell in the diagram). Target:The cytoplasm is injected into anoocyte arrested in G₂(middle cell). Purpose: ○ The cytoplasm containsMPF(Maturation Promoting Factor), which is a key driver of meiotic progression. ○ MPF triggers the transition of the G₂-arrested oocyte intomeiosis I. Step 2: Progression to Meiosis I A fter receiving MPF, the oocyte progresses fromG₂ arrest tomeiosis I: ○ Chromosomal Changes: Homologous chromosomes align on the spindle apparatus (green structures represent spindle microtubules). ○ T he oocyte undergoes segregation of homologous chromosomes during this phase. Step 3: Arrest at Metaphase of Meiosis II Following meiosis I: ○ The oocyte progresses tomeiosis IIbut arrests at metaphase of meiosis II. ○ Reason for Arrest: The arrest is mediated by a high level of MPF, which stabilizes the metaphase state. ○ This stage is typical of mature eggs awaiting fertilization. Key Molecular Insights Highlighted by the Diagram: 1. Role of MPF: ○ MPF is sufficient to trigger the transition from G₂ to meiosis I and subsequent progression to meiosis II. ○ High MPF activity maintains metaphase II arrest until fertilization. 2. Cytoplasmic Regulation: ○ The cytoplasm from an egg in metaphase II contains critical factors (e.g., MPF) that can "instruct" a G₂-arrested oocyte to enter and progress through meiosis. PF activity is high in mitotic cytoplasm but low in interphase M cytoplasm 1. G₂ Arrest (Low MPF Activity): a. Initial state: Oocytes are arrested in the G₂ phase of the cell cycle. b. MPF activity islowduring this phase, preventing progression into meiosis. 2. Progesterone Stimulation: a. Progesterone treatment triggers the activation of MPF. b. T his rise in MPF activity drives the oocyte fromG₂ arrest into meiosis I. 3. Meiosis I (High MPF Activity): a. MPF activity peaks during meiosis I, enabling chromosome condensation, spindle formation, and other mitotic events. b. After homologous chromosomes segregate, MPF activity temporarily drops, signaling the transition to meiosis II. 4. Metaphase II Arrest (Sustained High MPF Activity): a. Following meiosis I, MPF activity rises again and remains high, maintaining the oocyte inmetaphase II arrest. b. This arrest ensures that the oocyte is prepared for fertilization. 5. Fertilization: a. Fertilization triggers arise in intracellular Ca²⁺, leading to: i. Cyclin B degradation(a key component of MPF). ii. A sharp decline in MPF activity, releasing the oocyte from metaphase II arrest. b. This allows the completion of meiosis II, forming a zygote. 6. First Embryonic Mitosis (Oscillatory MPF Activity): a. After fertilization, MPF activity rises again to drive the first mitotic division of the zygote. b. This is followed by periodic oscillations of MPF activity during subsequent synchronous embryonic divisions. 7. Second Embryonic Mitosis: a. MPF activity oscillates, with peaks corresponding to mitosis and troughs during interphase. b. These rapid, synchronized divisions are characteristic of early embryogenesis. ) How to identify 2 cyclins Biochemical Approach: 35S-Methionine Labeling: ○ Injected into cells to label newly synthesized proteins. SDS-PAGE Analysis: ○ Protein extracts collected at multiple timepoints. ○ Proteins separated by size to detect changes in abundance over time. Result: ○ Cyclins were identified as proteins with levels that increase and decrease cyclically during cell cycle transitions. ○ Bands corresponding to cyclins show periodic appearance and disappearance. ○ Specifically, cyclins B and C were identified. C yclin abundancepeaks before each cell division. Degradation of cyclin corresponds to the completion of mitosis. Peaks in cyclin levels precede spikes in cell division activity, indicating thatcyclin accumulation triggers mitosis. itosis Promoting Factors: heterodimers of mitoticcyclin and M Cyclin-Dependent Kinase (CDK) 2. CDK in yeasts and mammals ) CDKs in budding or 1 Yeast as a Model Organism for Cell Cycle Studies: fission yeasts Yeast (both budding and fission yeast) is widely used due to: ○ H aploid cells: Genetic studies are simpler since mutations are immediately expressed. ○ Ease of manipulation: Yeast can be genetically engineered using a variety of techniques. ○ Distinct division methods: Budding yeast divides by forming a bud, making cell cycle stages easy to observe visually. Fission yeast divides symmetrically, providing a simple, binary division model. Benefit: Allows tracking of cell cycle progression. Yeast