DNA Replication, Mutation, and Repair 2024 PDF
Document Details
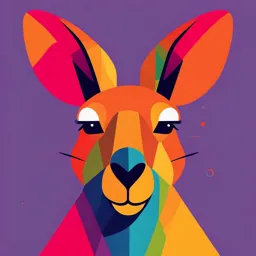
Uploaded by FascinatingDouglasFir7101
University of Debrecen Faculty of Medicine
2024
Scholtz Beáta
Tags
Related
- DNA Replication, Mutation & Repair Lecture Notes PDF
- Lecture 10 - DNA, Replication, and Mutation PDF
- Lecture 10 - DNA, Replication, and Mutation PDF
- Lecture 14 Mutation & Repair II Fall 2024 BIOL 23373 PDF
- Concepts to Refresh-Biochem PDF
- Harper's Biochemistry Chapter 35 PDF - DNA Organization, Replication, & Repair
Summary
This document is a lecture on DNA replication, mutation, and repair, suitable for an undergraduate or graduate level biology course. The document covers topics such as replication errors, types of mutations, DNA repair mechanisms, and the effects of mutations on the organism. The lecture was given in 2024.
Full Transcript
DNA REPLICATION, MUTATION AND REPAIR 2024 Scholtz Beáta E-mail: [email protected] MAIN POINTS 1. DNA replication and the cell cycle 2. Events and enzymes of DNA replication: initiation, DNA polymerases, telomerase complex 3. T...
DNA REPLICATION, MUTATION AND REPAIR 2024 Scholtz Beáta E-mail: [email protected] MAIN POINTS 1. DNA replication and the cell cycle 2. Events and enzymes of DNA replication: initiation, DNA polymerases, telomerase complex 3. Types and causes of mutations 4. Effects of mutations in the cell and in the organism 5. DNA repair systems DNA REPLICATION: DUPLICATION OF THE CELL'S DNA The chromosomal DNA should be duplicated before cell division, so that both daughter cells will have their own complete genomes. Continuously dividing (i.e., proliferative) cell populations: in mucosal membranes, skin, bone marrow, reproductive organs, etc. Most of our cells are not proliferative, so they don't need to replicate their chromosomal DNA. Replication of the mitochondrial genome is regulated differently, not coupled to cell division. Replication of the mitochondrial genome is activated mostly by growing cell size, or by increased energy requirements. DNA REPLICATION OCCURS IN THE S PHASE OF THE CELL CYCLE Continuously dividing somatic cells go through interphase, followed by mitotic (M) phase repeatedly – the two phases form the cell cycle. Interphase also has three sub-phases: G1, S and G2 phases M phase has two sub-phases: mitosis, when dissolution of the cell nucleus and division of the chromosomes occurs; followed by cell division (cytokinesis). DNA REPLICATION IS SEMICONSERVATIVE: ONE STRAND OF THE NEW DOUBLE HELICES IS DERIVED FROM THE ORIGINAL DNA, AND THE OTHER STRAND IS NEWLY SYNTHESIZED Separation of the two DNA strands is followed by their duplication. Replication fork: This is where the two The original DNA strands are the strands of the original DNA start to separate template strands (black). Both original strands serve as templates for the synthesis of the new, complementer strands (red). Two new DNA double helices PHASES OF DNA REPLICATION 1. Initiation phase: separation of the DNA strands occurs at certain chromosomal positions, and this is where DNA synthesis begins 2. DNA synthesis, copying of the template strands 3. Termination, completion of chromosomal ends (Replication of the mitochondrial genome will not be discussed) INITIATION OF DNA REPLICATION Cells establish the future replication origins on the chromosomes early on after cytokinesis, at DNA the beginning of the G1 phase. Multiple proteins form a complex at the origin of replication – ORC (origin recognition complex). The active pre-initiation complex (Pre-IC) forms ATP hydrolysis later, at the beginning of the S phase – the binding of the helicase complex helicase activity of this complex is essential to protein phosphorylation unwind the DNA double helix, and to begin binding of other proteins the replication. The human genome contains over 300 000 replication origins – the large genome size requires multiple initiation sites to finish DNA replication in time. INITIATION OF DNA REPLICATION: THE DETAILS The ORC (origin recognition complex) is a complex of 6 proteins: ORC1-6, produced