01 - Light and Radiation PDF - Lighting Design & Technology
Document Details
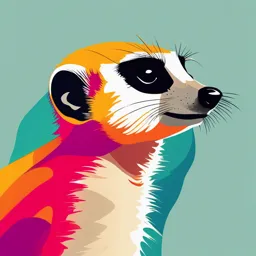
Uploaded by BrandNewTrumpet
Politecnico di Milano
2024
Chiara Bertolaja
Tags
Summary
This document is a presentation from the Specializing Master Lighting Design and Technology program at Politecnico di Milano. It introduces concepts such as light and radiation, detailing historical theories and exploring topics like wave and corpuscular theory. The presentation also covers electromagnetic radiation and the visual system.
Full Transcript
SPECIALIZING MASTER LIGHTING DESIGN AND TECHNOLOGY, 2024/2025 01 LIGHT AND RADIATION Arch. Chiara Bertolaja Prof. Andrea Siniscalco LIGHT Also known as the visible...
SPECIALIZING MASTER LIGHTING DESIGN AND TECHNOLOGY, 2024/2025 01 LIGHT AND RADIATION Arch. Chiara Bertolaja Prof. Andrea Siniscalco LIGHT Also known as the visible portion of a more wide electromagnetic spectrum. Through the years, scientists have tried to describe light, from Huygens and Newton up to Planck and Einstein, with various hypotheses and models. So, what is light? How do we perceive it? And most of all, how can we measure it to fit our purposes? Wave theory In 1690, the Dutch physicist, mathematician, astronomer and inventor Christiaan Huygens argued a theory describing light as a wave propagating through a fluid (the Aether) that permeated the universe. The Wave theory is regarded as the first mathematical theory of light. The theory was partially abandoned in favour of Newton’s corpuscular theory, only to be recovered thanks to the observations of the French physicist Augustin-Jean Fresnel that proved that Huygens’s theory Christiaan Huygens explained phenomena such as rectilinear propagation and diffraction of light. 1629 - 1695 Corpuscular theory Sir Isaac Newton stated that the geometric nature of reflection and refraction of light could only be explained if the light was made of particles, referred to as corpuscles. The theory was simple and straightforward but failed to explain phenomena such as diffraction, interference and polarization of light. For this reason, the idea was abandoned, only to be partially recovered later when the concept of Isaac Newton duality of light was postulated. 1642 - 1727 Electromagnetic radiation theory James Clerk Maxwell was a Scottish physicist who formulated the theory of electromagnetic radiation, bringing together electricity, magnetism, and light as different manifestations of the same phenomenon for the first time. The theory describes light as propagating a set of two waves (electric and magnetic) carrying electromagnetic radiant energy. The two fields are perpendicular to each other and perpendicular to the direction of propagation. Maxwell elaborated a series of four equations describing light's electromagnetic nature. James Clerk Maxwell 1831 - 1879 In 1887, Heinrich Rudolf Hertz conducted an experiment in which he discovered a phenomenon later called the “photoelectric effect”. When electromagnetic radiation hits a metal, electrons are emitted from its surface. The wave theory could not explain it. Quantum theory In 1900, the German theoretical physicist Max Planck studied the black body (an idealised physical body that absorbs all incident electromagnetic radiation) and postulated a theory that stated that electromagnetic energy could be emitted only in a quantised form (multiples of an elementary unit). The mathematics behind this theory laid the foundations of quantum mechanics. In the same years, Albert Einstein took up the concept of "quantum". He concluded that the energy carried by a "quantum of light" (later named "Photon") was equal to the Planck constant multiplied by the frequency of the radiation. This theory explains why very high-frequency radiation (such as x-rays or gamma rays) can transmit large amounts of energy while low-frequency ones carry Max Planck Albert Einstein smaller amounts. 1858 - 1947 1879 - 1955 Wave - Particle duality In his 1924 PhD thesis, the French physicist and mathematician Louis de Broglie hypothesised that electrons could have an undulatory nature associated with a wavelength. He then reworked Planck's theories and finally theorised that light (and matter as well) can behave both as a wave and particle. His ideas were confirmed experimentally in 1927 by Clinton Joseph Davisson and Lester Halbert Germer, who observed diffraction from a beam of electrons (particles), hence, what is now known as wave-particle duality was born. Louis de Broglie 1892 - 1987 Quantum electrodynamics In recent times, some physicists such as Paul Dirac, Wolfgang Pauli, and Werner Heisenberg formulated the quantisation of the electromagnetic field, giving