genetics identified temperature-sensitive (ts) mutants of CDKs, which revealed their critical roles in the cell cycle. (a) Scanning Electron Micrograph of S. cerevisiae Observation of Cell Cycle Stages: ○ Cells show varying sizes of buds at different stages of the cycle. ○ Bud Size:Larger buds indicate progression further into the cell cycle. Bud Scars: ○ V isible marks on the cell surface where previous daughter cells budded off. (b) Cell Cycle Events in S. cerevisiae 1. Key Features: ○ G1 Phase: Daughter cells must grow to a specific size before passing through theSTART checkpoint(commitment to the cell cycle). ○ G2 Phase: Not well-defined in budding yeast (indicated by a dashed arrow in the figure). ○ Mitosis (Closed Mitosis): The nuclear envelope remains intact throughout mitosis. Unlike higher eukaryotes, chromosomes do not condense visibly under light microscopy. 2. Unique Characteristics: ○ Closed Mitosis: Nuclear envelope does not disassemble during mitosis. ○ Small Chromosomes: Chromosomes do not condense enough to be observed under light microscopy. (c) Scanning Electron Micrograph of S. pombe Observation of Cell Cycle Stages: ○ Long Cells:About to enter mitosis. ○ Short Cells:Have just completed cytokinesis. (d) Cell Cycle Events in S. pombe 1. Key Features: ○ Short G1 and S Phases: Compared to metazoans, these phases are relatively brief in fission yeast. ○ Prominent G2 Phase: This phase is well-defined and prominent, similar to that of metazoan cells. ○ Closed Mitosis: Like S. cerevisiae, the nuclear envelope does not disassemble during mitosis. 2. Comparison with S. cerevisiae: ○ Chromosome Condensation: Similar to S. cerevisiae, chromosomes are not highly condensed. ○ Cell Shape: Cylindrical shape of S. pombe facilitates isual distinction between cell cycle stages v based on length. Key Comparisons Between S. cerevisiae and S. pombe Feature S. cerevisiae S. pombe (Fission (Budding Yeast) Yeast) G2 Phase Not well-defined Prominent uclear N emains intact R emains intact R Envelope (closed mitosis) (closed mitosis) hromosome C inimal, not visible M inimal, not visible M Condensation under light under light microscopy microscopy ivision D Asymmetric budding ymmetric binary S Mechanism fission Cell Shape Round with buds Cylindrical Mutant Studies in Fission Yeast (CDC2 Mutants) 1. CDC2 Recessive Mutant: ○ Phenotype:Cells fail to progress through the cell cycle and continue growing without dividing, resulting in abnormally elongated cells. ○ Cause:Loss of function in CDC2 (a CDK) prevents entry into mitosis. 2. CDC2 Dominant Mutant: ○ Phenotype:Cells divide prematurely, producing smaller-than-normal daughter cells. ○ Cause:Hyperactive CDC2 accelerates mitosis, bypassing proper growth and preparation. 3. Wild-Type CDC2: ○ Phenotype:Normal cell division with properly sized daughter cells. 4. Significance: ○ These mutants demonstrate that CDC2 (a Cyclin-Dependent Kinase) is essential for regulating the timing of cell cycle transitions, particularly the entry into mitosis. 2) CDKs in mammals Primary Cell Culture and Cell Line Definition of Cell Lines: ○ Mammalian cells can be isolated and cultured in a controlled environment. ○ Primary cultures: Derived directly from tissues. ○ Cell lines: Immortalized cells that can proliferate indefinitely in vitro. Microscopic Observation: ○ Phase-contrast microscopy is used to examine the morphology and cell cycle progression of cultured cells. etermining Cell Cycle Stages with FACS (Fluorescence Activated D Cell Sorting) FACS Overview: A powerful technique for analyzing cell cycle stages by DNA content. DNA is stained with a fluorescent dye, and fluorescence intensity correlates with DNA quantity. Key DNA Content Indicators: 2N DNA Content: Cells in the G1 phase. 4N DNA Content: Cells in G2/M phase. Intermediate fluorescence represents cells in S phase (DNA synthesis). Peaks correspond to distinct cell cycle stages. FACS Analysis After Drug Treatments Effect of Drug Treatments: ○ Drugs that target specific cell cycle processes reveal CDK activity: ○ DNA Replication Inhibitor: Arrests cells in S phase. ○ MT (Microtubule) Inhibitor: Blocks mitosis. ○ CDK1 Inhibitor: Prevents entry into mitosis. ○ S erum Starvation: Arrests cells in G1 phase due to nutrient deprivation. FACS Graph Analysis: ○ DNA content peaks show how specific inhibitors block cell cycle transitions. Cyclin-CDK Pairing by Phase: G1 Cyclin-CDK: Cyclin D-Cdk4/6. G1/S Cyclin-CDK: Cyclin