Food Packaging Materials Physical Properties PDF
Document Details
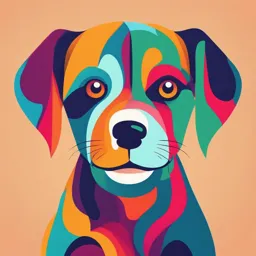
Uploaded by ThriftyCliff
Universiti Malaysia Terengganu
Tags
Summary
This document discusses the physical properties of food packaging materials, focusing on thermal properties such as thermal conductivity, heat capacity, thermal expansion, and transition temperatures. It examines the behavior of different materials under thermal stress and provides specific values and examples for various materials used in food packaging applications.
Full Transcript
Physical properties of packaging materials.. packaging’s behavior under physical stress or treatment. … do not involve modification or change of chemical structure. Classification: – THERMAL: describe the behavior of a material to heat. Thermal conductivity, thermal capacity, speci...
Physical properties of packaging materials.. packaging’s behavior under physical stress or treatment. … do not involve modification or change of chemical structure. Classification: – THERMAL: describe the behavior of a material to heat. Thermal conductivity, thermal capacity, specific heat, heat of formation, heat of combustion, transition temperatures, sealing properties, thermal strain. – ELECTROMAGNETIC (electric; optical): describe the behavior of a material to electromagnetic radiation. Refractive index, transparency/absorption/reflectivity, gloss, haze, behavior to microwave, behavior to ionizing radiation. – MECHANICAL: describe the behavior of a material to mechanical forces. Coefficient of friction, mechanical strength, density, shock resistance, elongation. – DIFFUSIONAL (include sorption): describe the behavior of mass transport phenomena. Solubility, permeability, diffusion. Thermal properties Thermal properties Describe the heat transport behavior and the change in material state under application of thermal energy. Heat transport phenomena often occur: – During thermal treatment (pasteurization, sterilization). – During long term storage with temperature variations. – During package fabrication (soldering, thermoforming, molding). Thermal properties – Thermal conductivity The tendency of a solid body to exchange heat by conduction (the main mechanism of heat transfer through a packaging material). – The easiness of thermal flow between two points inside the solid, due to temperature gradient. – The rate of heat transfer through unit area of unit thickness for unit temperature difference. Fourier’s Law: dQ / dt kA(dT / dx) – Q = heat, t = time, A = surface area, T = temperature, x = distance in thermal flow direction, k = thermal conductivity. – Negative sign: heat flows from a point of high temperature to a point of low temperatures. – Common units: kcal m-1 h-1 oC-1 or J m-1 s-1 oC-1 or W m-1 oC-1 Thermal properties – Thermal conductivity Typical thermal conductivity values of some materials used for packaging. Material Thermal conductivity (W/m oC) Aluminum (25oC) 237.00 Stainless steel 54.00 Glass (30oC) 0.96 LDPE (density = 920 kg/m3) 0.48 Expanded LDPE (density = 43 kg/m3) 0.053 Wood (oak) 0.14 Cork 0.04 METAL: High thermal conductivity - due to the ability of metal ions to absorb heat energy by vibrating faster collision with neighboring ions transfer of heat energy. Thermal properties – Thermal conductivity Different thermal conductivity may affect the heating or cooling rate in heat processes, e.g. pasteurization or sterilization of packaged food.. Metallic materials have high thermal conductivity due to the electron mobility in metallic bonds. Organic materials, e.g. plastics and wood products, have low thermal conductivity due to their atomic and intermolecular bonds. Still air (200C) has k of 0.02 W m-1 0C-1 very low thermal conductivity Thus.. corrugated board and expanded polystyrene are better insulating materials than solid board and homogenous plastics (at the same thickness). Thermal properties – Heat capacity (Cp) Measures the amount of heat necessary to produce a unit change of temperature in a body. Q = Cp ∆T – Q = transferred heat, ∆T = change in temperature, Cp = heat capacity. – Common units: kcal 0C-1 or J K-1 When it is referred to for unit mass (kcal kg-1 oC-1) and not for a body, it is often called specific heat. Metallic and inorganic materials increase their temperature with a less amount of energy than organic materials. Amorphous solid has larger heat capacity than crystalline. – Heat capacity of semi crystalline materials, e.g. plastics and cellulosic, changes with the distribution between amorphous and crystalline phase fractions. Larger heat capacity = better thermal insulation better protection for sensitive foods under heat-shock conditions. Thermal properties – Heat capacity (Cp) Typical specific heat of some packaging materials Material Specific heat (kJ/kg 0C) Stainless steel 0.46 Glass 0.84 Aluminum 0.90 LDPE 