rise to quantum electrodynamics (QED), which sees Richard Feynman among the best- known exponents. QED investigates the dynamics of the interaction between matter and light. Summarising everything, we can therefore describe light as a set of particles (photons) that have corpuscular behaviour on a Richard Feynman macro-scale. At the same time, on the 1918 - 1988 micro-scale (related to wavelengths), they also have the typical properties of waves. Light and visual system The visual sensation depends on the intensity and wavelength of the radiation and on how the eye responds to it Light and the visual system 380 nm 780 nm Light and visual system Within the visible range, eye sensitivity varies The eye responds to the various wavelengths enormously with different wavelengths of the same with a colour impression that goes from violet to energy content. For example, under the condition yellow, orange, and red with the increasing the of Photopic vision, the eye is about 20 times more wavelength value sensitive to light with a wavelength of 550nm (yellow) than to wavelengths of 700nm (deep red) or 450nm (violet-blue) Spectral eye sensitivity curve for photopic and scotopic vision according to CIE. The difference Visible range spectrum between the peaks is called the Purkinje shift. (between 380 nm and 780 nm) Luminous Efficacy (lm/W) Scotopic Photopic Light and visual system At 555 nm 1W gives 683 lm At the wavelength of 555nm, we have the maximum sensitivity of the human eye (100%) The maximum luminous flux of 683 lumen corresponds to this maximum eye sensitivity 780 Φ = K ∫ V (λ )Pλ dλ 380 K = maximum luminous efficacy (683 lm/W) Standard spectral eye sensitivity curve for Photopic vision (CIE 1932) V(λ) = adimensional multiplier related to the variation of human sensibility to different wavelengths. P = spectral power expressed in Watts dλ = considered the range of wavelengths Light sources A light source is an object capable of emitting a luminous flux This is possible generally because another form of energy is transformed into light energy. The light produced from the sources can be: 1. Natural (natural lighting) - direct sunlight - diffuse light from the sky 2. Artificial (artificial lighting) - light produced by the lighting fixtures Thermal radiation Energy distribution curves for thermal radiators (the black body radiator) of different temperatures from 2000 K to 7000 K When a solid body is heated to a temperature of about 525°C, it will begin to emit a dull red light. If the temperature increases, the colour will change from dull red to: 1. bright red, orange, 2. yellow, 3. white, 4. and finally blueish white The efficacy of such theoretical "metal" (i.e. the quantity of light that it can produce in relation to the total absorbed energy, and therefore also approximately the total radiated power) grows as the tip of the curve approaches the middle of the range of "visible radiations". The eye’s sensitivity reaches its maximum value (the V(λ) curve has its maximum for λ = 555 nm). The Correlated Colour Temperature CCT Intensity Intensity λ 500 1000 2000 λ 500 1000 1500 2000 The term colour temperature defines the colour impression obtained from an incandescent body of that temperature. At low temperatures, the high wavelengths are prevalent in the spectrum, giving the light a reddish appearance; at high temperatures, the low wavelengths are more prevalent, and the light has a white-light-blue hue. The Correlated Colour Temperature CCT The correlated colour temperature (CCT) of the light radiation of a source is expressed in Kelvin (K) and has no relationship with the actual temperature of the light source that emits IMPORTANT: the colour temperature is only a characteristic of white light sources and is not defined for the coloured light sources! The Correlated Colour Temperature CCT - Planckian locus An ideal black body (Plackian radiator) emits light as it is overheated. The colour shade of white light emitted is related to the temperature of this ideal radiator. This colour is measured in Kelvin (K). These colours can span from 1000 K (very warm) up to 20000 K (very cold) and more. By varying the temperature from 1.000 K to 20.000 K, the blackbody moves on a curve of the chromaticity diagram, the so-called Planckian locus (by the physicist Max Planck, who described the characteristics of the blackbody). If the colour of a light source deviates excessively from the Planckian locus, its colour temperature is not defined. In lighting, colour temperature is critical because of its impact on the colour appearance of illuminated objects. The 1931 CIE chromaticity diagram Light sources The energy emitted from a light source is expressed by