Body Fluid Compartments (PDF)
Document Details
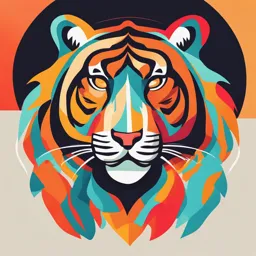
Uploaded by AdventuresomeSatyr
UCL
Dr Richard Tunwell
Tags
Summary
This document appears to be lecture slides from UCL on Body Fluid Compartments. It covers topics such as chemical composition, the properties of water, osmotic pressure, diffusion, and methods for measuring fluid volumes. The slides also discuss related concepts like osmolality and tonicity and provides examples relevant to physiology.
Full Transcript
PHOL0001 PHOL0001 Body Fluid Compartments Dr Richard Tunwell [email protected] Body Fluid Compartments This lecture will discuss: The chemical composition of the body The properties of water as a biological solvent The osmotic pressure and to...
PHOL0001 PHOL0001 Body Fluid Compartments Dr Richard Tunwell [email protected] Body Fluid Compartments This lecture will discuss: The chemical composition of the body The properties of water as a biological solvent The osmotic pressure and tonicity of aqueous solutions How body water is distributed between the various fluid compartments How the volumes of the compartments can be measured. Reading Human Physiology: Pocock, Richards and Richards 3rd Edition: Chapter 2, pages 5-16 4th Edition: Chapter 2, pages 14-25 Chapter 3, pages 27-43 5th Edition: Chapter 2, pages 14-25 Chapter 3, pages 27-43 Body Composition The human body largely consists of the four elements oxygen, carbon, hydrogen and nitrogen: Percentages are expressed as per cent body weight Body Mass Water accounts for about two thirds of lean body mass: The body of a normal 70 kg adult holds about 42 litres of water. Water the “universal” solvent The electronegativity of oxygen and the fact that the atoms in water are not linear result in the molecule being polar. The charge difference allows the molecule to ‘hydrogen bond’ with other water molecules and dipoles in other molecules. This explains many of the properties of water, such as its solvent properties. Taken from https://en.wikipedia.org/wiki/Properties_of_water Classification Chemical species can be divided into three classes: Hydrophilic substances that readily dissolve in water. These molecules (including ions) are polar in nature Examples include glucose, Na+, ethanol and many proteins. Hydrophobic substances that are insoluble in water. These molecules are non-polar such as fats and waxes and cholesterol. Amphiphilic substances that have mixed properties - one part of the molecule is polar and the other is non-polar. Examples are the long chain fatty acids, bile salts and the phospholipids. Structure Hydrophilic (polar) Hydrophobic (non-polar) Amphiphilic Diffusion When in solution, substances (solute) disperse in a random process called diffusion. The rate of diffusion depends on: The concentration gradient The area available for diffusion The molecular mass of the solute The diffusion coefficient – A physical constant that reflects the molecular characteristics of both solute and solvent. This constant also depends on temperature. Diffusion Fick’s Law of Diffusion: Amount moved = Coefficient x Area x Concentration gradient Or in symbols: dC J =- D A dx D is the diffusion coefficient, A is the area over which diffusion can occur and dC/dx is the concentration gradient. The negative sign indicates that diffusion occurs from a region of high concentration to one of low concentration. The diffusion coefficient becomes smaller as the molecular size increases so that large molecules diffuse more slowly than small ones. Osmotic Pressure Osmosis is the movement of water (or other solvent) through a semipermeable membrane that permits the passage of the water but not the solute particles. A hydrostatic pressure sufficient to stop this flow of water is called the osmotic pressure. Osmotic pressure plays an important role in the transport of molecules across membranes. Osmotic Pressure Substances in solution exert an osmotic pressure Pressure= force/Area solution pure water semi-permeable membrane Initial volume Water drawn Pressure applied to of solution across