Biomolecules and Cytology PDF
Document Details
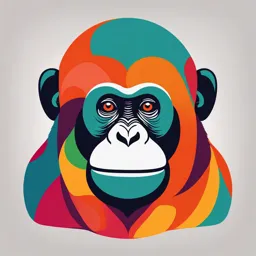
Uploaded by FantasticSimile7063
Xaverian College
Tags
Related
- Biomolecules: Carbohydrates, Lipids, Proteins, and Nucleic Acids PDF
- Biomolecules: Lipids, Carbohydrates, Proteins, and Nucleic Acids PDF
- Biomolecules 2024 - Carbohydrates (1) PDF
- Biomolecules (PL1003) Notes on Carbohydrates PDF
- Lesson 2 Carbohydrates Biomolecules PDF
- Biomolecules Carbohydrates CLawson 2024 PDF
Summary
These notes cover Biomolecules and Cytology for a Pre-Medical/Pre-Dental Foundation Year, Semester One. They discuss carbohydrates, including monosaccharides, disaccharides, and polysaccharides, as well as their structures and functions.
Full Transcript
Pre-Medical / Pre-Dental FOUNDATION YEAR Semester One 1. Bio-Molecules & Cytology Carbohydrates – mono and disaccharides Carbohydrates contain the elements, carbon, hydrogen and oxygen. These elements are present in the ratio: Carbohydrates can be can be classified into three groups...
Pre-Medical / Pre-Dental FOUNDATION YEAR Semester One 1. Bio-Molecules & Cytology Carbohydrates – mono and disaccharides Carbohydrates contain the elements, carbon, hydrogen and oxygen. These elements are present in the ratio: Carbohydrates can be can be classified into three groups: monosaccharides, disaccharides and polysaccharides. Monosaccharides and disaccharides are small, soluble molecules that are easy to transport and sweet to taste. Monosaccharides (single sugars) Monosaccharides are the basic molecular units (monomers) of carbohydrates. They are mainly used in respiration to provide energy and in growth during the formation of larger carbohydrates. They include: glucose, fructose and galactose which all have the same formula C6H12O6 (hexoses) but different structure (isomers). All are reducing sugars so give a +ve Benedict’s test result (brick red colour). Structure of Glucose Disaccharides (double sugars) A disaccharide is formed when two monosaccharides are joined together by a glycosidic bond in a condensation reaction. A condensation reaction is a reaction in which smaller molecules join together to form a larger molecule with the release of water. Monosaccharide + Monosaccharide Disaccharide + Water Glucose + Glucose + Water Glucose + Sucrose + Water Glucose + Galactose + Water C6H12O6 + C6H12O6 + Water 2 A disaccharide can be broken down into its monosaccharides during a hydrolysis reaction. Hydrolysis is the breakdown (lysis) of a larger molecule into smaller molecules with the addition of water (hydro). Disaccharides can be hydrolysed by boiling with acid e.g. dilute HCl or by heating with an enzyme at an optimum temperature. Example: Hydrolysis of sucrose Enzyme sucrase Sucrose + Water Glucose + Fructose C12H22O11 + H20 C6H12O6 + C6H12O6 Disaccharide Monosaccharides Example: Formation & Hydrolysis of Maltose 3 Carbohydrates – polysaccharides Polysaccharides are large polymers of monosaccharide molecules. The polysaccharides, cellulose, starch and glycogen are polymers of glucose and are formed by the joining of glucose molecules during condensation reactions. Polysaccharides differ in the number and arrangement of the glucose molecules they contain. They function as storage or structural molecules. They are non-reducing – giving a negative result in the Benedict’s test. They are unsweet to taste and relatively insoluble in water. Starch This is the storage carbohydrate found in plants. Starch is stored in starch grains (amyloplasts) in the cytoplasm. Starch has approximately 1,000 α glucose molecules joined together by condensation reactions. There are α1-4 glycosidic bonds, this mean the glucose molecules form into a chain called amylose. There are also α1-6 bonds that form branch points along the chain forming amylopectin. Hydroxyl (OH) groups form hydrogen bonds within the same molecule producing compact helical chains. Structure of Starch 4 Starch is ideally suited to its function as a storage compound as It is insoluble and therefore.............................................................................................. The molecule has a helical shape..................................................................................... Starch is hydrolysed by the enzyme amylase to produce the disaccharide maltose. Amylase Starch(Polysaccharide) + Water Maltose (disaccharide) Glycogen This is the storage carbohydrate found in the cytoplasm of animal cells and fungi. It is sometimes referred to as ‘animal starch’ due to similarities in structure and function between the two molecules. In humans it is found in liver and skeletal muscle cells. Glycogen is: insoluble and osmotically inactive - ideal storage properties; A polymer of glucose, similar to starch but with more branches (free ends). How is this structure an adaptation of the polysaccharide that makes it better suited to its function in animals? 