2.30 Wood 2.72 Material Spatial organization Glassy Amorphous Ceramic Crystalline/amorphous Cellulosic Crystalline (pure cellulose) Metallic Crystalline Plastic Amorphous/crystalline Thermal properties – Thermal expansion (linear and volumetric) Coefficient of linear expansion (α) and volume expansion (β): the relative changes in length and volume for a temperature change at constant pressure. L = L0 (1 + α ∆T) V = V0 (1 + β ∆T) In general, α = β/3 The coefficients depend on the temperature. A change in packaging dimensions (due to temperature change) may have dramatic effect, for example: – Linear expansion or shrinking of belts assuring a load during shipment serious economic consequences. – Expansion of glass jars in a constrained space breakage. – Repeated exposure of flexible package to heat and cold expansive phenomenon. Thermal properties – Thermal expansion (linear and volumetric) Linear thermal expansion coefficients of some packaging materials (per 1 oC) Material Thermal expansion α (1/oC) Polypropylene 110 x 10-6 Rubber 77 x 10-6 Aluminum 24 x 10-6 Polyester 17 x 10-6 Steel 13 x 10-6 Glass 6 x 10-6 Wood 4 x 10-6 Ceramic 3 x 10-6 Thermal properties – Tolerable thermal range The lowest limit: the temperature at which the material shows the maximum fragility affordable during the commercial distribution. Highest limit: the temperature that begins to cause a physical distortion of the object, a lack of performance, or the beginning of chemical change (e.g. browning, oxidation, etc). Also called ‘service temperature’ More typical of the final package than of the raw material, due to method of manufacturing (e.g. degree of crystallization, orientation, additives) Thermal properties – Tolerable thermal range Lowest and highest temperatures tolerable for some packaging materials: Material Lowest tolerable T (0C) Highest tolerable T (0C) Stainless steel -45 600 Glass (boron aluminates) Depends on thermal 490 Glass (sodium silicate) shock 460 Aluminum (anodized) -40 350-400 Fluoropolymers -100 260 Polyester (crystallized PET) -60 220-230 Cellophane -18 180 Polyamide (Nylon 6) -10 60-160 Polyester (amorphous PET) -60 65-70 HDPE -90 60-85 Polyvinyl chloride -15 65-85 Thermal properties – Transition temperatures (Tm, Tg) The temperatures at which a change in the state of substance occurs under standard pressure. For packaging materials, important transition temperatures are: melting point, glass transition, and crystallization temperature. Adjustment of degree of crystallization makes it possible to change some important features of: – Plastics: diffusional, mechanical, thermal properties. – Ceramics: strength, fragility. – Metals: ductility. Thermal properties – Transition temperatures (Tm, melting point) Tm (melting point): transition temperature of the phase change from a solid to a liquid state. – Important for both inorganic and organic materials. – Crucial for materials intended to take a final form in some steps of package preparation through solidification from a liquid or semi-solid state. – Only materials with a true crystalline organization have sharply defined melting temperatures. – Amorphous, non-crystalline materials have no melting point. They soften with heating to reach progressively a fluid form, e.g. glasses and amorphous polymers. Variation of stiffness with increasing temperature for amorphous and crystalline materials Amorphous materials: as T increases, they become easier to shape with reduction of stiffness. Crystalline materials: as T increases, they are still not easily deformed until the melting temperature is reached. Thermal properties – Transition temperatures (Tg, glass transition temp.) Tg (glass transition temperature): a temperature at which a substantial change in molecular chain mobility takes place. – It is below melting point. – Below Tg: the molecules show minimum mobility and significant decrease of the free volume maximum fragility, low diffusion properties. – Around Tg the materials show an unusual thermal sensitivity. – Important for polymers. At ambient temp.; If Tg < room temperature: rubbery (soft and flexible). If Tg > room temperature: glassy (more rigid). Melting and glass transition temperatures for some packaging materials. Material Tm (0C) Tg(0C) Glass - 1450* Stainless steel 1430** - Aluminum 660** - Polyester 267 69 Tin 232** - Polypropylene 176 -18 Polyvinyl chloride 212 87 Polyamide (Nylon 6.6) 265 50 Cellulose - 40 Synthetic rubber (1-4 - -70 polyisoprene) 100* Polymethyl methacrylate - 94* Polystyrene atactic - -125 HDPE 137 -110 Linear LDPE 112 *Completely amorphous materials ** No glass transition temperatures for metal Thermal properties – Heat of combustion (QC) The amount of thermal energy Heat of combustion for some packaging released from the total materials and fuels combustion of a material. Material QC ( MJ/kg) Related with the