E-Cdk2. S Cyclin-CDK: Cyclin A-Cdk2. M Cyclin-CDK: Cyclin A/B-Cdk1. Functions of Cyclins: Activate CDKs: Cyclins bind to CDKs to activate their kinase activity. Recruit Specific Substrates: Direct CDKs to target proteins for phosphorylation. Importance of CDK Substrates: CDKs regulate the cell cycle by phosphorylating target proteins. Methodology Using ATP Analog: Mutant kinases that accept modified ATP analogs are used for specific substrate labeling. Steps in Substrate Identification: Kinase binds ATP analog and transfers a phosphate group to substrates. Phosphorylated substrates can then be isolated for analysis. Advanced Substrate Identification Techniques Workflow for Substrate Identification: 1. Mutant Kinase Design: Introduce a specific mutation in the kinase to accept ATP analogs. 2. Thio-Phosphorylation: Use ATP analog containing sulfur for selective labeling. 3. Sulfur Coupling: Isolate proteins with sulfur-phosphorylated sites. 4. Protein Sequence Analysis: Determine the amino acid sequences of phosphorylated substrates to identify CDK targets. Goal: Identify and characterize the substrates regulated by CDKs during the cell cycle. 3. Cell cycle regulation in eukaryotic cells ) Regulation of cyclin 1 Cyclin Expression: (activator) C yclins are expressed at specific stages of the cell cycle, binding to CDKs and activating their kinase activity. Cyclins ensure proper timing and progression through cell cycle phases. Cyclin types and their roles: ○ Cyclin D:Active in G1 phase. ○ Cyclin E:Peaks during the G1/S transition. C ○ yclin A:Active in the S phase. ○ Cyclin B:Peaks during mitosis. Cyclin Degradation: Cyclins are degraded byE3 ubiquitin ligases: ○ SCF Complex: Targets G1 cyclins and cyclins during the G1 → S transition. ○ APC/C Complex: Degrades mitotic cyclins (e.g., Cyclin B) during the metaphase-to-anaphase transition and M → G1. Ubiquitination Mechanism: E3 ligases tag cyclins with ubiquitin, marking them for degradation by the26S proteasome. S CF and APC/C play distinct roles: ○ SCF:Functions throughout the cell cycle, especially in G1/S transitions. ○ APC/C:Active during mitosis to promote anaphase and exit from mitosis. Structural Changes in CDKs: C yclin binding induces a conformational change in CDKs, partially activating them by exposing the T-loop for further phosphorylation. ) Regulation by CKIs 2 CKIs Overview: (inhibitor) C KIs are proteins that bind to cyclin-CDK complexes and inhibit their activity, providing a checkpoint mechanism to halt cell cycle progression under unfavorable conditions. Types of CKIs: p21-CIP and p27-KIP1: ○ Broadly inhibit multiple cyclin-CDK complexes. ○ Play roles in DNA damage responses. p16-INK4: ○ Specifically inhibits Cyclin D-Cdk4/6, preventing G1 progression. CKI Degradation: C KIs are degraded by theSCF complex, which removes their inhibitory effects and allows cell cycle progression. This regulated degradation ensures that CKIs halt the cell cycle only temporarily, when needed. ) Regulatory 3 phosphorylation of Activating Phosphorylation: CDKs ○ CDK-Activating Kinase (CAK): Phosphorylates the T-loop of CDKs, fully activating them. This phosphorylation stabilizes the cyclin-CDK interaction and enhances substrate binding. Inhibitory Phosphorylation: ○ Wee1 Kinase: Adds inhibitory phosphates to CDKs, rendering them inactive. This ensures the cell does not prematurely progress through the cell cycle. ○ Reversal by Cdc25 Phosphatase: Removes the inhibitory phosphates added by Wee1, reactivating CDKs. Essential for checkpoint release and progression into mitosis. Structural Insights into CDK Regulation: ○ Inactive CDK State: T-loop blocks the active site, preventing substrate binding. ○ Partially Active CDK State: Cyclin binding opens the active site by displacing the T-loop. ○ Fully Active CDK State: CAK phosphorylation of the T-loop stabilizes the open conformation and enhances activity. G-loop phosphorylation 1. Active and Inactive States of CDK: ○ Active CDK: Cyclin binds to CDK, partially activating it. CDK-activating kinase (CAK) adds an activating phosphateto theT-loop, further stabilizing and fully activating the CDK. ○ Inactive CDK: Wee1 Kinaseadds aninhibitory phosphateto the CDK, even if cyclin is bound. This inhibitory phosphorylation blocks CDK activity, preventing premature progression through the cell cycle. 