the radiometric magnitude known as spectral radiant output spectral radiant output provides information on: how much energy is emitted (area under the spectrum) in which wavelengths (spectrum shape: colour) The spectral characteristics (colour of emitted light) of a source are defined by the distribution of relative power The Correlated Colour Temperature CCT Relative Spectral Power Distribution (SPD) of an incandescent lamp Comparison between the relative spectral power distribution of daylight and an incandescent lamp Extra LV Halogen Lamps Relative spectral power distribution of a Dichroic Halogen reflector lamp Colour Rendering Index (CRI) A measure of the degree of colour shift objects undergo when illuminated by a light source as compared with those same objects when illuminated by a reference source of comparable colour temperature. The reference source has a CRI of 100. Colour Rendering Index (CRI), Indicated by Ra or CRI; Describes the ability of a light source to visually render the colour of objects with respect to a reference source; It is calculated using 8 + 6 colour samples taken from the Munsell colour system; It can be generally associated with the fullness and continuity of the spectrum of the light source. Ra < 70 Ra > 80 Ra > 90 Colour Rendering Index (CRI), The colour rendering of a light source is evaluated with the CIE method: it consists of comparing the test source with a sample source (standard illuminants) having a colour temperature close to the test one. The sample source par excellence is natural light; it assumes different shades and, therefore, colour temperatures depending on the atmospheric conditions and the time of day. However, the chromatic aspect of the objects does not vary appreciably under the different conditions of natural light. Other sample sources used are incandescent lamps operating at very precise temperatures, alone or with additional filters. The comparison between the sample source and the source under examination is done by illuminating, alternately with one and the other, a unified series of eight coloured plates, chosen so that their colour points are well distributed in the CIE colour space and the results obtained coincide with a sufficient approximation with those deriving from the use of a much greater number of coloured plates. Colour Rendering Index (CRI) - Test colour samples The 14 Munsell colours used to calculate CRI: #1 - 7.5R 6/4 (Light greyish red), #2 - 5Y 6/4(Dark greyish yellow), #3 - 5GY 6/8 (Strong yellow green), #4 - 2.5G 6/6 (Moderate yellowish green), #5 - 10BG 6/4 (Light bluish green), #6 - 5PB 6/8 (Light blue), #7 - 2.5P 6/8 (Light violet), #8 - 10P 6/8 (Light reddish purple), and #9 - 4.5R 4/13 (Strong red), #10 - 5Y 8/10 (Strong yellow), #11 - 4.5G 5/8 (Strong green), #12 - 3PB 3/11 (Strong blue), #13 - 5YR 8/4 (Light yellowish pink), #14 - 5GY 4/4 (Moderate olive green). The first eight samples are relatively low saturated colours and are evenly distributed over the complete range of hues. These eight samples are employed to calculate the general Colour Rendering Index The last six samples provide supplementary information about the colour-rendering properties of the light source; the first four are for high saturation, and the last two are representatives of well-known objects (such as human skin and green leaves). Gaseous radiators The most effective way of making a gas emit light is by sending a stream of electrons through it. When one of these electrons hits an electron orbiting the gas atoms, this may be ejected to an orbit farther from the nucleus than the stable orbit belonging to that electron. The electron, going back to his stable orbit, releases a defined amount of energy in the form of a flash of electromagnetic radiation. The radiation emitted by gasses does not display a continuous spectrum, spreading over the visible and the adjacent IR and UV ranges, but is confined to several lines or bands of exactly defined wavelengths. CCT and CRI CCT and CRI CCT and CRI 830 The quality of light: comparing sources Efficacy Raising in Luminous Raising in the Power Efficacy (1) reduction the flux Source Type(3) Flux CRI source cost (2) (W) (lm/W) (2) cost (2) (lm) (%) (%) (%) 24 1750 65 85 24 1400 52 91 49 4300 78 85 - 18% +27% +56% 49 3500 64 91 LINEAR FLUORESCENT LAMP 54 4500 74 85 CCT: 4000 K 54 3800 62 91 18 1200 60 82 18 950 48 ≥ 95 24 1800 69 82 24 1500 58 ≥ 95 -18% +34% +63% 36 2900 76 82 COMPACT FLUORESCENT 36 2350 62 ≥ 95 LAMP 55 4800 79 82 CCT: 3000 K 55 4000 66 ≥ 95 35 3600 80 ≥ 80 35 2800 72 ≥ 90 -12% +34% +52% 70 7300 86 ≥ 80 METAL HALIDE LAMP CCT: 3000 K 70 6300 74 ≥ 90 1,05 124 118 80 1,05 107 102 85 -20% +44% +77% LED CREE XP-G3 1,05 100 95 95 