5 Lipids Lipids are a broad group which includes fats, waxes, sterols, fat-soluble vitamins and phospholipids. The main biological functions of lipids include …………………………………………................. ………………………………………………………………………………………………………………. Lipids contain the elements, carbon, hydrogen and oxygen. They possess a lower proportion of oxygen and a higher proportion of hydrogen than carbohydrates. Lipids are ideal storage compounds yielding twice as much energy as carbohydrates when fully oxidised (respired), and, being insoluble, are osmotically inactive. Although insoluble in water, they are soluble in a range of organic solvents e.g. alcohol. Lipids in the human diet, and when stored in adipose tissue under the skin, are in the form of triglycerides (or triacylglycerols), which consist of glycerol and three fatty acids. Structure of Glycerol: The general formula of a fatty acid is R-COOH. The R represents a hydrocarbon chain and COOH is a carboxylic acid group. Structure of fatty acids: 6 Formation and hydrolysis of a triglyceride molecule Condensation: The three fatty acids are usually different, and many different kinds of triglycerides occur naturally. Most fatty acids contain 16, 18, or 20 carbon atoms, but the positions and number of double carbon-carbon bonds vary. Most natural plant and animal ‘fats’ contain a complex mixture of individual triglycerides giving them very different properties. Hydrolysis Lipids can be hydrolysed into fatty acids and glycerol by: Heating with acid or alkali. Using the enzyme lipase Phospholipids Phospholipids are lipids containing a phosphate group. In the commonest type of phospholipid, a phosphate group replaces one of the fatty acid molecules. A phospholipid molecule consists of one glycerol, two fatty acids and a phosphate group joined in a condensation reaction. Formation of a phospholipid 7 The phospholipid molecule has a polar hydrophilic ‘head’ containing the phosphate group. Non-polar hydrophobic ‘tails’ consisting of long fatty acid chains. Phospholipid layers A thin layer of phospholipids in contact with water forms a single layer as the hydrophilic heads are attracted to the water whereas the hydrophobic tails are water repellent and project outwards. The phospholipids can arrange themselves into micelles or sheet structures. In the cell membrane a bilayer (two layers) of phospholipids is present as the hydrophobic tails are attracted to each other and away from water. 8 Proteins Proteins contain carbon, hydrogen, oxygen, nitrogen and sometimes sulphur. Amino acids are the sub-units (monomers) from which proteins (polymers) are formed. All amino acids have an amino group and a carboxylic acid group, but differ in the atomic group R. There are 20 different commonly-occurring amino acids in living organisms. Amino acids are joined together by peptide bonds (-CONH-) formed by condensation reactions. Two amino acids joined together form a dipeptide. Many amino acids joined together in a chain form a polypeptide. A protein may consist of one or more polypeptides Generalised structure of an amino acid A dipeptide is formed by a condensation reaction between two amino acids. 9 The condensation process can continue to produce a polypeptide. A part of the peptide backbone NCCNCCNCC is shown below. Hydrolysis of proteins Proteins can be hydrolysed by heating with acid or by using enzymes (proteases). These enzymes include: endopeptidases which hydrolyse internal peptide bonds in proteins to produce smaller polypeptides; exopeptidases which remove single amino acids from the ends of the polypeptide chains, eventually producing a dipeptide and amino acids. 10 The structure of proteins Proteins vary in the number, type and sequence of amino acids they contain. This produces a vast number of different protein molecules. They can be classified according to their structure. Primary structure This refers to the sequence of amino acids in the polypeptide chain; this sequence determines the specific shape of the protein. Secondary structure This represents the folding or coiling of the polypeptide chain as a result of hydrogen bonding between amino acids. Secondary structures include A the helix and B the -pleated sheet. 