DNA from 6 different genes. All other proteins required for the active replication complex are anchored through the ORC. The position Cdc6 and Cdt1 attach, and sequence of eukaryotic replication release of energy origins is variable, and difficult to identify Cdc6 experimentally. Cdc6/Cdt1 binds to ORC at the G1/S phase boundary. Cdc6 hydrolyzes an ATP, and the energy is used for the binding of the 6- Cdc6 and Cdt1 depart, MCM2-7 complex subunit MCM2-7 helicase complex to the attaches ORC. At this point the Cdc6/Cdt1 proteins dissociate from the ORC. Binding of the MCM2-7 helicase complex results in the formation of the pre-replicative Extra material complex (Pre-RC). INITIATION OF DNA REPLICATION: THE DETAILS Pre-RC is activated at the beginning of the S phase, depending on the activation of S phase- specific cyclin-dependent protein kinases: CDK and DDK. CDK and DDK phosphorylate their target proteins in the Pre-RC (including some subunits of the MCM complex), promoting the binding of GINS and Cdc45 proteins. The pre-initiation complex (Pre-IC) is created: phosphorylated MCM2-7, GINS és Cdc45 together form the active replication helicase complex. Pre-IC is formed only once during the cell cycle on a replication origin, since an inhibitor protein (geminin) accumulates during S-G2-M phases and inhibits repeated activations. Extra material DNA IS SYNTHESIZED IN THE 5'-3' DIRECTION Template DNA DNA polymerase DNA is synthesized by DNA polymerase enzymes, moving from 5' to 3' direction. To start the synthesis, these enzymes require a short oligonucleotide binding to the DNA through complementary basepairing – this is the primer, which will be extended by the polymerases. The enzymes attach the next nucleotide to the free 3' OH group of the last nucleotide in the primer, copying the template DNA sequence. DNA POLYMERASES NEED A PRIMER TO START THE SYNTHESIS The primer is synthesized by the primase enzyme, which is an RNA polymerase. The primase synthesizes 7-12 nucleotide (nt) of RNA – thus, DNA replication requires an RNA oligonucleotide primer. Next, the RNA primer is extended by DNA polymerase a, but only by 20 nt DNA. From this point on DNA polymerase d and e takes over – these are the main replicative DNA polymerases. REPLICATION OF BOTH TEMPLATE DNA STRANDS BEGINS AT THE REPLICATION FORK In the DNA double helix the two strands are in Lagging strand antiparallel orientation – however, DNA polymerases can synthesize only in the 5'-3' direction. e d The leading strand is copied by DNA polymerase e in a Leading continuous fashion, in 5'-3' direction. strand The lagging strand is looped back to present itself to Primer DNA polymerases DNA polymerase d in the correct orientation – the two DNA polymerases (e and d) form a complex at the replication fork, and they need to move together during synthesis. After 150-200 bp the DNA polymerase d releases the template strand, and locates the next free primer end. Therefore, the lagging strand is copied in 150-200 bp fragments – these are the Okazaki fragments. This means that multiple primers are needed to copy Lagging strand copy the lagging strand, whereas a single primer is needed on the leading strand. LEADING STRAND, LAGGING STRAND AND OKAZAKI FRAGMENTS Lagging strand e d Leading strand Primer DNA polymerases Primers Leading strand Lagging strand Lagging strand copy Single stranded DNA binding proteins Lagging strand primer Pol a + primase Okazaki fragment DNA Helicase PCNA: supports the DNA polymerases, prevents premature Leading strand dissociation from the template DNA. ELECTRON MICROSCOPY IMAGE OF THE REPLICATION COMPLEX ssDNA binding proteins Leading strand dsDNA Lagging strand JOINING OF THE OKAZAKI FRAGMENTS DNA polymerase d reaches the RNA primer of the next Okazaki fragment. With the help of an attached helicase the polymerase peels off the primer + some of the DNA from the template, then fills in the gap (DNA synthesis). An endonuclease enzyme cleaves off the "hanging" single stranded DNA at the branch point. The RNA primer has been removed, but the two Okazaki