environmental impact of the material. Polyester 33 Only applies to organic Polypropylene 46 materials (cellulosic and PVC 20 plastics). Cellulose 15-30 Measured by burning the material in a bomb calorimeter Wood 16-20 pressurized with a large excess Fuel oil 43 of oxygen. Municipal solid waste 25 Electromagnetic properties Electromagnetic properties Electromagnetic radiation (= radiation): the energy that propagates through a material with change in electric and magnetic fields. The energy is characterized by wavelengths and/or frequency of the disturbance of coupled electric and magnetic fields. – Cosmic rays (the shortest in wavelengths), γ and X rays, UV, visible, infrared lights, microwaves, radio waves (the longest wavelengths). Food packaging: concern on how and how much these energy are absorbed, reflected or transmitted by the packaging materials. – Infrared absorption spectrum identification of synthetic polymers. – Transparency, clarity, and color of plastic films and glasses aesthetic/optical properties – Gloss of metallized surface homogeneity evaluation of a thin layer coating – X ray absorption to measure thickness of continuously extruded plastic films. Properties: refraction index; transparency; gloss; haze; absorption/transmission behavior to UV, visible, infrared and microwave radiations. Electromagnetic properties Electromagnetic radiation as relevant use in packaging application Radiation Frequency Wavelength Interest (Hz) (nm) γ rays 1021 – 1018 10-4 – 0.1 Material sterilization X rays 1019 – 1016 0.01 – 10 Thickness measurement Ultraviolet 1016 – 1015 10 – 400 Food protection performance Visible 1015 400 – 800 Color, transparency, etc. Infrared 1015 – 1012 800 – 20000 Identification Microwave 1011 - 1010 104 – 105 Heating, etc. Electromagnetic properties – Refractive index (n) The Law of Refraction (Snell’s Law): when a monochromatic light passes from a medium m1 to another m2 of higher density, the angle of incidence (Фi ) and the angle of refraction (Фr) are different. Фr is lower because the velocity of the light in m1 (v1) decreases in m2 (v2). – The ratio (n) is called index of refraction or refractive index. – n = sin Фi / sin Фr = v1 / v2 Absolute index of refraction of a material: the ratio of the speed of light in a vacuum to the speed of light in the material. The higher the index of refraction of a material the slower the light travels through it. Electromagnetic properties – Refractive index (n) Relative index of refraction: the ratio of the absolute indices of refraction for light traveling from any medium to another. – Involved wavelength, temperature and pressure. – Generally used for an objective characterization of clearness of glasses and transparent plastics. – The higher the index of refraction of a transparent material: the more the incidence light will deviate toward the normality of the interface the clearer the vision. Schematic apparatus for determining relative refractive index Electromagnetic properties – Refractive index (n) Relative refractive index for some packaging materials and references Material Refractive index (200C, 589.3 nm) PbO 2.5-2.7 Diamond 2.42 Glass 1.52-1.89 Polyester 1.64 Polypropylene 1.50 Polycarbonate 1.59 Polymethyl methacrylate 1.49 Polyamide (nylon 6.6) 1.57 Water 1.33 Air 1.00 Electromagnetic properties – Transparency (%T) An aesthetic character wanted and appreciated by the consumers. Can be measured as specular transmittance (= the transmitted radiant flux measured includes only that in the same direction as that of the incident flux, with a deviation of an angle less than 0.10) of an electromagnetic radiation between 540 and 560 nm, central in the visible light spectrum. ‘see through clarity’. Inversely correlated to the thickness of the material. Electromagnetic properties – Transparency (%T) Beer’s Law: I = Io exp (-kcl) – I = the intensity of the radiation – Io = the original intensity of the radiation – l (cm) = thickness of a material – k (cm-1 M-1) = molar absorptivity – c (M) = molar concentration Schematic apparatus for transparency determination Electromagnetic properties – Transparency (%T) Ratio I/Io = transmission factor = T – Transmittance = %T Transparency of flexible packaging materials is greatly affected by: – Degree of crystallinity – Homogeneity – Amount of some ingredients (e.g. pigments and inert fillers) – Thickness of some coatings (e.g. varnishes, very thin metallic layers) Transparency can be modified, not only for the optical/hedonistic property, but also for the protection of light-sensitive foods. Absorbance = Optical density (OD) – OD = log10 (1/T) – OD = log10(e) x kcl – OD is directly proportional to the concentration of absorbing ingredient. Electromagnetic properties – Transparency (%T) Transparency of some packaging materials Material %T (spectacular transmittance at 550 nm) Glass (180 µm) 