11 Tertiary structure The is formed by the further folding and coiling of the secondary structure due to hydrogen bonds, ionic bonds and disulphide bridges. Quaternary structure The quaternary structure relates to highly complex proteins consisting of more than one polypeptide chain. The chains are held together by ionic and hydrogen bonds and hydrophobic interactions. For example, adult haemoglobin consists of four polypeptide chains of two types; two alpha chains (each contains 141 amino acids) and two beta chains (each contains 146 amino acids). Denaturation of proteins Denaturation is an alteration in the tertiary structure of a protein. This loss of the three dimensional shape of the protein is associated with the breaking of bonds holding the tertiary structure together. It is often irreversible and the protein no longer functional. It can be caused by high temperatures above the optimum, breaking of hydrogen Changes in pH away from the optimum, breaks ionic bonds. Reducing agents can break disulphide bridges. Heavy metal ions can bind to sites on the protein and bring about changes in shape. 12 Types of bonds and interactions found in proteins In all these diagrams the polypeptide backbone is shown as a coiled spring, the side chains responsible for the bond shown are highlighted as boxes and the side chains (R groups) shown. Hydrogen bonds may form between the C=O and N-H groups of the backbone (flanking the peptide bond) these occur within the same polypeptide chain and are responsible for the formation of secondary structures α helix and β sheet. Hydrogen bonds may also form between the R groups of polar amino acids Ionic bonds form between a positive Hydrophobic regions form as hydrophobic side and negatively charged side chain of chains tend to exclude water and are attracted to basic and acidic amino acids each other. respectively. A disulphide bond forms as result of an oxidation reaction between two cysteines 13 Cytology Eukaryotes Eukaryotic cells are characterised by the presence of a nucleus and many cell organelles. Cell structure – organelles Cell organelles found in eukaryotic cells include the nucleus, centrioles, mitochondria (singular mitochondrion), Golgi body, lysosomes, rough and smooth endoplasmic reticulum and ribosomes. 14 Nucleus The nucleus contains the genetic material, DNA, determining the development, structure and function of the cell. The nucleus is bound by a double membrane, the nuclear envelope which has nuclear pores allowing communication with the cytoplasm. The nucleus contains nucleoplasm in which chromatin (DNA and protein), and nucleoli are interspersed. Nucleoli are composed of proteins and nucleic acids and are regions of RNA synthesis. In a dividing cell the chromatin is in the form of chromosomes The nucleus controls cell activities including; protein synthesis, cell division and the production of ribosomes. 15 Mitochondria Mitochondria are involved in the biochemical reactions of aerobic respiration yielding ATP. They are variable in shape and size, but are often rod shaped and between 1-10m in length. They are bounded by two membranes forming an envelope around an inner matrix. Between the outer smooth membrane and the inner folded membrane is the inter-membrane space. The folds of the inner membrane are called cristae (individual = crista) and provide a large surface area for the stalked particles they possess. These stalked particles contain enzymes associated with ATP production by the electron carrier system (oxidative phosphorylation.) The matrix contains the enzymes of the Krebs cycle (aerobic respiration) and also contains mitochondrial DNA and ribosomes. The DNA contains the information required for the mitochondria to replicate and the ribosomes are required for protein synthesis. Cells requiring large amounts of ATP for processes such as movement (muscle contraction), biosynthetic reactions e.g. glycogen synthesis in the liver, or cells involved in active transport have numerous mitochondria. 