Blood Pressure PDF
Document Details
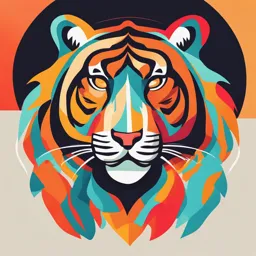
Uploaded by PropitiousSchrodinger830
Tags
Summary
This document provides a detailed explanation of blood pressure. It covers definitions, types of blood pressure (systolic and diastolic), and factors affecting blood pressure such as cardiac output and peripheral resistance. It also touches on the regulation of blood pressure.
Full Transcript
BLOOD PRESSURE Definitions (terminology) Blood Pressure Blood pressure is the lateral pressure exerted by the flowing blood on the walls of the vessels. It is usually measured in mmHg. Without any further qualification, the term blood pressure denotes the arterial pressure. While describing the p...
BLOOD PRESSURE Definitions (terminology) Blood Pressure Blood pressure is the lateral pressure exerted by the flowing blood on the walls of the vessels. It is usually measured in mmHg. Without any further qualification, the term blood pressure denotes the arterial pressure. While describing the pressure exerted by the blood column in other types of blood vessels, the type of vessels is also mentioned, e.g. capillary pressure and venous pressure. Systolic Blood Pressure The arterial pressure varies with phases of cardiac cycle. The maximum arterial pressure during systole is called systolic blood pressure, and occurs during ventricular ejection. The systolic blood pressure is a function of the cardiac output (CO), i.e. it represents the extent of work done by the heart. Normal systolic blood pressure in a young adult is 120 mmHg (range: 105–135 mmHg). Systolic blood pressure undergoes considerable fluctuations, e.g. it is increased during excitement, exercise and meals, and is decreased during sleep and rest. Diastolic Blood Pressure Diastolic blood pressure refers to the minimum arterial pressure during diastole and occurs just before the onset of ventricular ejection. Normal diastolic blood pressure in a young adult is 80 mmHg (range 60–90 mmHg). The diastolic pressure is the function of total peripheral resistance (TPR) and indicates the constant load against which the heart has to work. It undergoes much less fluctuations. Conventional Expression of Blood Pressure Conventionally, systolic and diastolic blood pressures are denoted as numerator and denominator, respectively. For example, blood pressure of a normal person is written as 120/80 mmHg. Pulse Pressure Pulse pressure is the arithmetic difference between systolic and diastolic blood pressures. Normally, average pulse pressure is 40 mmHg. It determines the pulse volume. It depends upon three factors: arterial volume, stroke volume and arterial elastic constant. Thus, according to Young’s elastic modules, the pulse 1 ΔV is arterial uptake volume (approximately equal to but not less than the stroke volume), V is arterial volume (proportional to the mean arterial pressure) and Ka is arterial elastic constant. The high pulse pressure is indicative of systolic hypertension and indirectly determines decrease in elasticity of blood vessels. Mean Arterial Pressure Mean arterial pressure (MAP) is the average of all pressure measured millisecond by millisecond throughout the cardiac cycle. Practically, MAP is roughly equal to diastolic pressure (DP) plus one-third of pulse pressure (PP). Normal value of MAP is 93 mmHg (range: 90–100 mmHg). Determinants of Arterial Blood Pressure The arterial blood pressure (BP) is a function of the product of cardiac output (CO) and total peripheral resistance (PR), i.e. Therefore, arterial blood pressure is affected by conditions that affect either cardiac output or peripheral resistance. Changes in cardiac output affect the systolic pressure more than diastolic pressure, while changes in peripheral resistance affect diastolic pressure more than the systolic pressure. The cardiac output is a function of heart rate and stroke volume, so these two are important determinants of the blood pressure. The peripheral resistance depends upon the viscosity of blood, elasticity of the vessel wall and velocity of the blood flow. Thus, these factors are also important determinants of the blood pressure. 1. Cardiac Output Systolic pressure is directly proportional to cardiac output. Whenever the cardiac output increases, the systolic pressure is increased and when cardiac output is less, the systolic pressure is reduced. Cardiac output increases in muscular exercise, emotional conditions, etc. So in these conditions, the systolic pressure is increased. In conditions like myocardial infarction, the cardiac output decreases, resulting in fall in systolic pressure. 