2. Key Players in Regulation: ○ Wee1 Kinase: Adds inhibitory phosphates, ensuring CDKs are inactive during inappropriate stages or in response to checkpoints. ○ Cdc25 Phosphatase: Removes inhibitory phosphates, reactivating CDKs and allowing progression through the cell cycle. ○ Cyclin Binding: Essential for CDK activation but insufficient alone without appropriate phosphorylation/dephosphorylation. CDK1 phosphorylation Sites of Phosphorylation: Tyrosine (Y) and Threonine (T) Residues: ○ Represent the key phosphorylation sites on CDK1. Tyrosine (Y) and Threonine Inhibition by Wee1/Myt1: ○ Adds inhibitory phosphate groups, blocking CDK activity. Threonine Activation by CAK: ○ CAK phosphorylates threonine (T), ensuring full CDK activation. CDK1 Regulation Cycle: Wee1/Myt1: ○ Adds inhibitory phosphates at tyrosine and threonine sites. ○ Inactivates CDK1 to prevent premature entry into mitosis. Cdc25 Phosphatase: ○ Removes inhibitory phosphates added by Wee1/Myt1. ○ Reactivates CDK1 at the appropriate time for mitotic progression. CAK (CDK-Activating Kinase): ○ Adds an activating phosphate to CDK1’s threonine residue, fully activating the kinase. summary Cyclins: Activate CDKs, but their activity is temporally regulated by synthesis and degradation. CKIs: Provide an additional layer of regulation by halting CDK activity during stress or checkpoints. Phosphorylation: Fine-tunes CDK activity, balancing activation (by CAK) and inhibition (by Wee1 and Cdc25). 4. Cell cycle progression and checkpoint G1 phase Key Cellular Processes in G1: G rowth in Size:Cell increases in size to preparefor division. Synthesis of RNAs and Proteins:Prepares the molecular machinery needed for subsequent phases. Synthesis of Organelles:Organelles are duplicatedto ensure proper cell function after division. G1 Checkpoint (START): ○ Acts as a decision point to determine if the cell commits to division. ○ Cells must pass this checkpoint to proceed to the S phase. Molecular Players: ○ Growth Factors and Nutrients:External signals that regulate progression through G1. ○ Cyclin D-Cdk4/6 Complex: Activated by growth factors and drives progression through G1. ○ Retinoblastoma Protein (Rb): Inhibits E2F transcription factors in its unphosphorylated state. Phosphorylation by Cyclin D-Cdk4/6 inactivates Rb, releasing E2F. ○ E2F Transcription Factor: Activates genes required for S phase entry, including those encoding Cyclin E and Cyclin A. ○ Cyclin E/A-Cdk2 Complex: Promotes DNA synthesis and centrosome duplication. Role of Rb and E2F: ○ Unphosphorylated Rb: Binds and inhibits E2F transcription factors. ○ Phosphorylated Rb: Cyclin D-Cdk4/6 phosphorylates Rb, releasing E2F. E2F Activation: ○ E2F drives the transcription of genes encoding Cyclin E and Cyclin A. ○ Cyclin E/A-Cdk2 complexes promote the transition from G1 to S phase. Feedback Loops: ○ Cyclin E-Cdk2 further phosphorylates Rb, enhancing E2F activity and creating a positive feedback loop. CKI Removal and SCF in G1-S Transition CKI (Cyclin-Dependent Kinase Inhibitor) Inhibition: ○ CKIs inhibit the S phase CDK (Cyclin E/A-Cdk2) to prevent premature entry into S phase. SCF Ubiquitin Ligase: ○ Adds ubiquitin tags to phosphorylated CKIs, marking them for proteasomal degradation. Key Steps in CKI Removal: ○ G1/S CDK Phosphorylation:Cyclin E-Cdk2 phosphorylates CKIs, signaling them for degradation. ○ CKI Degradation:SCF ubiquitin ligase tags CKIs, allowing the cell to progress into the S phase. Molecular Players Recap: ○ Growth Factors and Nutrients:Activate Cyclin D-Cdk4/6. ○ Cyclin D-Cdk4/6:Phosphorylates Rb to activate E2F. ○ Cyclin E/A-Cdk2:Promotes DNA synthesis and CKI degradation for S phase progression 1. Initial Inhibition by Rb: ○ Unphosphorylated Rb (Retinoblastoma protein): Rb binds to and inhibitsE2F transcription factors, preventing transcription of S-phase-promoting genes. 2. Activation by Growth Factors: ○ Externalgrowth factorsactivate theCyclin D-Cdk4/6 complex. ○ Cyclin D-Cdk4/6phosphorylates Rb. 3. Phosphorylation of Rb: ○ Phosphorylation inactivates Rb, causing it to release E2F. ○ This step is essential to overcome the G1 checkpoint. 4. E2F Activation: ○ FreedE2Fbinds to DNA and activates the transcription of genes required for S phase entry, including: Cyclin EandCyclin Agenes. 5. Production of Cyclin E and Cyclin A: ○ Transcription of Cyclin E and Cyclin A mRNA leads to their synthesis. ○ These cyclins bind to CDK2 to form activeCyclin E/A-Cdk2 complexes. 6. Role of Cyclin E/A-Cdk2: ○ Drives progression through the G1/S transition (START) and prepares the cell for S phase: S Phase Initiation:Promotes DNA replication. Centrosome Duplication:Ensures proper spindle formation during mitosis. 