fragments are not yet attached covalently. A DNA ligase joins the Okazaki fragments with the usual phosphodiester bond. DIFFERENT DNA POLYMERASES ARE REQUIRED FOR REPLICATION AND FOR DNA REPAIR DNA polymerases Functions DNA polymerase α, δ and ε Main replicative polymerases DNA polymerase γ Mitochondrial DNA replication DNA polymerase ζ Replication of damaged DNA DNA polymerase β Base excision DNA repair DNA polymerase λ and μ Repair of double stranded DNA breaks DNA polymerase η, ι and κ Damaged DNA repair (poor fidelity) Extra material CONFORMATIONAL CHANGE IN THE DNA STRUCTURE WITH UNWINDING OF THE DOUBLE HELIX: THE SUPERCOIL PROBLEM chromosome chromosome end, attached end, attached Replication complex rotation due to unwinding Direction of replication fork movement Supercoils The ends of the chromosomes (DNA double helix) are attached to the nuclear membrane or the nuclear matrix, and cannot rotate freely – there would not be enough space in the crowded nucleus, anyway. The mitochondrial genome is circular, also without free ends. Unwinding of the DNA during replication causes local rotation and formation of supercoils in front of the replication fork. These have to be resolved, or the replication stalls. Deewese JE et al. DOI 10.1093/nar/gkn937 TOPOISOMERASE ENZYMES: RESOLUTION OF SUPERCOILS Topoisomerase 1B functions by cutting one DNA strand, rotating the cleaved strand around the uncleaved strand, then ligating (covalently joining) the DNA strand ends using ATP. This eliminates the supercoils. Type 2 topoisomerase enzymes cleave both DNA strands to allow free rotation, then religate them. Single stranded DNA binding proteins Lagging strand primer Pol a + primase Topoisomerase Okazaki fragment DNA Helicase Leading strand TERMINATION OF REPLICATION Replication "bubbles" starting from neighboring origins reach each other. Leading strand of one replicon is ligated to the lagging strand of the neighboring replicon ("fusing") – both strands of the new DNA double helices become continuous. Replication of chromosome ends (telomers) requires an additional enzyme complex. THE LAGGING STRAND GETS SHORTER WITH EACH REPLICATION Original DNA double helix After replication Two new double helices An Okazaki fragment is missing Another round of (only at the lagging strand!) replication from the "lagging strand" DNA helix One of the new DNA double helices The DNA molecule gets shorter PREVENTING INFORMATION LOSS DUE TO CHROMOSOME SHORTENING: TELOMERE SEQUENCE AND TELOMERASE COMPLEX Telomerase enzyme Chromosome Telomer centromer RNA template other proteins The telomeric DNA is made of a short repetitive sequence: TTAGGG, The telomerase enzyme is a reverse in several hundred copies. This transcriptase: it copies RNA into DNA. minisatellite doesn't carry genetic The enzyme binds an RNA template, with information. The 3' end of the partially complementary sequence to the chromosome has a short overhang. telomeric repeat. PREVENTING INFORMATION LOSS DUE TO CHROMOSOME SHORTENING: TELOMERE SEQUENCE AND TELOMERASE COMPLEX RNA template of the telomerase hybridizes to the 3' overhang of the telomer. The telomerase enzyme copies the RNA template, extending the chromosomal DNA, making a longer 3' overhang. Translocation: the RNA template hybridizes to the new repeat, translocating in the 3' direction. The telomerase enzyme extends the chromosomal DNA again. This is repeated multiple times, so that finally the DNA polymerase a + primase can bind to the end, to synthesize the complement of the 3' overhang. THE TELOMERASE COMPLEX REMAINS ACTIVE ONLY IN SPECIALIZED CELLS AFTER BIRTH The activity of the telomerase complex is at the highest during early embryonic development, when almost all cells divide continuously. After birth the complex remains active in tissue stem cells, which are responsible for tissue regeneration, and in the poliferative cell populations of certain tissues: mucosal membranes, bone marrow, skin, etc. Reproductive organs also have active telomerase. Specific inhibitor proteins are also at work to limit the activity of the telomerase