2. Heart rate. An increase in heart rate usually increases the cardiac output and decreases the duration of cardiac cycle and thus raises the blood pressure. The increase in cardiac output due to increase in 2 heart rate increases diastolic pressure more than the systolic pressure (because increased heart rate duration of diastole decreases more than systole and the tendency of fall in pressure during diastole decreases). Conversely, reduction in heart rate decreases the arterial blood pressure. 3. Stroke volume. An increase in stroke volume increases the cardiac output and raises the arterial pressure, and the reverse effect occurs due to decrease in the stroke volume. When increase in cardiac output is primarily due to increase in stroke volume, it mainly increases systolic blood pressure. While an increase in cardiac output due to increase in both the stroke volume and heart rate increases systolic as well as diastolic pressure. 4. Peripheral resistance (Pr). Peripheral resistance is an important determinant of arterial pressure. Peripheral resistance is the resistance offered to the blood flow at the periphery. Resistance is offered at arterioles, which are called the resistant vessels. When peripheral resistance increases, diastolic pressure is increased and when peripheral resistance decreases, the diastolic pressure is decreased. Peripheral resistance in turn is determined by radius of vessels (arterioles), velocity of blood flow and viscosity of blood. 5. Elasticity of Blood Vessels Blood pressure is inversely proportional to the elasticity of blood vessels. Due to elastic property, the blood vessels are distensible and are able to maintain the pressure. When the elastic property is lost, the blood vessels become rigid (arteriosclerosis) and pressure increases as in old age. Deposition of cholesterol, fatty acids and calcium ions produce rigidity of blood vessels and atherosclerosis, leading to increased blood pressure. An increase in arterial elastic constant (or loss of elasticity of vessel wall) with advancing age results in decreased stretching of the elastic vessels during systole. This results in increased pressure during systole (systolic hypertension) with normal diastolic blood pressure. It is characterized by high pulse pressure. When stiffness occurs in small vessels, the diastolic blood pressure is also increased. 6. Viscosity of Blood Arterial blood pressure is directly proportional to the viscosity of blood. When viscosity of blood increases, the frictional resistance is increased and this increases the pressure. 3 7. Velocity of Blood Flow Pressure in a blood vessel is directly proportional to the velocity of blood flow. If the velocity of blood flow increases, the resistance is increased. So, the pressure is increased. 8. Diameter of Blood Vessels Arterial blood pressure is inversely proportional to the diameter of blood vessel. If the diameter decreases, the peripheral resistance increases, leading to increase in the pressure. 9. Blood Volume Blood pressure is directly proportional to blood volume. Blood volume maintains the blood pressure through the venous return and cardiac output. If the blood volume increases, there is an increase in venous return and cardiac output, resulting in elevation of blood pressure Variations in Blood Pressure Physiological Factors Affecting Blood Pressure 1. Age. In healthy humans, both systolic and diastolic pressures rise with age. At birth, systolic blood pressure is 40 mmHg (range: 20–60 mmHg). It then rises rapidly up to 1 month of age. At 1 month of age, the systolic blood pressure becomes about 80 mmHg and then rises slowly. At about 17 years of age, normal adult level of blood pressure 120/80 mmHg is reached, which remains almost constant up to 40 years of age and then very slowly rises. At about 70 years of age, the normal value of blood pressure is 160/90 mmHg. The increase in blood pressure associated with advancing age is due to increase in rigidity of vessel wall owing to arteriosclerotic changes. A convenient empirical formula as to what should be considered as normal blood pressure at various ages (after 10 years of age) is: - Systolic blood pressure: 110 + 3/5 of age in years. - Diastolic blood pressure: 70 + 2/5 of age in years. 4 2. Sex. Before menopause, females have little lower (4–6 mmHg) systolic blood pressure than males of corresponding age. This may be because of effect of oestrogen, while after menopause, systolic blood pressure in females is little higher (4–6 mmHg) than males of same age group. 