92.1 Polypropylene biaxially oriented (28 µm) 92.0 Cellophane (20 µm) 92.0 Polyamide (nylon 6.6.) biaxially oriented (18 89.2 µm) 87.3 PVC (40 µm) 82.6 Polyamide (nylon 6.6) cast (50 µm) 82.3 Polypropylene cast (40 µm) 81.8 Polyester (25 µm) 43.5 Polystyrene (38 µm) 21.4 Polytetrafluoroethylene (36 µm) 8.7 Pergamine (45 µm) 3.1 White polypropylene (36 µm) 1.3 Vegetable parchment (78 µm) Veg. parchment Electromagnetic properties – Transmittance/Absorption Spectra in UV, VIS and IR The behavior of a transparent packaging material in response to the light irradiation. Transmission/absorption spectra allows the quantification of the transparency at any wavelength to know which proportion of the light energy is absorbed by the material. – Electromagnetic radiations (UV/VIS) are absorbed mostly by atoms whose electrons are undergoing transitions to higher energy state. – These information are related to: Atomic structure of the material Color of the material The kind of protection the material can give to light-sensitive food. – Electromagnetic properties in UV or visible light can be measured by various types of spectrophotometer instruments. Electromagnetic spectrum Electromagnetic properties – Transmittance/Absorption Spectra in UV UV radiations (200 – 400 nm) – Might be dangerous both for foods and packaging materials, since it can: Activate the mechanisms of photo-oxidation of lipids. Damage other photo-sensitive compounds, e.g. pigments and vitamins. In several plastics and cellulosic materials: changes in color, gloss, loss of flexibility, and enhancement of migration. – Even low levels of UV radiation in natural and artificial lights (incandescence and fluorescence lamps) have a serious problem for various foods during shelf life. – Many additives are available for plastics and glasses to the reduce the UV transmissions that work by: Absorbing UV radiation Emitting less dangerous longer wavelength light by the quenching phenomenon (taking down a UV excited substance to a stable state without any unwanted side reactions), or Scavenging the radicals formed by UV irradiation. Why shorter wavelength of radiation is more dangerous?? The higher the frequency, the shorter wavelength the more energetic is the light/ radiation. Enough energy to strips electrons from atoms (ionizing radiation) The more energetic a ray of light is, the more that light will blast apart the bonds between molecules. Initiate many chemical reactions. For microwave radiation high energy vibrate water molecules producing large heat Electromagnetic properties – Transmittance/Absorption Spectra in VIS and IR Visible light radiations (400 -800 nm) – May risk color stability, flavor maintenance and general appearance of foods during their shelf life. This region should be considered for protective purposes more than for aesthetic (e.g. see-trough clarity or color brightness). Coloring of transparent materials can change their visible transmission spectra. Infrared radiation – Absorption spectrum is unique since it reflects molecular structure of materials used for identifying substance. – Region: wavelength between 800 – 50000 nm (0.8-50 µm). – Divided into ‘near’, ‘medium’, and ‘far’ IR. – Medium IR between 3000 – 25000 nm, where the absorption is mainly due to the vibration levels of molecules, is the region where identification of organic molecules is mostly obtained. Electromagnetic properties – Haze Haze: degree of opacity (for transparent materials, e.g. plastic films, glass). – Measured as the percentage of transmitted light which deviates more than 2.50 from the incident radiation by forward scattering in passing through a specimen. – An aesthetic characteristic of transparent materials. – Represents the homogenous distribution or the dimension of constituents, which may lead to light-scattering phenomena decreased transparency. Haze = 100 x (Is / IT) Is = the intensity of scattered light (with the trap light) IT = the total light transmitted (without the trap light) Schematic apparatus for haze determination Electromagnetic properties – Haze Haze values for some packaging materials Material Haze (%) Glass 0-0.2 Polystyrene 0.1-3.0 LDPE (50 µm) 30 Polypropylne (25 µm) 2.50 HDPE (30 µm) 5-6 Polycarbonate 0.5-2.0 Cellophane 3.0 Polyester (15-30 µm) 2.5-5.0 LDPE Electromagnetic properties – Gloss Specular gloss: attribute of a surface indicating shiny appearance of packaging materials or objects, transparent or opaque. – Defined as the ration of luminuous flux reflected from a specimen at a specific solid angle to the luminuous flux incident at the same angle, i.e. in the specular direction. – The higher the gloss value, the shinier the surface, the more appreciated the colored print or metalized surface of a package. More relevant for aesthetic purposes than for functionality. Gloss values are used mainly in smoothness evaluation