7. Positive Feedback Loop: ○ Cyclin E-Cdk2further phosphorylates Rb, enhancing E2F activity. ○ This creates a positive feedback loop, ensuring robust commitment to the S phase. 1. CKI Inhibition in Mid-Late G1 Phase CKI (Cyclin-Dependent Kinase Inhibitor): ○ Binds to S-phase Cyclin-CDK complexes (Cyclin A-Cdk2 or Cyclin E-Cdk2). ○ Prevents premature activation of S-phase CDKs, halting the transition to the S phase. 2. Phosphorylation of CKI by G1/S Cyclin-CDK Complex Role of G1/S Cyclin-CDK: ○ Phosphorylates CKI, marking it for degradation. ○ Phosphorylated CKIs are now recognized as substrates by the SCF ubiquitin ligase. 3. SCF-Mediated Polyubiquitination SCF Ubiquitin Ligase: ○ Attaches multiple ubiquitin molecules to phosphorylated CKIs. ○ This polyubiquitination marks CKIs for destruction by the proteasome. 4. Proteasomal Degradation CKI Removal: ○ Ubiquitinated CKIs are degraded by the26S proteasome. ○ This degradation relieves inhibition on the S-phase Cyclin-CDK complex. 5. Activation of S-Phase Cyclin-CDK Complex Result of CKI Removal: ○ S-phase Cyclin-CDK complex becomes fully active. ○ Promotes the initiation of S phase by driving DNA replication and other S-phase processes. 2) S phase Cellular Processes in S Phase Growth in Size: ○ The cell continues to increase in size to provide sufficient resources for division. DNA Synthesis: ○ Entire genome is replicated to ensure accurate genetic information is passed to daughter cells. Centrosome Duplication: ○ Centrosomes are replicated to prepare for spindle formation in mitosis. Sister Chromatids Cohesion: ○ Cohesin complexes ensure replicated sister chromatids are held together until separation during mitosis. Regulation of DNA Replication in S Phase Replication Origin Firing: ○ Replication origins are activated only once per cell cycle to ensure accurate replication. Replication Initiation Complex: ○ Loaded onto DNA at replication originsafter mitosis, when CDK activity is low. ○ Activated exclusively byS-phase CDKsto initiate replication fork progression. Prevention of Re-Replication: ○ The activation of replication origins by S-phase CDKs prevents origins from being reused in the same cell cycle. Molecular Players in S Phase Key Cyclin-CDK Complexes: Cyclin A-Cdk2: ○ Activates the replication machinery, including the replication initiation complex. ○ Drives progression through the S phase. S Phase CDKs: ○ Phosphorylate and activate essential proteins like MCM helicases for DNA replication. Replication Initiation Complex: Composed of multiple factors, including: ○ MCM Helicase:Essential for unwinding DNA at replication forks. ○ DDK (Dbf4-Dependent Kinase):Works alongside Cyclin A-Cdk2 to activate replication origins. Cohesion of Sister Chromatids Cohesin Complex: ○ Holds replicated sister chromatids together after DNA replication. ○ Ensures proper alignment and prevents premature separation. Role of CDKs in Cohesion: ○ S-phase CDKs ensure cohesin complexes are properly established during DNA replication. ○ Cohesion persists until mitosis, ensuring accurate segregation of sister chromatids. Key Steps in S Phase Regulation 1. Loading of the Replication Initiation Complex: ○ Occurs after mitosis when CDK activity is low. 2. Activation of the Replication Machinery: ○ S-phase CDKs phosphorylate and activate replication proteins like MCM helicase. 3. Progression of Replication Forks: ○ Cyclin A-Cdk2 drives replication fork activity. 4. Establishment of Cohesion: ○ Cohesin complexes are loaded onto replicated DNA strands to maintain alignment. 1. Pre-Replication Complex (Pre-RC) Loading: MCM Helicase: ○ TheMCM (Mini-Chromosome Maintenance) helicase is loaded onto replication origins during G1 when CDK activity is low. ○ The helicase remains inactive until the S phase. 2. Activation of the MCM Helicase: S Phase CDK and DDK Activation: ○ S-Phase CDKs:Phosphorylate key proteins like Cdc45andSld3/Sld2to activate replication. ○ DDK (Dbf4-Dependent Kinase): Specifically phosphorylates and activates the MCM helicase. 