semi-permeable solution prevents membrane by osmosis water uptake The osmotic pressure of a solution is the pressure that is just sufficient to prevent the movement of water into a solution across a semi-permeable membrane. Osmotic Pressure Osmotic pressure (π) = MRT Where M is the Molality, R is the universal gas constant (8.31JK- 1mol-1) and T is the absolute temperature (310K at normal body temperature) Osmotic pressure depends on the number of solute particles present per unit volume of solvent and NOT on their chemical makeup. Salts separate into their constituent ions, so the osmotic pressure that a salt such as sodium chloride will exert will be twice its molar concentration. Osmole The unit of osmotic concentration is the osmole. This is a non SI unit of measurement that defines the number of moles of solute that contribute to the osmotic pressure of a solution. The total osmotic concentration of a solution is the sum of the osmotic concentrations due to each of the solutes in the solution. Reverse Osmosis Osmotic pressure is the basis of process to purify water called reverse osmosis. The water to be purified is placed in a chamber and put under an amount of pressure greater than the osmotic pressure exerted by the water and the solutes dissolved in it. Part of the chamber opens to a semi permeable membrane that lets water molecules through, but not the solute particles. Reverse Osmosis The osmotic pressure of sea water is about 27 atm so reverse osmosis desalinators use pressures around 50 atm (5,066 kPa) to produce fresh water from sea water. One atm equals 101.325 kPa Osmotic Pressure Rather than measure osmotic pressure directly, it is more convenient to state: osmolarity (moles solute particles per litre of solution) or osmolality (moles solute particles per kg of water). In clinical medicine, osmotic pressures of body fluids are generally expressed as osmolality The difference between osmolality and osmolarity when considering body fluids is negligible because these fluids are typically dilute aqueous solutions. By Definition: One mole of a non-dissociating substance in 1kg of water has an osmolality of 1 Osmol kg-1 Similarly a 1M solution of glucose has a concentration of 1 Osm and osmolarity of 1 Osmol L-1 Osmolality The total osmolality of a solution is the sum of the osmolality due each of the constituents of the solution The blood plasma has an osmolality of around 300 mOsmol kg-1 The principal ions (Na+, K+, Cl-, HCO3-) contribute most of this (around 290 mOsmol kg-1). Glucose and other small molecules contribute just under 10 mOsmol kg-1. Proteins contribute only about 1 mOsmol kg-1 (less than 0.5% of total plasma osmolality). Osmolality of Blood Plasma Why do proteins contribute such a small amount towards the total osmotic concentration (osmolality) of blood plasma and therefore to the total osmotic pressure? Given each kg of plasma contains ~ 6.76 g of NaCl and 47.4g of albumin and the molecular weight of NaCl is 58.4 and albumin is 69,000 Calculate the osmolality due to NaCl: Calculate the osmolality due to albumin: Osmotic Pressure Why do proteins contribute such a small amount towards the total osmolality of blood plasma and therefore to the total osmotic pressure? Each kg of plasma contains ~ 6.76 g of NaCl and 47.4g of albumin and the molecular weight of NaCl is 58.4 and albumin is 69,000 The osmolality due to NaCl: 2(6.76/58.4) = 0.231 Osmol kg-1 or 231 mOsmol kg-1 The osmolality due to albumin: 47.4/69,000 = 0.687 mOsmol kg-1 The blood plasma and other body fluids typically have an osmolality of around 300 mOsmol kg-1 Iso-osmotic vs Isotonic The tonicity of a solution refers to the influence of its osmotic concentration on the volume of cells. Two solutions with the same osmotic concentration are iso-osmotic. The volume of cells normally remains constant so the intracellular fluid must have the same osmolality as the extracellular fluid. The two solutions are said to be isotonic with each other. Fluids which are isotonic are also iso-osmotic. However, not all iso-osmotic solutions are isotonic