3. Effect of Meals Systolic blood pressure increases by 4–6 mmHg after meals and this effect lasts for about 1 h. This occurs due to two reasons: Increase in heart rate due to pressure over the heart by distended abdomen and Increased epinephrine release from adrenal medulla. Diastolic blood pressure either remains unchanged or decreases slightly due to vasodilation in splanchnic vessels. 4. Emotions Increased sympathetic activity during emotional situations such as excitement, fear and worry leads to increase in systolic blood pressure due to increase in the cardiac output. 5. Climatic Temperature Exposure to cold produces rise in the blood pressure by increase in peripheral resistance due to cutaneous vasoconstriction through hypothalamic stimulation. Conversely, exposure to hot temperature lowers the blood pressure by decreasing peripheral resistance due to cutaneous vasodilation, again through hypothalamic pathway. 6. Diurnal Variation Systolic blood pressure shows a diurnal variation of about 6–10 mmHg, the values being lower in the morning and higher in the afternoon. In night workers, however, a reverse rhythm is observed. 7. Exercise In muscular exercise, generally systolic blood pressure rises and diastolic blood pressure falls. However, the effect of exercise is different in trained individuals (regular exerciser) than the untrained individuals (occasional exerciser). Further, the effect also varies depending upon the severity of exercise, whether mild, moderate or severe. However, blood pressure returns to normal levels within 5 min of stoppage of exercise due to sudden relaxation of muscles which produces vasodilation. 5 8. Effect of Gravity In standing position, due to hydrostatic (gravitational) effect of the blood column, the pressure in the vessels below heart level is increased and in the vessels above heart level, it is decreased. For every centimetre below or above the heart level, the pressure increases or decreases by 0.77 mmHg, respectively. Therefore, in an individual having 120/80 mmHg blood pressure in brachial artery, the blood pressure in the dorsalis pedis artery (which is about 100 cm below heart level) will be about 200/160 mmHg, i.e. about 80 mmHg more (0.77 × 100 = 77 mmHg = about 80 mmHg). Therefore, for clinical recording, blood pressure should always be checked at the heart level. 9. Effect of Change in Posture. Sudden change in posture from lying down to standing initiates some momentary changes in blood pressure which in normal humans are immediately rectified by baroreceptor reflexes, and practically such changes are not experienced. However, in patients with autonomic disturbances, these changes become symptomatic. A chain of physiological changes in blood pressure during change of posture is: Immediately on standing, there occurs peripheral pooling of blood in dependent parts leading to decreased venous return and decreased cardiac output, and momentary fall in systolic blood pressure. Fall in systolic pressure immediately decreases baroreceptor discharge via vasomotor centre leading to increased diastolic blood pressure. On standing, there also occurs an increase in peripheral resistance and momentary increase in diastolic blood pressure. Thus, immediately after standing from lying down posture, a rise in diastolic blood pressure can be recorded for about 30–60 s. Later on, due to decrease in baroreceptor discharge, blood pressure comes back to normal and no symptoms are experienced by the normal individuals. 10. Sleep. In complete relaxed state, during early hours of sleep, there occurs general vasodilation leading to fall in blood pressure up to 15–20 mmHg. However, in disturbed sleep, blood pressure increases due to increased sympathetic discharge. 11. Body Build Systolic blood pressure is slightly higher in obese individuals compared with thin-built individuals. Further, falsely higher values are obtained in obese individuals while testing brachial artery blood pressure using standard arm cuff. This occurs because there is more tissue between the cuff and artery and so, some of the cuff pressure is dissipated. Therefore, use of a cuff that is wider than the standard arm cuff is recommended for accurate record of blood pressure in obese individuals. 