16 Centrioles Centrioles are small hollow cylinders (about 0.3 – 0.5μm long and about 0.2μm in diameter), each containing 9 triplets of microtubules. They occur in pairs in most animal cells. At the beginning of nuclear division the centrioles replicate themselves and the two pairs migrate to the opposite poles of the spindle, the structure on which the chromosomes line up. The spindle itself is made of microtubules (‘spindle fibres’) which control the separation of the chromosomes or chromatids. Ribosomes Ribosomes are very small organelles (20nm diameter), consisting of two subunits, one large and one small, each made up of protein and ribosomal RNA. Ribosomes can be present in the cytoplasm singly, in a chain (polysomes) or attached to the RER. Ribosomes are used in protein synthesis, joining amino acids together Endoplasmic reticulum (ER) ER consists of flattened membrane sacs called cisternae, forming an internal transport system in the cell. The surface of the rough endoplasmic reticulum (RER) has ribosomes that produce proteins that are transported through the cisternae. The proteins produced by the RER are usually secretory proteins i.e. proteins exported out of the cell. These secretory proteins are often sent to the Golgi body for packaging. The smooth endoplasmic reticulum lacks ribosomes and is involved in the production and transport of lipids 17 Golgi body As with the endoplasmic reticulum the Golgi body consists of a stack of flattened, membrane-bound sacs called cisternae. The cisternae are continually being formed at one end and pinched off as vesicles at the other end, Secretory cells have large Golgi bodies The Golgi body acts as an internal processing and transport system and has numerous functions including: Production of glycoproteins. Packaging and secretion of proteins e.g. enzymes. Formation of lysosomes. 18 Lysosomes Lysosomes are spherical (0.2- 0.5μm) organelles found in most eukaryotic cells. They are surrounded by a single membrane and are simple sacs containing digestive (hydrolytic) enzymes such as proteases, nucleases and lipases which break down proteins, nucleic acids and lipids respectively. The enzymes have to be kept apart from the rest of the cell or they would destroy it. The enzymes contained within lysosomes are synthesised on rough ER and transported to the Golgi apparatus. Golgi vesicles containing the processed enzymes later bud off to form the lysosomes. Functions: 1. Digestion of material taken in by endocytosis - Lysosomes may fuse with the vesicles or vacuoles formed by endocytosis, releasing their enzymes into the vacuole and digesting the material inside. The products of digestion are absorbed by the cytoplasm leaving undigested remains. The vacuole usually migrates to the cell surface membrane and releases its contests (exocytosis). 2. Autophagy – This is the process by which unwanted structures within the cell are engulfed and digested within lysosomes. They are first enclosed by a single membrane, usually derived from smooth ER, and this structure fuses with a lysosome to form an ‘autophagic vacuole’ in which the unwanted material is digested. This is part of the normal turnover of cytoplasmic organelles, old ones being replaced by new ones. 3. Release of enzymes outside the cell – sometimes the enzymes of lysosomes are released from the cell. For example, sperm contain a special lysosome called the acrosome. This releases its enzymes outside the cell to digest a path through the layers of cells surrounding the egg just before fertilisation. 19 Epithelial tissues Epithelial tissues line the inside or outside of organs and may have a range of functions. They can be classified according to shape into three basic types; squamous, cuboidal and columnar. Columnar epithelial cells can be found in the small intestine and proximal convoluted tubule of the kidney. They are cylindrical in shape and are specifically adapted for the absorption of small molecules, mainly by active processes. The cell membrane is folded into microvilli providing a large surface area. Numerous mitochondria are present providing ATP for the active uptake of molecules such as amino acids and sugars. 20 Techniques in Cytology Differential Centrifugation Centrifugation separates structures of different size and density. Differential centrifugation involves centrifuging at different speeds and can be used to separate and isolate the different organelles in a cell. The cells are first broken open by grinding (homogenising) a tissue such as liver in an ice cold, isotonic, buffer solution using a blender. An isotonic solution prevents the osmotic movement of water in or out of organelles, which might cause them to burst or shrivel. A low temperature prevents the action of enzymes within the cells that might cause self-digestion (autolysis) of the organelles. 