3. Formation of the Replication Fork: Recruitment of Replication Factors: ○ Activation of the MCM helicase leads to the recruitment of the following factors: Cdc45/Sld3:Assists in helicase activation and assembly of the replication machinery. Dpb11/Sld2:Helps in stabilizing the replication fork and recruiting other proteins. GINS Complex:Acts as a processivity factor, stabilizing the MCM helicase for efficient DNA unwinding. Helicase Activity: ○ The active MCM helicase unwinds DNA strands, creating a replication fork and allowing access for DNA polymerase to start replication. MCM Helicase: Key complex for DNA unwinding at replication forks. S-Phase CDKs: P hosphorylate replication initiation factors to activate the helicase. DDK (Dbf4-Dependent Kinase): Activates MCM helicase through phosphorylation. Replication Factors: C dc45, Sld3, Sld2, Dpb11, GINS Complex:Assist in replication fork assembly and stability. 1. G1 Phase: Cohesin Loading: ○ Cohesin complexes encircle individual DNA strands during G1. ○ Cohesins:Protein complexes that hold sister chromatids together after replication, ensuring their proper alignment and separation during mitosis. 2. S Phase: Replication Fork Formation: ○ DNA replication begins at replication origins, creating replication forks. ○ TheMCM helicaseand associated replication machinery unwind DNA and synthesize new strands. ○ Two sister chromatids are formed for each replicated DNA strand. Establishment of Cohesion: ○ CoAT Complex (Cohesion Activation Transition): Activates cohesin complexes to establish sister chromatid cohesion. Cohesin complexes now link newly synthesized sister chromatids together, preventing premature separation. 3. Post-Replication Cohesion: Sister Chromatids: ○ After DNA replication, cohesins hold the replicated sister chromatids tightly together. ○ This cohesion persists until the anaphase stage of mitosis, ensuring proper chromatid alignment on the spindle apparatus. Key Molecular Players: Cohesin Complexes: ○ Encircle DNA strands and establish cohesion between sister chromatids after replication. Replication Fork: ○ Machinery that drives DNA unwinding and synthesis during S phase. CoAT Complex: ○ Activates cohesins during S phase to hold sister chromatids together. G2 phase 1.Cellular Processes in G2 Phase Growth in Size: ○ The cell enlarges to ensure sufficient resources for mitosis. RNA and Protein Synthesis: ○ Prepares molecular machinery required for mitotic events. Preparation for Mitosis: ○ Ensures proper spindle formation and chromosome condensation. Checkpoint Monitoring: ○ DNA is assessed for replication errors or damage. ○ The cell decides whether to: Commit to Mitosis: If no issues are detected. Arrest in G2: If DNA damage is detected for repair. 2. DNA Damage Checkpoint and G2 Arrest Monitoring Genomic Integrity: ○ Ensures no DNA replication errors or damage are carried into mitosis. Genotoxic Stress Pathways: ○ DNA damage triggers two main pathways: p53 Pathway: Activates transcription of genes like ATF3, which suppress cell cycle progression. ATR/ATM Pathway: Activates Chk1/Chk2, which phosphorylate Cdc25A phosphatase to prevent mitotic entry. Cdc25 Phosphatase Inhibition: ○ Cdc25A degradation stops Cyclin-Cdk activity, arresting the cell in G2 phase. Outcomes of G2 Arrest: ○ Repair Damage: Cell resumes the cycle if DNA is repaired successfully. ○ Programmed Cell Death: Cell undergoes apoptosis if damage is irreparable. 3. Regulation of CDK Activity in G2 CDK1 Inhibition by Wee1 Kinase: ○ Adds inhibitory phosphates to CDK1, maintaining its inactive state. CDK1 Activation by Cdc25 Phosphatase: ○ Removes inhibitory phosphates from CDK1, reactivating it for the G2-to-M transition. Balance of Regulation: ○ Precise balance between Wee1 kinase and Cdc25 phosphatase ensures proper timing of mitosis entry. 4. Feedback Mechanisms in CDK Activation Positive Feedback Loops: ○ Once CDK1 is activated, it reinforces its activation through: Cdc25 Activation: CDK1 activates Cdc25 phosphatase, further removing inhibitory phosphates. Wee1 Inhibition: CDK1 inhibits Wee1 kinase, preventing the addition of inhibitory phosphates. Outcome of Feedback Loops: ○ Creates a robust, irreversible commitment to mitosis. 5. Molecular Players in G2 Phase Cdc25 Phosphatase: ○ Removes inhibitory phosphates from CDKs, enabling mitotic progression. ○ Its degradation during DNA damage halts progression to mitosis. Wee1 Kinase: ○ Adds inhibitory phosphates to CDKs to maintain G2 arrest when necessary. Cyclin A/B-Cdk1 Complex: ○ Key driver of the G2-to-M transition once activated. Chk1/Chk2: ○ Activated by the ATR/ATM pathway during DNA damage. ○ Phosphorylates and inhibits Cdc25 to enforce G2 arrest. 