complex – ideally, chromosomal ends do not become shorter or longer with repeated replications. The system is not foolproof: ageing is associated with telomer shortening in some cells. Cancer cells can proliferate indefinitely only if they reactivate the telomerase complex – otherwise they die after a certain number of cell divisions, due to critical chromosomal shortening. AGEING AND TELOMERS Shortening telomers: more If the telomer becomes too short, the and more cells are affected telomerase complex cannot bind. with ageing. The free DNA ends are identified as damaged 💥 DNA by the cells. cell divisions Continuous "damaged DNA" signal stops the Telomerase complex cell cycle – this interferes with tissue regeneration. 💥 After this cells become senescent and die – however, this process causes local inflammation in the tissues. Dysfunctional tissue regeneration and inflammation causing tissue damage together contribute to organ dysfunction and degenerative diseases associated with ageing. Extra material DNA MUTATION AND DNA REPAIR Many thanks to Professor Virág for the lecture material! MUTATIONS AND DNA REPAIR What is a mutation? Causes of mutations: - Spontaneous (e.g. replication error) - Chemical agents - Physical agents Effects of mutations - beneficial (genetic variability, antibody repertoire) - pathological (diseases, cancer) DNA repair - Excision repair– BER and NER - Mismatch repair – repairing replication errors - Repairing single- or double-strand DNA breaks -... etc. Diseases associated with DNA repair dysfunction MUTATION A small alteration in the DNA sequence affecting a few nucleotides: it occurs randomly, as a result of environmental agents or replicative errors, mostly in somatic cells. The frequency of the mutant variant is low in the human population, below 1%. Mutations are sometimes necessary and natural in the body, such as the so-called hypermutation in the antibody-coding genes. This process contributes to the extreme variability of the amino acid sequence in the antibodies. CHROMOSOMES MAY UNDERGO LARGE-SIZED ALTERATIONS AS WELL Recombination: it has different types, including the exchange of DNA regions with homologous sequences between paternal and maternal chromosomes during meiosis. It can also be activated during DNA repair. Chromosomal aberrations: deletion, recombination, inversion, translocation, amplification of larger regions. Chromosome copy number changes. These are discussed in detail in Genetics. Chromosomal translocation example: The product of the BCR-ABL fusion gene is an oncogene, and causes leukemia. The aberrant chromosome 22 is called the Philadelphia chromosome. Extra material TYPES OF MUTATIONS Point mutation: Alteration of a single nucleotide transition: purine-purine, or pyrimidine-pyrimidine change. E.g. adenine changes to guanine, or cytosine to thymine. transversion: purine-pyrimidin, or pyrimidine-purine change. E.g. adenine changes to cytosine, or thymine to guanine. Insertion or deletion (indel): insertion or deletion of one or a few nucleotides. MUTATIONS AND DNA REPAIR What is a mutation? Causes of mutations: - Spontaneous (e.g. replication error) - Chemical agents - Physical agents Effects of mutations - beneficial (genetic variability, antibody repertoire) - pathological (diseases, cancer) DNA repair - Excision repair– BER and NER - Mismatch repair – repairing replication errors - Repairing single- or doubl-strand DNA breaks -... etc. Diseases associated with DNA repair dysfunction CAUSES OF MUTATIONS Replication error (spontaneous mutation) mismatch replication slippage, causing indel Chemical mutagens covalent modification of bases intercalating agents Physical mutagens ionizing radiation, UV, heat REPLICATION ERROR - SPONTANEOUS MUTATIONS The main replicative DNA polymerase d and e copy the DNA with extremely high fidelity. Mechanisms ensuring error-free replication: nucleotide selection error recognition and repair - proofreading Spontaneous errors do occur at a very low rate, caused by incorporation of the wrong nucleotide. This causes a mismatch, since the error