1. The resulting suspension is then poured into a tube and spun in a centrifuge at a low speed to remove cell debris such as cell walls of plant cells which form a sediment (pellet) at the bottom of the tube. 2. The supernatant liquid above the sediment contains suspended organelles and is spun at a higher speed (force) for a longer time period. 3. The heaviest organelles i.e. nuclei are forced to the bottom forming sediment whilst the lighter organelles remain suspended in the supernatant. 4. The supernatant is again removed and spun at a higher speed for a longer time period. This procedure is repeated increasing the speed and time of centrifugation in order to obtain a series of pellets containing organelles of decreasing size. 5. The organelles are usually isolated in the order; nuclei, chloroplasts (if plant cells are used), mitochondria, endoplasmic reticulum and finally ribosomes. 21 Electron Microscopy The use of the electron microscope has proved invaluable as a means of investigating the fine structure (ultrastructure) of a cell. The electron microscope uses a beam of electrons focused by electromagnets as opposed to the focusing of light rays by lenses in light microscopy. COMPARISONS OF ELECTRON AND LIGHT MICROSCOPES FEATURE ELECTRON LIGHT Radiation Source Wavelength Maximum resolution Maximum useful magnification Lenses Specimen Advantages of electron microscopy The maximum useful magnification is much higher than with light microscopy. Electron microscopy uses electrons that have a shorter wavelength than light and thus greater resolution i.e. the ability to distinguish between two close objects. The light microscope has a maximum resolution of around 200nm whereas with the electron microscope it is 0.5nm. At higher magnifications images become blurred when using light microscopy due to poor resolution (see diagram below). Disadvantages of electron microscopy As a vacuum is required living specimens cannot be seen. Preparation and staining techniques are more complicated than with light microscopy and can produce artefacts (‘false images arising from poor technique’). Expensive to buy and expert training is required in their use. 22 Calculating the size of cellular structures Image Magnification = Actual Image Actual = Magnification Image = Actual x Magnification When asked to calculate the actual size or magnification of a cellular structure in an exam there are a few simple rules to follow. Rule 1 If required rearrange the formula, Image = Actual x Magnification as shown above. Note: It’s easy to remember the formula as I am i.e. I = a x m Rule 2 If required it is often easier (although not essential) to make any measurements in mm. This allows for easier conversion to micrometres (µm) = 10 -6 metre which is the unit most often used in measuring the size of cellular structures. Rule 3 Ensure you convert any measurements you make (or any the examiner may provide) to the same units required for the answer e.g. convert millimetres (mm) into micrometres (µm). It is often better to do this before carrying out the calculation. Units of Measurement 1 cm (centimetre) = 10-2 metre 1 mm (millimetre) = 10-3 metre Make sure you know these measurements -6 1 µm (micrometre) = 10 metre and can convert one into the other. 1 nm (nanometre) = 10-9 metre 23 Converting units X1000 x1000 mm µm nm /1000 /1000 Exercise Measurement Convert into Answer 1m mm 1000 mm 650 mm µm 17 mm nm 25.5 nm mm 398 µm mm 5.5 nm µm 1.5 m µm For example You are asked to calculate the actual size of an organelle (e.g. a chloroplast) in micrometres (µm) = 10-6 metre. You are given the magnification as x 30 000 Rule 1 – Rearrange the formula Actual size = Image size Magnification Rule 2 – Measure the image size e.g. = 90 mm Rule 3 – Convert to units required in the answer i.e. micrometres 90 mm = 90 x 103 (i.e. x 1000) micrometres (µm) = 90 000 Therefore Actual size = Image size = 90 000 µm = 3.0 µm Magnification 30 000 24 The Cell Membrane The entry and exit of substances to cells is controlled by the cell membrane or plasma membrane, which surrounds the cytoplasm of a cell. The structure of the plasma membrane is described by the fluid-mosaic model. The plasma membrane The cell membrane consists of protein and phospholipid. The phospholipid molecules form a bilayer but are constantly moving about giving a fluid structure. The protein molecules are unevenly distributed throughout the membrane forming a mosaic. The selective permeability of the cell membrane is related to the type and distribution of protein and phospholipid molecules present in the membrane The phospholipid bilayer enables lipid soluble molecules rapid passage through the cell membrane but restricts passage of ions and polar molecules. 