6 REGULATION OF BLOOD PRESSURE Arterial blood pressure is controlled by several mechanisms which under physiological conditions maintain the normal mean arterial pressure (MAP) within a narrow range of 95–100 mmHg. Each mechanism performs a specific function. Various mechanisms controlling arterial pressure can be grouped as: A. Rapid blood pressure control mechanism, B. Intermediate blood pressure control mechanisms and C. Long-term blood pressure control mechanisms. Rapid Blood Pressure Control Mechanism (Nervous Regulating Mechanism) Rapid blood pressure control mechanism or the so-called short-term control mechanism primarily includes the following three nervous reflexes: 1. Baroreceptor reflexes 2. Central nervous system ischaemic response and 3. Chemoreceptor reflexes Features Their salient features are: These act very rapidly, i.e. within seconds to few minutes of alterations in the blood pressure. These are short-term mechanisms, i.e. these act for few hours to few days and are thus insignificant in long-term regulation of blood pressure. These are useful in preventing acute decreases in blood pressure (e.g. during severe haemorrhage) as well as in preventing excessive increases in blood pressure (e.g. as might occur in response to excessive blood transfusion). A. Baroreceptor reflex This includes fast, neural mechanisms. It is a negative feedback system that is responsible for the minute-to-minute regulation of arterial blood pressure. Baroreceptors are stretch receptors located within the walls of the carotid sinus near the bifurcation of the common carotid arteries. 7 Steps in the Baroreceptor Reflex a. A decrease in arterial pressure decreases stretch on the walls of the carotid sinus. Because the baroreceptors are most sensitive to changes in arterial pressure, rapidly decreasing arterial pressure produces the greatest response. Additional baroreceptors in the aortic arch respond to increases, but not to decreases, in arterial pressure. b. Decreased stretch decreases the firing rate of the carotid sinus nerve [Hering’s nerve, cranial nerve (CN) IX], which carries information to the vasomotor center in the brain stem. c. The set point for mean arterial pressure in the vasomotor center is about 100 mm Hg. Therefore, if mean arterial pressure is less than 100 mm Hg, a series of autonomic responses is coordinated by the vasomotor center. These changes will attempt to increase blood pressure toward normal. d. The responses of the vasomotor center to a decrease in mean arterial blood pressure are coordinated to increase the arterial pressure to 100 mm Hg. The responses are decreased parasympathetic (vagal) outflow to the heart and increased sympathetic outflow to the heart and blood vessels. The following four effects attempt to increase the arterial pressure to normal: (1) ↑ heart rate, resulting from decreased parasympathetic tone and increased sympathetic tone to the SA node of the heart. (2) ↑ contractility and stroke volume, resulting from increased sympathetic tone to the heart. Together with the increase in heart rate, the increases in contractility and stroke volume produce an increase in cardiac output that increases arterial pressure. (3) ↑ vasoconstriction of arterioles, resulting from the increased sympathetic outflow. As a result, TPR and arterial pressure will increase. (4) ↑ vasoconstriction of veins (venoconstriction), resulting from the increased sympathetic outflow. Constriction of the veins causes a decrease in unstressed volume and an increase in venous return to the heart. The increase in venous return causes an increase in cardiac output by the Frank–Starling mechanism. Example of the baroreceptor reflex: response to acute blood loss Central Nervous System Ischaemic Response When the blood pressure drops to dangerously low levels (below 50 mmHg), blood flow to the VMC becomes inadequate to sustain the metabolism of its neurons. The resultant hypoxia then stimulates the neurons of the VMC directly and very strongly. This results in a powerful 8 sympathetic vasoconstriction capable of increasing the blood pressure to 270 mmHg for about 10 minutes. Maximum stimulation occurs when the blood pressure falls to about 15 mmHg. If the response fails to relieve the ischaemia of the VMC neurons within 3 minutesm the nerons begin to die. Chemoreceptor Reflexes When the blood pressure falls to levels below 60 mmHg (levels at which baroreceptors are inactive), the blood flow through the tissues is slowed down causing hypoxia, hypercapnea and acidosis. These chemical changes stimulate the chemoreceptors in the carotid bodies and the medulla so that there is an increase in respiration and stimulation of VMC inorder to increase the blood pressure and flow to tissues. Emergencies such as massive hemorrhage activate the chemoreceptors. Their role is limited by the fact that the direct effects of hypoxia, hypercapnea and acidosis on blood vessels are vasodilation, which antagonizes their reflex action. Intermediate Blood Pressure Control Mechanisms The intermediate blood pressure control mechanisms that are important in blood pressure control after several minutes of acute pressure changes are: 1. Renin–angiotensin vasoconstrictor mechanism 2. Capillary fluid shift mechanism. Features of intermediate blood pressure control mechanisms are: These mechanisms come into play after several minutes of acute pressure changes and reach full function within a few hours. These mechanisms play their role from few days to few weeks. 