is just on the new strand initially, causing an aberrant basepair. Example: C = A or G = T The DNA repair systems usually recognize and correct the mismatch very effectively. If not, a point mutation may develop, affecting both DNA strands. mismatch REPLICATION ERROR - MISMATCH AND REPAIR Replicated DNA, no error Unsuccessful repair: point mutation Replication error, Template DNA mismatch Replicated DNA, with error Successful repair NUCLEOTIDE SELECTION FOR HIGH FIDELITY REPLICATION G G T C G T T C C X X A A X A A Conformation of the DNA polymerase changes after binding the proper (complementary) nucleotide-triphosphate: it folds tightly around the future basepair, forming the active center of the enzyme (closed conformation). The enzyme can bind only dexoxyribonucleotides. If the enzyme binds a non-complementary nucleotide-triphosphate, the closed conformation cannot form properly, and the polymerase activity of the enzyme is inhibited. PROOFREADING FOR HIGH FIDELITY REPLICATION DNA polymerase d and e have an additional 3'-5' exonuclease domain, that can remove the non- complementary nucleotide if The last nucleotide in proper incorporated in error. basepairing: the polymerase activity is dominant Error recognition = incorrect basepair at the end, distorted structure. The polymerase slows down and stops. Error repair = the DNA polymerase now works as a 3'-5' exonuclease, and The last nucleotide not in proper removes the non-complementary basepairing: the end of the new nucelotide from the end. After this the strand translocates to the enzyme switches to polymerase mode exonuclease domain, and gets again, continuing the DNA synthesis. digested NOT ALL MISMATCHES ARE CAUSE BY POLYMERASE ERROR : tautomeric shift of the bases can also be blamed Example: Thymine may be in keto form (dominant), or can shift spontaneously to the enol form (rare), then shift back later. Enol- thymine basepairs with guanine. If a thymine happens to be in the enol form when the replication fork passes, then the DNA polymerase incorporates a guanine instead of adenine. When the enol-thymine shifts back spontaneously, this causes a mismatch. Similarly: guanine has keto and enol forms – enol-guanine basepairs with thymine. adenine has amino and imino forms – imino-adenine basepairs with cytosine. REPLICATION SLIPPAGE: MAY CAUSE INDEL MUTATIONS Replication slippage: The template strand and its copy slip relative to each other in the replication fork. Depending on the direction of slippage, part of the template sequence is copied twice, or not at all. Repetitive sequences are especially prone to replication slippage. Di- and trinucleotide microsatellites are copied with a high error rate by DNA polymerases. Microsatellite lengths (i.e. the number of repeats) are highly variable in the human population. REPLICATION SLIPPAGE Replicated DNA, no error Unsuccessful repair: + 1 CA dinucleotide, the repeat region became longer Replication slippage, Template DNA, CA insertion with dinucleotide (CA) repeat Replicated DNA with error Successful repair DISEASES CAUSED BY TRINUCLEOTIDE REPEAT EXPANSION >>> Extra material Extra material HUNTINGTON DISEASE Huntington disease is caused by genetically determined degeneration of the neurons in specific brain regions. The disease manifests with uncontrolled movements (chorea), cognitive decline, and emotional disturbances. The disease can be inherited. Usually manifests between the age of 30 to 50, but George Huntington: described the disease in 1872. age of onset and severity of symptoms vary depending on the number of repeats and other genetic factors. The mutant huntingtin protein causes neuronal death – although it is produced from birth, it takes decades for the affected brain regions to accumulate enough damage for the symptoms to appear. The affected brain regions CHEMICAL MUTAGENS Covalent modification of bases Intercalating agents COVALENT MODIFICATION OF BASES: DEAMINATING AGENTS Deamination of cytosine produces uracil – this pairs with adenine, resulting in point mutations (T-A instead of C-G). Deamination of