of colored or uncolored surfaces, typically for some packaging materials. Schematic apparatus for gloss determination Electromagnetic properties – Gloss Gloss values for some packaging materials Material (angle, thickness) Gloss units PVC (200, 20 – 50 µm) 120-160 Polyvinylidene chloride (450, 60 µm) 115 White polypropylene (25 µm) 70 Polypropylene (25 µm) 80 Ionomers (200) 25 White pp pvc pvdc Electromagnetic properties – Behavior to ionizing radiations Some electromagnetic radiation may promote ionization when they pass a material change some properties of the materials. – It can happen directly from radiations carrying a charge like accelerated electrons (β radiation) or indirectly from their high energy (X and γ radiation). Possible consequences: a) Fragmentation of macromolecules: Positive: lethal effect on unwanted microorganisms – γ radiations are used to sterilize packaging materials and also packed foods. Negative (in packaging materials): lead to intense migration, loss of mechanical resistance, increased permeability. b) Reticulation (extensive networking by side chain bonds) General improvement of the performance of packaging materials: stronger, less permeable, more inert. Actually, some plastic films and glasses are submitted to ionizing radiation to improve their properties: – For plastics: increase in gas barrier properties, increase in mechanical properties. – For glasses: less fragility. Electromagnetic properties – Behavior to ionizing radiations Behavior of some packaging materials to ionizing radiation Material Behavior Polyethylene Networking Polystyrene Networking Polyester Networking Ethylene vinyl acetate Networking Polytetrafluoroethylene Networking Glass Networking Polyvinylidene chloride Fragmentation Cellulose Fragmentation Fragmentation and reticulation phenomena in PVC Both a polymer under the effects of Cellulose esters Both electromagnetic radiation Polycarbonate Both Polyamide Both Polypropylene Both Electromagnetic properties – Behavior to microwaves Microwaves: electromagnetic radiations with frequencies between 300 MHz and 30 GHz. Food packages are submitted to microwave irradiation for heating, cooking, defrosting. Behavior of materials under microwaves is different according to their chemical composition and the presence of some impurities. If the packaging material has residual moisture or contains free ions or polar molecules able to orientate according to the direction of propagation of electromagnetic fields, the heat will increase the material temperature even to melting not desired. Electromagnetic properties – Behavior to microwaves Behavior of some packaging materials to microwave irradiation Material Behavior Notes Metals Absorbing/reflective Risk of electric arcs Glass Transparent - Cellulose Transparent/absorbing Heat dissipation Polyethylene Transparent Risk of melting Polyester Transparent - Polyamide Transparent - Mechanical properties Mechanical properties How a material reacts to respond to the application of forces (particularly the material’s behavior under mechanical loads). Factors: – Type of force involved. – Time length of application of force. – Magnitude of force. – Direction of force. – Source of force. Consist of: – Resistance of materials to possible stresses (e.g. stiffness, fragility, burst strength, resilience). – Performance characteristics (elasticity, coefficient of friction, flex resistance, tackiness, cushioning properties). Important for: – Selection of materials. – Design and production of packages – Optimization of transport and distribution. – Shelf life prediction. Mechanical properties – Density and related properties Weight: the force which always acts on whatever material and its body product of mass and acceleration of gravity. Density (d): a way to evaluate concentration in a material – Measured by the mass per unit volume (g cm-3 or kg m-3) Bulk density (kg m-3): mass per total volume of the material consisting of solids and voids (e.g. plastic foams, expanded polymers, corrugated boards, and raw materials in pellets or granules). Grammage (G) = basis weight: mass per unit area of surface – Widely used for paper and paperboard, plastic films, coatings and varnishes. – The ratio of grammage to density = thickness (l) G (g m-2) / d (g cm-3) = l (µm) Density of some packaging materials Material Mass per unit volume (g cm-3) Steel 8.0 Tinplate 8.0 Aluminum 2.70-3.20 Glass 2.40-2.80 Cellophane 1.50 Paper 0.75-1.15 Polyethylene 0.91-0.94 Polyester 1.35-1.40 PVC 1.20-1.35 Polyamide (Nylon 6) 1.14 Polypropylene 0.88-0.91 Polystyrene 1.04-1.08 Wood (balsa) 0.11-0.14 Wood (oak) 0.60-0.90 Cork 0.22-0.26 Mechanical properties – Coefficient of friction Friction: the resisting force arising between two surfaces (of the same or different materials) that slide each over. Important for materials used as films or sheets on automatic