CCT: 2700 K 1) The ratio between the emitted luminous flux and the nominal power 2) Considering the source with the highest CRI and the one with the lowest 3) Considering the sources with CRI and CCT shown in the table only Comparison between efficacies and costs of the most common lamps for home and offices ANSI/IES TM-30-20 The USA (IESNA) Technical Memorandum, TM-30 (recently updated) is an alternative to the EU Colour Rendering Index. It starts from the assumption that with the same level of CRI (that take in account a small number of samples) it is possible to tweak light in order to have different results. The TM-30 considers 99 samples. ANSI/IES TM-30-20 In order to measure color with the TM-30 it is necessary to consider: The Colour fidelity The accurate rendition of the colour so that they appear as they would under reference illuminants. This value is measured with the Fidelity Index (Rf), that can span from 0 to 100. The Color Gamut The average level of saturation relative to standard illuminants. This value is measured with the Gamut Index (Rg) that (when Rf is over 60), can have values from 40 to 140. Color Vector Graphic Together with the two indexes, the TM-30 gives also a visual help to better understand the differences (Hue changes and Saturation changes) of the Sample compared with the Reference. Increased saturation: the Color Quality Scale (CQS) The figures represent a setup of a real scene illuminated by two different sources: a) decreases the saturation of the colours; b) increases the saturation. Some studies have shown that observers tend to accept more sources that produce an increase of saturation (no distortion of tint) than those which determine a decrease. Other scales can be considered besides the CRI and the TM-30 (even though, from a standard point of view, the CRI is still official). For example, the Color Quality Scale considers not only colour fidelity but also colour preference and other aspects. They have the same value, but they are different… Let’s take two LED light sources that seem to be identical, but if we look at them, they are noticeably different. They have the same value, but they are different… If we measure the light with an appropriate instrument (a spectra-radiometer), we can see that while CRI, CCT and even the spectrum are nearly identical, there is one value that looks different. This value is the Duv and it measures how much the light stray from the planckian locus. The Planckian locus and the isotherms Along the curve of the planckian locus it is possible to draw straight lines in correspondence of the CCT. These lines are called isotherms. Along these isotherms it is possible to evaluate the Duv. Color consistency If you buy a stock of LEDs it is not said that all of them are perfectly identical. For sure quality helps, but in the end, it might happen to finish in situations like these: Same type of LED, same producer, same model, but dramatically different results. BINNING = Selection and aggregation of LEDs with homogeneous characteristics, done by manufacturers. Perceptual disuniformity of the CIE diagram MacAdam’s ellipses There are regions of the chromaticity diagram in which the visual system does not distinguish color differences. Within each ellipse we do not see color differences. On the left, the Mc Adam ellipses shown on the CIE chromaticity diagram of 1931. On the right an enlarged ellipse. The steps of difference are always referred to the central target. For example, point A is one step from the target but two steps from B. In practice, the points on an eclipse (e.g. C and D) are three steps from the target but not between them. The quality of light: the need to recognise colours The quality of light: the need to recognise colours The quality of light: the need to recognise colours The quality of light: the need to recognise colours The quality of light: the need to recognise colours www.bblighting.it The quality of light: the need to recognise colours www.bblighting.it The quality of light: choosing the lighting source The quality of light: choosing the lighting source Low pressure sodium lamp Relative spectral power distribution Main characteristics Very high luminous efficacy (up to 200 lm/W) long lifetime (10000 hours) Very warm light Warm colour light appearance CRI almost inexistent Application fields Every time the colour rendering is not important, while it is relevant containing the energy costs: lighting of highways, tunnels The quality of light: choosing the lighting source Low-pressure sodium LED "Good lighting is unnoticeable. Bad lighting is unmistakable.“ “La buona illuminazione è impercettibile. La cattiva illuminazione è inconfondibile." chiara bertolaja