adenine produces hypoxanthine – this pairs with cytosine, resulting in point mutations (G-C instead of A-T). Deamination of guanine produces xanthine – this inhibits the DNA polymerase (replication), but it's not mutagenic. COVALENT MODIFICATION OF BASES: ALKYLATING AGENTS Dimethyl nitrosamine EMS (ethylmethane sulfonate) Effect: depends on the position of the modification as well may change basepairing (point mutation see below) may block replication by crosslinking the two DNA strands large groups attached to bases may block the progress of the replication complex simply due to their size. COVALENT MODIFICATION OF BASES: OXIDATION, 8-OXOGUANINE Aberrant cellular Oxidative chemical Ionizing radiation processes, stress mutagens Oxidation 8-oxoguanine may basepair with adenine, causing point mutations. Reactive oxygen species/oxidative agents entering the cells or being produced within the cells may damage other bases as well, but guanine is the most sensitive, and 8-oxoguanine is the most frequent type of modification. CHEMICAL MUTAGENS: INTERCALATING AGENTS Intercalating agents contain 3-4 aromatic rings, forming a flattened structure. This structure enables them to fit easily between the planes of the neighboring basepairs in the DNA double helix. Intercalating agents inhibit replication, transcription and topoisomerases, and may cause insertion mutations. Their cytotoxic effects can be harnessed in medicine – some of them are used as antitumor agents. Extra material Natural intercalating agents, examples: berberin – present in barberry, but hardly absorbed from the GI tract. ellipticin – isolated from Ochrosia trees (a relative of leander). doxorubicin – isolated from a Streptomyces species in the 1950-s. Used in cancer therapy. doxorubicin PHYSICAL MUTAGENS UV, heat, ionizing radiation PHYSICAL MUTAGENS: UV UV light induces dimerization of neighboring pyrimidine bases (among other photoproducts). Thymines are especially sensitive, and thymine dimerization can cause deletions – mutagenic. repeated skin damage due to sunburn melanoma PHYSICAL MUTAGENS : HEAT High temperature may cause hydrolysis of the glycosidic bond between the base and deoxyribose. Abasic site is produced: apurinic/apyrimidinic site (AP site). Over 10 000 such sites are produced daily in a cell. Abasic sugar-phosphate units are unstable, and get degraded – one strand of the DNA ends up with a single nucleotide gap. Cells can repair this type of damage quickly and precisely, it is rarely mutagenic. EFFECTS OF GENOTOXIC ENVIRONMENTAL AGENTS: MAIN TYPES AGENT EFFECT ON THE LIVING ORGANISM Carcinogen Causes tumorigenic transformation (initiation of tumors) Oncogen Supports the proliferation, survival and evolution of tumor cells (after initiation) Mutagen Causes mutations (but not necessarily carcinogenic or oncogenic!) Teratogen Casuses developmental abnormalities (embryonic-fetal) Clastogen Causes chromosomal breaks MUTATIONS AND DNA REPAIR What is a mutation? Causes of mutations: - Spontaneous (e.g. replication error) - Chemical agents - Physical agents Effects of mutations - beneficial (genetic variability, antibody repertoire) - pathological (diseases, cancer) DNA repair - Excision repair– BER and NER - Mismatch repair – repairing replication errors - Repairing single- or double-strand DNA breaks -... etc. Diseases associated with DNA repair dysfunction POSSIBLE EFFECTS OF POINT MUTATIONS IN THE CODING DNA REGION Synonymous: different codon, encoding the same amino acid. Non-synonymous : different codon, encoding a different amino acid. Variable effect on protein function. Nonsense: the mutation creates a stop codon – may result in a shorter, truncated protein. Readthrough: the mutation eliminates a stop codon – the protein may become longer. EFFECTS OF INDEL MUTATIONS IN THE CODING REGION 1. 1-2 basepair deletions or insertions (or multiples of these): causes translation frameshift. All codons change after the indel, resulting in a garbled amino acid sequence, and usually in premature stop codon. SEE THE CAT? XS EET HEC AT? SEE ATH ECA T? EFFECTS OF INDEL MUTATIONS IN THE CODING REGION 2. 