6. Outcomes of G2 Regulation Proper Mitotic Entry: ○ Activation of Cyclin A/B-Cdk1 ensures the cell is ready for mitotic division. Prevention of Error Propagation: ○ The G2 checkpoint prevents the division of cells with damaged or incomplete DNA. Decisions in G2: ○ Progression: If DNA is intact, the cell progresses to mitosis. ○ Repair or Death: If DNA damage is detected, the cell either repairs it or undergoes apoptosis. Genotoxic Stress Detection: G enotoxic Stress:Includes DNA damage caused by replication errors, UV radiation, or other mutagenic factors. Two main pathways respond to DNA damage: 1. p53 Pathway 2. ATR/ATM Pathway p53 Pathway: Activation of p53: ○ DNA damage activates the tumor suppressor proteinp53. p53-Induced Transcription: ○ p53 promotes the expression ofATF3(a transcription factor). Inhibition of Cdc25A Transcription: ○ ATF3 inhibits the transcription of theCdc25A gene, reducing its mRNA levels. Outcome: ○ D ecreased production of Cdc25A prevents cell cycle progression. ATR/ATM Pathway: Activation of ATR/ATM: ○ ATR (Ataxia Telangiectasia and Rad3-related) andATM (Ataxia Telangiectasia Mutated)are kinases activated in response to DNA damage. Checkpoint Kinases (Chk1/Chk2): ○ ATR/ATM phosphorylates and activates checkpoint kinasesChk1andChk2. Targeting Cdc25A: ○ Chk1/Chk2 phosphorylates Cdc25A, marking it for degradation. Cdc25A Degradation: Role of Cdc25A: ○ Cdc25A is a phosphatase required for activating Cyclin-Cdk complexes, driving cell cycle progression. Degradation: ○ Phosphorylated Cdc25A is targeted for proteasomal degradation. Outcome: ○ Depletion of Cdc25A halts Cyclin-Cdk activity, leading tocell cycle arrestin G2 phase. Cell Cycle Progression or Arrest: Without Cdc25A: ○ Cyclin A/B-Cdk1 complexes remain inactive. ○ The cell is arrested in G2, preventing entry into mitosis. Repair or Death: ○ During arrest, the cell attempts to repair DNA damage. ○ If repair is unsuccessful, the cell undergoes apoptosis. Summary of Molecular Players: p53: ○ Activates ATF3 to inhibit Cdc25A transcription. ATR/ATM: ○ Kinases that activate checkpoint proteins in response to DNA damage. Chk1/Chk2: ○ Phosphorylate Cdc25A, marking it for degradation. Cdc25A: ○ A phosphatase essential for Cyclin-Cdk activation and cell cycle progression. Outcome: ○ Prevents damaged cells from entering mitosis, ensuring genomic integrity. Summary of the Process: Genotoxic Stress Response: 1. Activation of Two Pathways: ○ p53 Pathway: Blocks Cdc25 transcription. i. Activation of p53due to stress. ○ ATR/ATM Pathway: Marks Cdc25 for degradation. i. Kinases that trigger checkpoint kinases (Chk1, Chk2). ii. These block Cdc25A advancement to help in problem cell pause-processing. 2. Cdc25A Inactivation: ○ Phosphatase activity halts to prevent damaged cells from progressing through the cycle. 3. Checkpoint Outcome: ○ Cell cyclearrests in G2. ○ DNA is repaired or apoptosis is initiated. 1. Inactive CDK1: ○ CDK1 is initially inactive due to inhibitory phosphorylation atY15(tyrosine) andT14 (threonine) byWee1 kinase. 2. Activation by Cdc25 Phosphatase: ○ Cdc25 phosphataseremoves the inhibitory phosphates from CDK1, converting it into its active form. ○ Activation is required for the G2 to mitosis transition. 3. Two Positive Feedback Loops: ○ Activation of Cdc25 Phosphatase: Active CDK1 enhances the activity of Cdc25 phosphatase, promoting further dephosphorylation of CDK1. ○ Inhibition of Wee1 Kinase: Active CDK1 inhibits Wee1 kinase, preventing additional inhibitory phosphorylation on CDK1. Outcome: These positive feedback loops ensure: ○ Rapid and robust CDK1 activation. ○ Irreversible commitment to mitosis. M phase 1. Cellular Processes in M Phase Key Events: ○ Nuclear envelope breakdown. ○ Chromosome condensation for segregation. ○ Spindle assembly ensures alignment. ○ Sister chromatid separation occurs at anaphase. ○ Mitotic exit and cytokinesis divide the cell into two daughter cells. 