1. Renin–Angiotensin Vasoconstrictor Mechanism The renin–angiotensin system has important roles in the regulation of blood pressure and in the regulation of extracellular fluid volume. Renin Secretion and Angiotensin Formation Renin, a protease enzyme is secreted by juxtaglomerular cells of the kidney into the blood. Its secretion is stimulated by a decrease in the blood pressure. 9 Renin catalyzes the conversion of angiotensinogen (α2 – globulin substrate present in the plasma) to angiotensin I. Angiotensin I is converted into angotensin II by the action of angiotensin converting enzyme (ACE) present in the endothelium of blood vessels throughout the body, especially in the lungs and kidneys. Angiotensin II persists in the blood until it is rapidly inactivated by multiple blood and tissue enzymes collectively called angiotensinases Effects of Angiotensin II Angiotensin II is the most potent pressor substance being 4 to 8 times more potent than norepinephrine. By producing generalized vasoconstriction of arterioles and veins throughout the body it raises both systolic and diastolic pressure. This effect of angiotensin II is important in intermediate blood pressure control during circumstances such as acute haemorrhage. Capillary Fluid Shift Mechanism Capillary fluid shift mechanism helps in restoring both low and high blood pressure back to normal: When blood pressure is raised, the mean capillary pressure is also high, resulting in shift of fluid from circulation to the interstitial fluid compartments. This reduces the blood volume to restore the arterial Pressure. When blood pressure is lowered, the mean capillary pressure is also low, resulting in absorption of fluid from the interstitial compartments to circulation. Thus, the blood volume is increased which helps to return the blood pressure back to normal. The capillary fluid shift mechanism is about two times more effective than baroreceptor reflex mechanism in controlling the blood pressure, but it acts much more slowly (intermediate-acting mechanism) than baroreceptor mechanism (rapid-acting mechanism). Long-Term Blood Pressure Control Mechanisms Kidneys play main role in the long-term control of blood pressure by the following mechanisms: 1. Direct mechanism, i.e. ‘renal body fluid feedback mechanism’. 2. Indirect mechanisms control kidney functions indirectly via following hormonal mechanisms: i. Aldosterone system and ii. Renin–angiotensin system 10 Renal–Body Fluid System for Arterial Pressure Control The most important mechanism for the long-term control of blood pressure is linked to control of circulatory volume by the kidney, a mechanism known as the renal–body fluid feedback system. In fact, it is similar to the capillary fluid shift mechanism except that only the renal glomerular capillaries are involved in the process. Modes of Operation of Renal–Body Fluid Feedback System The renal–body fluid system corrects blood pressure by causing appropriate changes in blood volume through diuresis and natriuresis: When blood pressure rises too high, the kidneys excrete increased quantities of sodium and water because of pressure natriuresis and pressure diuresis, respectively. As a result of increased renal excretion, the extracellular fluid volume and blood volume both decrease until blood pressure returns to normal and the kidneys excrete normal amounts of sodium and water. When the blood pressure falls too low, the kidneys reduce the rate of sodium and water excretion, and over a period of hours to days, if the person drinks enough water and eats enough salt to increase blood volume, the blood pressure will return to its previous level. This mechanism being very slow to act is not of major importance in the acute control of arterial pressure. However, it is by far the most potent of all long-term arterial pressure controllers. The sequence of events in order of occurrence during control of blood pressure by this mechanism is summarized as: 11 Salient features of renal–body fluid feedback mechanism. The salient features of the renal–body fluid feedback mechanism can be summarized as: The renal–body fluid feedback mechanism takes several hours to show any significant response. These mechanisms operate very powerfully to control arterial pressure over days, weeks and months. The effectiveness of these mechanisms becomes steadily greater with time. These mechanisms, if given sufficient time, control arterial pressure at the level that provides normal output of salt and water by the kidneys. 