packaging and printing machinery. Factors affecting: – Natural roughness of superficial layers. – Surface treatments. – Processing additives, e.g. lubricants and plasticizers, esp. for plastics. Desirable or non-desirable. – Desirable, e.g. when several packages are required to stacked one over another. – Non-desirable e.g. when several glass bottles have to run quickly close each other along a filling line. Mechanical properties – Coefficient of friction Schematic apparatus for determining coefficient of friction Static coefficient of friction: – The resistance opposing the force required to start the movement of one surface over the other. Dynamic or kinetic COF: – The resistance which opposes the force to move one surface at a fixed speed. Mechanical properties – Coefficient of friction Coefficient of friction (K = kinetic, S= static) of some packaging materials Material Coefficient of friction Cellophane on itself (K; kinetic) 4.50 Aluminum on itself (S; static) 1.90 Glass on glass (S) 0.9-1.0 Oriented polypropylene on itself (K) 0.94 Glass on glass lubricated (S) 0.30-0.60 Steel on steel (S) 0.58 Polystyrene on polystyrene (S) 0.50 Paper kraft 67 µm, on itself (K) 0.30 PVC on itself (K) 0.27 LDPE on itself (S) 0.20 Wood on wood (S) 0.20 Polyester on polyester (K) 0.45-0.38 Mechanical properties – Strength properties Stress The load divided by the area corresponding to its action against the body. A force per unit area (N mm-2 or MPa). Strain The measurement of deformation relative to a reference configuration of length, area, or volume (non-dimensional). The change in size or shape of a body in response to a stress applied. Brittleness, elasticity, tensile strength and toughness related to physical durability or resistance of the packaging materials. – Brittleness: the inability to experience a significant amount of strain without rupture; the attitude of breaking in the elastic field. – Elasticity: a reversible stress/strain behavior. – Tensile strength: the maximum tensile stress that a material can sustain. – Toughness: the energy that a material can absorb before rupturing; it is generally measured as the area under the stress strain curve. Mechanical properties – Strength properties Ductility and plasticity should be considered in the package manufacture, e.g. cold forming of metals or plastics – Ductility: the ability of a material to be plastically deformed by elongation without rupture. – Plasticity: the ability to be molded and retain a shape for a significant period under finite forces. Creep phenomenon is observed in the pressurized package, e.g. carbonated drinks. – Creep: the slow deformation measured under constant stress. Relaxation of stress needs to be considered in selection of proper material for stretch wrapping. – Relaxation: the attitude to experience, under a constant deformation, a reduction in stress required to sustain that deformation. Mechanical properties – Strength properties To describe the behavior of a solid material responding to an applied force, it is necessary to classify type, origin, and direction of the force itself. – Types of force applied: Static force: acts for length of time in a constant intensity, e.g. the load due to stacked packages on a shelf or a truck, the stress of a stretch wrapping. Dynamic force: quick in action and vary in intensity, e.g. impacts, falls, vibrations. – Origin of force: Internal to the package, e.g. overpressure of a carbonated drink, pressure changes during pasteurization and sterilization. External to the package, e.g. over-imposed stacking, knocks, impact. – Direction of force: Tensile stresses, e.g. the stretching force applied to wrap a food by a transparent thin film. Compressive stresses, e.g. the crushing forces exerted by industrial equipments to reduce the volume of plastic objects for waste disposal. In real conditions (packaging, handling, transport, storage, etc.), all the possible combinations of type, origin, and direction of forces are likely to be imposed. Mechanical properties – Strength properties: Tensile strength Tensile test: – A straight rectangular strip is generally used for thin films, while a dog-bone shape is requested for thicker samples – Specimen is stretched with a constant and low rate between a fixed jaw and a moveable head – Changes in length (strain) are monitored with the corresponding stress. Tensile test of a material specimen. A typical sample may be 150 mm long and 20 mm wide, with the center section 10 mm wide and 60 mm long. Mechanical properties – Strength properties: Tensile strength The main parameters obtainable from a typical Stress = σ = F / A ‘stress-strain’ curve in a tensile test – Unit: Mpa (N mm-2) Strain = ε = (L – Lo)/ Lo = ∆L / Lo – Lo: original length of the sample; L: the length after deformation. The area under the first linear tract: elastic region – The