2. Molecular Players Spindle Assembly Checkpoint (SAC): ○ Monitors KT-MT attachments. ○ Ensures proper chromosome alignment. ○ Delays progression to anaphase if errors are detected. APC/C (Anaphase Promoting Complex/Cyclosome): ○ E3 ubiquitin ligase facilitating: Cohesin degradation for chromatid separation. Cyclin destruction to drive mitotic exit. 3. KT-MT Attachment Errors Types of Errors: ○ Amphitelic (Correct):Equal tension with proper attachment to opposite poles. ○ Monotelic:One kinetochore unattached. ○ Syntelic:Both chromatids attach to the same spindle pole. ○ M erotelic:One kinetochore attached to multiple spindles. Correction Mechanism: ○ Low tension activates Aurora B Kinase. ○ Aurora B disconnects incorrect MT attachments. How Errors Are Identified: L ow Tension:Aurora B kinase is active, indicating incorrect attachment. High Tension:Aurora B kinase is inactive, confirming proper attachment. ister chromatid separation S Cohesin Complex: H olds sister chromatids together to ensure proper alignment during metaphase. Prevents premature chromatid separation. APC (Anaphase-Promoting Complex): A ctivated by Cdc20 during metaphase-to-anaphase transition. Acts as an E3 ubiquitin ligase to tag target proteins for degradation. Securin Degradation: A PC ubiquitinates securin, marking it for degradation. Securin inhibits separase under normal conditions. Separase Activation: D egradation of securin releases active separase. Separase cleaves cohesin, breaking the linkage between sister chromatids. Chromatid Separation: O nce cohesins are cleaved, sister chromatids are pulled to opposite poles by the mitotic spindle, leading to proper chromosome segregation. Mitotic exit and cytokinesis Cyclin A/B-Cdk1 Complex (Active): C yclin A/B binds to Cdk1, forming an active complex critical for mitotic progression. Role of APC/C (Anaphase-Promoting Complex/Cyclosome): A PC/C ubiquitinates Cyclin A/B during the transition from metaphase to anaphase. Ubiquitination marks Cyclin A/B for proteasomal degradation. Cdk1 Inactivation: D egradation of Cyclin A/B leads to the inactivation of Cdk1. Cdk1 inactivation is necessary for mitotic exit and the initiation of cytokinesis. Mitotic Exit and Cytokinesis: M itotic exit involves chromosome decondensation, nuclear envelope reformation, and the completion of cell division (cytokinesis). Proper inactivation of Cdk1 ensures the cell successfully transitions from mitosis to the G1 phase. Fusion of Cells Overview of the Experiment: H uman cells at different cell cycle stages (G1, S, and G2) were fused to form single cells with two nuclei to study the regulation of S phase. The diagram illustrates three experimental scenarios (A, B, C), showing how the presence of active cyclin–Cdk complexes affects cell cycle progression and DNA replication. Scenario (A): Fusion of G1 and S Phase Cells Observation: ○ The G1 nucleus begins DNA replication, driven by the active S-phase cyclin–Cdk complexes present in the S-phase nucleus. Interpretation: ○ Cyclin–Cdk complexes in S phase can activate the replication machinery in the G1 nucleus, initiating DNA replication prematurely. Implication: ○ The S-phase nucleus produces factors (likely S-phase cyclin–Cdk complexes) that can override the G1 block and push the G1 nucleus into S phase. Scenario (B): Fusion of S and G2 Phase Cells Observation: ○ The G2 nucleus does not reinitiate DNA replication, even in the presence of the S-phase nucleus. Interpretation: ○ Once the G2 nucleus completes S phase, a block prevents re-replication. This block is likely maintained by specific mechanisms that inhibit reactivation of origins of replication. Implication: ○ A "re-replication block" ensures that DNA replication occurs only once per cell cycle, maintaining genomic integrity. Scenario (C): Fusion of G1 and G2 Phase Cells Observation: ○ Neither nucleus undergoes DNA replication. The G1 nucleus remains in G1, and the G2 nucleus does not initiate replication. Interpretation: ○ The G2 nucleus cannot influence the G1 nucleus to enter S phase because it lacks active S-phase cyclin–Cdk complexes. ○ The G1 nucleus does not produce signals to initiate replication in the G2 nucleus. Implication: ○ G2 nuclei are resistant to factors that could drive re-replication, and G1 nuclei require specific -phase cyclin–Cdk signals to progress into S replication. Key Conclusions: 1. Role of Cyclin–Cdk Complexes: ○ S-phase cyclin–Cdk complexes are crucial for initiating DNA replication in G1 nuclei. 2. Re-replication Block: ○ G2 nuclei have a safeguard mechanism preventing re-replication of DNA, ensuring a single round of replication per cell cycle. 3. Cell Cycle Stage-Specific Regulation: ○ The outcomes demonstrate strict regulation of the cell cycle, with transitions driven by distinct cyclin–Cdk activity and inhibitory mechanisms. summary . 1 1 checkpoint (START point) G Goal: inhibit G1/S cyclin-Cdk2 activity Target: E2F activity 2. G2/M checkpoint Goal: inhibit M cyclin-Cdk1 activity Target: Cdc25 activity 3. M checkpoint (Spindle assembly checkpoint) Goal: keep M cyclin-Cdk1 activity Target: APC/C activity