12 As long as kidney function is unaltered, these mechanisms overcome the disturbances that tend to alter arterial pressure such as increased total peripheral resistance over a long period and thus are able to control the blood pressure. B. Renin–angiotensin–aldosterone system This is a slow, hormonal mechanism and is used in long-term blood pressure regulation by adjustment of blood volume. Steps in the Renin–Angiotensin–Aldosterone System a. A decrease in renal perfusion pressure causes the juxtaglomerular cells of the afferent arteriole to secrete renin. b. Renin is an enzyme that catalyzes the conversion of angiotensinogen to angiotensin I in plasma. c. Angiotensin-converting enzyme (ACE) catalyzes the conversion of angiotensin I to angiotensin II, primarily in the lungs. ACE inhibitors (e.g., captopril) block the conversion of angiotensin I to angiotensin II and, therefore, decrease blood pressure. Angiotensin receptor (AT1) antagonists (e.g., losartan) block the action of angiotensin II at its receptor and decrease blood pressure. d. Angiotensin II has four effects: (1) It stimulates the synthesis and secretion of aldosterone by the adrenal cortex. Aldosterone increases Na+ reabsorption by the renal distal tubule, thereby increasing extracellular fluid (ECF) volume, blood volume, and arterial pressure. This action of aldosterone is slow because it requires new protein synthesis. (2) It increases Na+–H+ exchange in the proximal convoluted tubule. This action of angiotensin II directly increases Na+ reabsorption, complementing the indirect stimulation of Na+ reabsorption via aldosterone. This action of angiotensin II leads to contraction alkalosis. (3) It increases thirst. (4) It causes vasoconstriction of the arterioles, thereby increasing TPR and arterial pressure 13 Conclusion To conclude, it can be stated that rapid control of arterial pressure begins with life-saving measures of the nervous reflexes, continues with sustaining characteristics of the intermediate pressure controls and finally is stabilized at the long-term pressure level by the renal–body fluid feedback mechanism. APPLIED PHYSIOLOGY Pathological variations of arterial blood pressure: A. Hypertension B. Hypotension. HYPERTENSION Definition Hypertension is defined as the persistent high blood pressure. Clinically, when the systolic pressure remains elevated above 150 mm Hg and diastolic pressure remains elevated above 90 mm Hg, it is considered as hypertension. If there is increase only in systolic pressure, it is called systolic hypertension. Types of Hypertension Hypertension is divided into two types: 1. Primary hypertension or essential hypertension 2. Secondary hypertension. 1. Primary Hypertension or Essential Hypertension Primary hypertension is the elevated blood pressure in the absence of any underlying disease. It is also called essential hypertension. Arterial blood pressure is increased because of increased peripheral resistance, which occurs due to some unknown cause. Primary hypertension is of two types: i. Benign hypertension ii. Malignant hypertension. 14 i. Benign hypertension Benign hypertension is the high blood pressure that does not cause any problem. It is defined as the essential hypertension that runs a relatively long and symptomless course. In early stages of this condition, there is moderate increase in blood pressure, with systolic pressure of 200 mm Hg and the diastolic pressure of about 100 mm Hg. However, in resting conditions and sleep, the blood pressure returns to normal level. Later, there is a further increase in blood pressure and it does not come back to normal level in resting conditions. Persistent increase in pressure over the years causes development of vascular, cardiac or renal diseases. ii. Malignant hypertension Malignant hypertension is a severe form of hypertension with a rapid course leading to progressive cardiac and renal diseases. It is also called accelerated hypertension. In this case, the blood pressure is elevated to a great extent. Systolic pressure rises to about 250 mm Hg and diastolic pressure rises to 150 mm Hg. It is always developed due to the combined effects of primary and secondary hypertension. Malignant hypertension cause severe damage of tunica intima of small blood vessels and organs like eye (retina), heart, brain and kidneys. It is a fatal disease, since it causes death within few years. 