3 bp deletion or insertion (or multiples of these): removal of entire codon(s) or insertion of new codon(s) in the sequence. The amino acid sequence remains unchanged after the indel – less likely to affect the protein function. BIG CAT RAN OFF! XXX BIG CAT RAN OFF! BIG BAD CAT RAN OFF! MUTATIONS IN THE NON-CODING REGION MAY ALSO HAVE AN EFFECT ON CELLULAR FUNCTIONS Deletion, Aberrant transcription: mutation of different tissues, too much regulatory RNA regions No transcription The non-coding DNA contains many transcription regulatory regions. If these are mutated or deleted, specificity of transcription (hence, the production of the gene product/protein) may be altered: the gene product is abnormally produced in other tissues as well, or the gene is activated at the wrong time, or the transcriptional activity is too high/too low. Mutations in introns or at the exon/intron boundary may cause aberrant splicing, or can alter the tissue specificity of splicing. Aberrant splicing means that the introns are not or incorrectly removed – this may result in a truncated or longer protein, or in frameshift. EFFECT OF MUTATIONS ON MULTICELLULAR ORGANISMS Mutation in germline cells: may be inherited, unless it causes embryonic/fetal lethality. If the offspring carrying the mutation does not reach reproductive age, inheritance stops at the 1st generation. Mutation in somatic cells: not a problem if the mutant cell dies, since there are many similar cells in the body. Mutations causing uncontrolled proliferation of the cells are more dangerous, because tumors can be formed. Effect of the mutation on gene/gene product function: silent – detectable in the DNA sequence, no effect on function loss-of-function – for some genes this is a problem even if only one allele is dysfunctional. This is called haploinsufficiency, when both alleles need to be active to produce enough protein. For other genes both alleles have to lose function to create a problem (recessive effect). gain-of-function – dominant effect. a) Quantitative, e.g. increased activity of a mutant protein kinase is a problem even if only one allele is affected, b) the protein gains a novel, damaging function with the mutation, c) the mutant protein is produced in tissues other than normal. BENEFICIAL EFFECTS OF MUTATIONS Mutations are the driving force of evolution. Genetic diversity originating from mutations supports the survival of the human population in the changing environment. Mutations can be important at the individual level as well. Sequence of the immunoglobulin genes (encoding antibodies) changes dramatically during the activation and differentiation of immune cells: this supports the enormous variability of the antibody repertoire. Random recombination of certain immunoglobulin gene regions, and the hypermutation of the variable (V) regions produces a vast number of different antibodies. This is why the immune system can recognize so many different chemical structures, and mount an effective defense against them. The mutation rate of the V regions is 6-7 order of magnitudes higher than usual for our genome. Extra material MUTATIONS AND DNA REPAIR What is a mutation? Causes of mutations: - Spontaneous (e.g. replication error) - Chemical agents - Physical agents Effects of mutations - beneficial (genetic variability, antibody repertoire) - pathological (diseases, cancer) DNA repair - Excision repair– BER and NER - Mismatch repair – repairing replication errors - Repairing single- or double-strand DNA breaks -... etc. Diseases associated with DNA repair dysfunction MAIN TYPES OF DNA REPAIR Excision repair Mismatch repair Repair of strand breaks DNA REPAIR: Base excision repair Base excision repair (BER) DNA bases may be modified chemically due to deamination, alkylation or other reactions. Damaged bases are recognized by specialized DNA glycosylases, and cleaved off of the deoxyribose ring, producing an AP site (apurinic- apyrimidinic site). The AP endonuclease cuts the affected DNA strand at the AP site, and removes some nucleotides, including