material behaves elastically – Deformation is reversible when the load is released, the sample will recover its original undeformed configuration, i.e. its original length. The area under the remaining part of the plot up to the sample break: plastic region. – Any increases in stress will lead to permanent deformation. – Deformation will be relatively large for small, almost negligible increases in stress. Tensile strength The maximum stress a material able to sustain before failure. For plastic films: the maximum y-value on the stress- strain plot before breakage. Elastic modulus (Young’s modulus) (MPa): The slope of the first rising and linear part of the stress-strain plot. Ratio of stress to strain. The higher the modulus, the higher its stiffness. Area under the curve (MPa or J) The energy required to break the sample of material. Related to the toughness of plastic polymers. The corresponding value on the x-axis is the elongation that a material can sustain without rupture. Strength values of some packaging materials in tensile test Material Modulus of elasticity Breaking point (MPa) (MPa) Aluminum 70000 70-210 Tinplate 1800000 330-740 Glass 70000 70 Cellulosic (kraft paper) - 25-100 Polypropylene 2000-3500 35-50 Polyester 4000-5000 170-270 Mechanical properties – Strength properties: Tear strength The resistance of packaging materials to tearing forces. Important, esp. for flat materials. For cellulosic and plastic packaging materials. The tear strength of the material is reduced if a notch in its edge or any other physical defect (micro- holes, micro-bubbles, sudden thickness changes, corners, cracks, etc) is present they act as stress concentrators or amplifiers may produce local stress even above the material maximum resistance. – The smaller the notch’s radius, the greater is the concentration factor. Mechanical properties – Strength properties: Burst strength Resistance to bursting: the pressure value required to obtain the rupture of the material in standard conditions. Pressure is applied at a controlled, slowly increasing rate, and it can be provided by water or compressed air according to specimen under examination. Common test for paper, board, plastic films, plastic sheets, glass bottles. Measurement is useful for predicting possible performance during the real usage of that item as well as for highlighting hidden or nonvisible defects in the structure. Mechanical properties – Strength properties: Creep resistance Creep: the slow deformation measured over time under constant stress. – When packages stacked on a pallet in the warehouse or on a shelf (creep by external forces). – For containers of pressurized beverage (creep by internal forces). – Heat processing also increases the internal pressure of food containers. Time dependent related to the commercial life of the package and/or the packaged product. Mechanical properties –Response to dynamic stresses Stresses that are variable in intensity and can be of very short duration: Impacts, falls, vibrations likely to occur during production and distribution lines. It is necessary to provide adequate protection against possible shocks and vibrations by choosing the reliable form of packaging. Related with external and internal fragility. Mechanical properties –Response to dynamic stresses Impact resistance – The severe shocks resulting from packages falling onto rigid surfaces or knocking against hard objects. – The resistance against these stresses = resilience = the capacity to absorb energy in impulsive form (shock) OR the capacity of a material to absorb energy when it is deformed elastically and then to return this energy with removal of the load. – Can be measured as the area under the strain-stress curve in the elastic region. – Resilience vs. fragility Mechanical properties –Response to dynamic stresses Cushioning properties – If a packaging material has to provide the food with protection against dynamic impacts or falls, it must not only resist the stress applied (it should not break), but it must also be able to absorb enough energy of the dynamic stress to reduce the stress amount reaching the food product. – How: By reducing the force per unit area, spreading them over the maximum possible surface and avoiding concentrated load on a single part (however, it can not protect against internal fragility). By sustaining the product at the strongest part in order to concentrate the stress on the more resistant point practically impossible for foods & does not reduce internal fragility. By extending the time of arresting or extending the distance of arresting (the length of space from the beginning of the contact to the end of movement) cushion material. – Designing cushion packaging for a delicate items needs: Identification of the product’s fragility. Identification of the dynamic cushioning property of the packaging material.