2. Secondary Hypertension Secondary hypertension is the high blood pressure due to some underlying disorders. The different forms of secondary hypertension are: i. Cardiovascular hypertension Cardiovascular hypertension is produced due to the cardiovascular disorders such as: a. Atherosclerosis: Hardening of blood vessels due to fat deposition b. Coarctation of aorta: Narrowing of aorta. ii. Endocrine hypertension Endocrine hypertension is developed because of hyperactivity of some endocrine glands: a. Pheochromocytoma: Tumor in adrenal medulla, resulting in excess secretion of catecholamines b. Hyperaldosteronism: Excess secretion of aldosterone from adrenal cortex c. Cushing syndrome: Excess secretion of glucocorticoids from adrenal cortex. 15 iii. Renal hypertension Renal diseases causing hypertension: a. Stenosis of renal arteries b. Tumor of juxtaglomerular cells, leading to excess production of angiotensin II c. Glomerulonephritis. iv. Neurogenic hypertension Nervous disorders producing hypertension: a. Increased intracranial pressure b. Lesion in tractus solitarius c. Sectioning of nerve fibers from carotid sinus. v. Hypertension during pregnancy Some pregnant women develop hypertension because of toxemia of pregnancy. Arterial blood pressure is elevated by the low glomerular filtration rate and retention of sodium and water. It may be because of some autoimmune processes during pregnancy or release of some vasoconstrictor agents from placenta or due to the excessive secretion of hormones causing rise in blood pressure. Hypertension is associated with convulsions in eclampsia. Manifestations of Hypertension Severe manifestations of primary hypertension: 1. Renal failure 2. Left ventricular failure 3. Myocardial infarction 4. Cerebral hemorrhage 5. Retinal hemorrhage. Treatment of Hypertension Secondary hypertension is cured by treating the disease causing hypertension. Primary hypertension can be controlled but cannot be cured. Following are the antihypertensive drugs to control primary hypertension: 16 1. Beta adrenoceptor blockers Beta adrenoceptor blockers or beta antagonists (adrenergic beta blockers or beta blockers) block the effect of sympathetic nerves on heart and blood vessels by binding with beta adrenoceptors, so that there is reduction in cardiac output and inhibition of vasoconstriction, leading to fall in blood pressure. 2. Alpha adrenoceptor blockers Alpha adrenoceptor blockers or alpha antagonists (adrenergic alpha blockers or alpha blockers) block the effect of sympathetic nerves on blood vessels by binding with alpha adrenoceptors, leading to vasodilatation and fall in blood pressure. 3. Calcium channel blockers Calcium channel blockers are drugs, which block the calcium channels in myocardium and thereby, reduce the contractility of myocardium. It causes decrease in cardiac output and fall in blood pressure. 4. Vasodilators Vasodilator agents reduce blood pressure by vasodilatation. 5. Diuretics Diuretics cause diuresis and reduce the ECF volume and blood volume. So, blood pressure is decreased. 6. Inhibitors of angiotensin-converting enzyme (ACE inhibitors) ACE inhibitors reduce the blood pressure by blocking the formation of angiotensin. 7. Depressors of vasomotor center Depressor drugs act on vasomotor center and reduce the vasomotor tone. So, vasoconstriction is prevented. 8. Angiotensin II receptor blockers Angiotensin II receptor blockers or antagonists are the antihypertensive drugs that decrease the blood pressure by blocking the effect of angiotensin II (vasoconstriction and secretion of aldosterone). 17 HYPOTENSION Definition Hypotension is the low blood pressure. When the systolic pressure is less than 90 mm Hg, it is considered as hypotension. Types 1. Primary hypotension 2. Secondary hypotension. 1. Primary hypotension Primary hypotension is the low blood pressure that develops in the absence of any underlying disease and develops due to some unknown cause. It is also called essential hypotension. Frequent fatigue and weakness are the common symptoms of this condition. However, the persons with primary hypotension are not easily susceptible to heart or renal disorders. 2. Secondary hypotension Secondary hypotension is the hypotension that occurs due to some underlying diseases. Diseases, which cause hypotension are: i. Myocardial infarction ii. Hypoactivity of pituitary gland iii. Hypoactivity of adrenal glands iv. Tuberculosis v. Nervous disorders. Orthostatic hypotension Orthostatic hypotension is the sudden fall in blood pressure while standing for some time. It is due to the effect of gravity. It develops in persons affected by myasthenia gravis or some nervous disorders like tabes dorsalis, syringomyelia and diabetic neuropathy. Common symptom of this condition is orthostatic syncope. 18