the AP nucleotide using its exonuclease activity. DNA polymerase β fills in the DNA polymerase β gap, then the fragment ends are DNA ligase joined covalently by a DNA ligase. DNA REPAIR: Nucleotide excision repair Nucleotide excision repair (NER) Damaged nucleotides distort the structure of the DNA double helix. Regardless of the type of damage, the aberrant structure is recognized by a 5' cleavage 3' cleavage protein complex, which cleaves one of the DNA strands 5' and 3' from the damaged site. Single-strand cleavage is Helicase, unwinding supported by helicases unwinding the DNA at the damaged site. Next an exonuclease removes 25-50 nucleotides, including the damaged ones. DNA polymerase δ or ε fills in DNA polymerase δ/ε the gap, then the fragment ends are DNA ligase joined covalently by a DNA ligase. MISMATCH REPAIR: IDENTIFICATION OF THE UNALTERED STRAND IS CRITICAL Incorrect basepairing is recognized by different MSH protein complexes – both single basepair mismatches, and short insertions/deletions. Single-strand cleavage Cleavage of the strand with the wrong nucleotide/indel 5' from the mismatch, then 5'-3' exonuclease digestion of the affected region, removing a short DNA fragment ssDNA binding proteins together with the mismatch/indel. The eukaryotic mechanism identifying the unaltered "good" strand is not fully understood. DNA polymerase fills in the gap, and DNA ligase joins the fragment ends. The mismatch repair system requires many additional proteins – only the main ones are shown. Modified from Gambhir N et al. DOI 10.1128/msphere.00087-22 REPAIR OF SINGLE-STRAND DNA BREAKS Oxidative stress in the cells (production of free radicals, reactive oxygen species) often causes single-strand breaks in the DNA. These breaks are usually not dangerous, since the integrity of the double helix is minimally affected, and the repair system is very effective. PARP1 (poly-ADP-ribose-polymerase-1) proteins mark and protect the single- stranded DNA regions. The gap is repaired by the enzymes of the BER system. REPAIR OF DOUBLE-STRAND DNA BREAKS Ionizing radiation, mutagens and even the replication may cause double-strand DNA breaks. It can be repaired by homologous recombination, or by non-homologous end joining as illustrated. MUTATIONS AND DNA REPAIR What is a mutation? Causes of mutations: - Spontaneous (e.g. replication error) - Chemical agents - Physical agents Effects of mutations - beneficial (genetic variability, antibody repertoire) - pathological (diseases, cancer) DNA repair - Excision repair– BER and NER - Mismatch repair – repairing replication errors - Repairing single- or double-strand DNA breaks -... etc. Diseases associated with DNA repair dysfunction DNA REPAIR DISEASES Often associated with increased cancer risk. Examples: Ataxia telangiectasia – loss-of-function mutation in the ATM gene (encodes a protein activating DNA repair) Werner syndrome (progeria) – mutation of a helicase/exonuclease, which is required for telomer maintenance as well. Xeroderma pigmentosum – sun disease, dysfunction of the system repairing DNA damage caused by UV light. ataxia telangiectasia xeroderma pigmentosum Werner syndrome PRACTICE QUESTIONS 1. Why is DNA replication "semiconservative"? 2. How is replication initiated? 3. What is the role of the primase and different DNA polymerases during replication? What is the leading strand and the lagging strand? What are the Okazaki fragments? 4. How does the telomerase complex work, and why is it needed? 5. What is the function of topoisomerases, and how do they work? 6. What are the mechanisms ensuring the high fidelity of DNS replication? 7. What is the definition of point mutations and indel mutations? 8. What are the causes of replication error? 9. What are the consequences of covalent modifications of bases and nucleotides by chemical or physical agents? 10. How do the intercalating agents work? 11. What is the effect of mutations on gene function? 12. Characteristics of base excision repair 13. Characteristics of nucleotide excision repair 14. Characteristics of mismatch repair