with cells. – iso-osmotic solutions of urea cause cells to swell… why? Iso-osmotic vs Isotonic A cell placed in 150 mM NaCl 300 mOsmol kg-1 300 mOsmol kg-1 Na Na+ Cl-Na The two solutions are iso-osmotic because their osmotic concentrations are the same and also isotonic because the ions cannot move into the cell. Iso-osmotic vs Isotonic A cell placed in 300 mM Sucrose 300 mOsmol kg-1 300 mOsmol kg-1 Sucrose Na a The two solutions are iso-osmotic because their osmotic concentrations are the same and also isotonic because the ions cannot move into the cell. Iso-osmotic vs Isotonic A cell placed in 300 mM Urea 300 mOsmol kg-1 300 mOsmol kg-1 Urea The two solutions are initially iso-osmotic, but very quickly the urea diffuses down its concentration gradient into the cell. The osmotic concentrations change, becoming lower outside and higher inside the cell. Iso-osmotic vs Isotonic A cell placed in 300 mM Urea 300 mOsmol kg-1 300 mOsmol kg-1 Urea H2 O Water moves by osmosis from low solute (outside) to high solute (inside). If the cell is flexible, such as an erythrocyte, it grows in size to accommodate the extra volume. The urea solution is not isotonic A cell placed in 300 mM Urea the volume or pressure get bigger and bigger until such time as the urea and water stop moving or it bursts! Osmotic Phenomenons Isotonic Hypotonic Hypertonic Blood plasma is isotonic with red blood cells (erythrocytes) Red blood cells burst if the blood is diluted sufficiently with water since their flexible membranes cannot withstand the osmotic pressure within the cells. Blood therefore diluted with an isotonic (0.9%) NaCl solution. H Osmotic Phenomenons Cells living in aqueous solutions are usually hypertonic and subject to a continuous influx of water. A pressure builds up inside that would rupture the cell. Some ciliates (e.g. Paramecium) and flagellates (e.g. Euglena) solve this problem by having a special organ, a pulsating vacuole, that actively pumps water out. 1995-2009 by Michael W. Davidson and The Florida State University. www.bio.mtu.edu/the_wall/phycodisc/EUGLENOPHYTA/ Body Water Transcellular water(0.8 liters) Interstitial water (10.4 liters) 24% 67% 7% Plasma (2.8 liters) Intracellular water (28 liters) Body Fluid Volumes Measurement of body fluid volumes Volumes of irregularly shaped objects can be measured by dilution methods. concentration = mass/volume volume = mass/concentration Total body water can be measured using 3H2O or 2H2O Plasma volume can be measured using the dye Evans Blue (high affinity for serum albumin). Extracellular volume can be measured using a plant polysaccharide called inulin (NOT insulin). Example Measuring plasma volume 10 ml of a 1% solution (0.1 g or 100 mg) of Evans Blue was injected into a subject’s vein. After 5 min a sample of blood was taken and the plasma was found to have 0.037 mg of dye per ml of blood. As volume = mass/concentration. Plasma volume = 100/0.037 = 2702 ml. Total Body Water Total body water = extracellular water + intracellular water Extracellular water = plasma + interstitial water If we can measure total body water, the plasma volume and the volume of the extracellular water, we can calculate the volumes of the interstitial and intracellular compartments. If total body water is 43 litres, the plasma volume is 3 litres and the extracellular water volume is 16 litres… Intracellular water volume = 43 - 16 = 27 L Interstitial water volume = 16 - 3 = 13 L Summary Water accounts for 50-60% of total body mass. It is the chief solvent of the body. It is divided between two main compartments: the intracellular fluid and the extracellular fluid. The extracellular fluid is made up of the plasma and the interstitial fluid. The volumes of these compartments can be measured using dilution methods. When substances dissolve in water, they exert an osmotic pressure that is proportional to their molar concentration. The intracellular and extracellular fluids are isotonic with each other. Cells swell (or burst) when placed in distilled water and shrink when placed in concentrated salt solutions.