25 Transport across membranes Movement of substances into and out of cells through the plasma membrane can occur by diffusion, facilitated diffusion, osmosis and active transport. Diffusion Diffusion is the net movement of molecules from a high concentration to a low concentration until the molecules are equally distributed. Diffusion is a passive process i.e. it does not require energy from respiration. Gaseous exchange occurs via diffusion. The rate of diffusion across a membrane occurs at a rate determined by a number of factors. Rate of diffusion is proportional to Surface area x Concentration difference (gradient) Thickness of exchange surface The rate of diffusion is increased by a higher concentration gradient, a large surface area e.g. microvilli, and by a short diffusion distance e.g. a single cell layer. 26 Facilitated diffusion This process allows the transport of polar molecules such as glucose and amino acids across membranes. It involves the use of channel proteins and carrier proteins Facilitated diffusion is a passive process i.e. it does not require energy It is not inhibited by respiratory inhibitors such as cyanide. Carrier proteins Channel proteins 27 Active Transport Active transport is the movement of molecules or ions through a partially permeable membrane by carrier proteins against a concentration gradient. Carrier Proteins possess a specific tertiary structure complementary to polar molecules e.g. glucose or amino acids, aiding their transport by facilitated diffusion and active transport. It requires energy in the form of ATP produced during respiration. Factors which decrease the rate of respiration will therefore decrease active transport: 1) Lowering of temperature. 2) Lack of oxygen. 3) Metabolic and respiratory inhibitors e.g. cyanide. Cells involved in active transport possess a large number of mitochondria to provide the ATP required via respiration. 28 Osmosis Osmosis is the net movement of water molecules across a partially permeable membrane Osmosis is essentially the diffusion of water molecules across a partially (selectively) permeable membrane. In terms of solute concentration - osmosis is the movement of water from a low concentration of solute molecules to a high concentration of solute molecules. In terms of water - osmosis is the movement of water from a high water potential to a low water potential. 29 Water potential Water potential can be defined as the potential (tendency) of water molecules to leave a solution by osmosis. The greater the concentration of ‘free’ water molecules in a cell or solution the higher is its water potential. In osmosis water moves from a higher (less negative) water potential to a lower (more negative) water potential through a partially permeable membrane 30 Solutions A and B separated by a partially permeable membrane Solution A Solution B 20 % Glucose 70 % Glucose Lower solute concentration Higher solute concentration Higher water potential Lower water potential Solution A has a higher water potential is as it has a lower concentration of solute molecules. Solution B has a lower water potential as it has a higher concentration of solute molecules. Water movement would occur by osmosis from solution A (higher water potential) to solution B (lower water potential). Net water movement would continue until the solutions were isotonic i.e. of equal water potential. At this point water movement would still occur, but would be equal in both directions, i.e. there would be no net movement of water. The diagram below shows three cells with different water potentials. Add arrows to indicate the movement of water by osmosis between these cells. -350Ψ -300Ψ -375Ψ 31 Osmosis and Fluid / Electrolyte Balance in the Body Since water potential determines the movement of water by osmosis into and out of cells, it follows that suitable water potentials must be maintained in the body’s tissue fluids. This is an aspect of homeostasis (of which much more later in the course). The diagram shows the typical arrangement of tissues and associated vessels: Fluids / Solutions can be described, relative to the normal water potential of tissue fluid as: Hypertonic Hypotonic Isotonic We will be examining the importance of electrolyte balance by using some case-studies – these will be provided separately. 32