Clinical Toxicology Lecture PDF (2021-2022)
Document Details
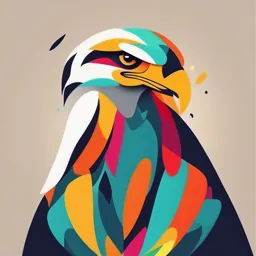
Uploaded by SpellboundYtterbium
Dr. Kassim Al-Saedi
Tags
Summary
These lecture notes cover basic principles of toxicology, focusing on factors influencing toxicity, such as composition, dose, and routes of administration. The document also addresses the metabolism of toxins and the importance of considering patient health conditions like liver or kidney issues in evaluating exposures.
Full Transcript
Lecture One in Clinical Toxicology ''Fifth Year Students'' First Semester (2021-2022) Basic Principles of Toxicology: Part I 1. Factors That Influence Toxicity. By Dr. Kassim Al-Saedi BSc Pharm., MS...
Lecture One in Clinical Toxicology ''Fifth Year Students'' First Semester (2021-2022) Basic Principles of Toxicology: Part I 1. Factors That Influence Toxicity. By Dr. Kassim Al-Saedi BSc Pharm., MSc.(Pharmacology& Toxicology), PhD (Pharmacology) - Clinical Toxicology: is involved with specific pathophysiologic changes caused by toxic agents and the management of these events. Clinical toxicology encompasses the study of chemicals originating from all sources. It is concerned with all aspects of the interaction between these chemicals and humans. - Factors Affecting Toxicity: 1- Composition of the Toxic Agent When examining a toxic episode, a basic fallacy is to view the responsible poison as a “ pure” substance. This implies that there are no contaminants present—that the vehicle, various adjuvants and excipients, and formulation ingredients are innocuous; the victim has not taken any drugs previously; and there is no batch-to-batch variation in the substance or product. The physicochemical composition of the toxic agent can sometimes be helpful in predicting the risk involved in exposure to a particular compound. In general, solids are less easily swallowed than are liquids, and bulky, high-density solids are more difficult to consume than are light, more fluffy compounds, especially for a small child. Thus, poisoning from the ingestion of bulky solids is less likely than is poisoning from liquids and small particles. These solid dosage forms can lodge in the oral cavity to cause local effects. The particulate size of the toxic agent is especially important in exposure by inhalation. Only particles with a small diameter (1 µm or less) will effectively reach the alveoli and be available for pulmonary absorption. Larger particles may be deposited on the walls of the throat and trachea to produce irritation or local injury to those tissues. The pH of the compound is another factor to consider. If the chemical is a strongly corrosive acid or alkali, obvious deleterious effects will occur with limited exposure of any tissue to the compound. On the other hand, the ingestion of mildly acidic or alkaline substances may cause little more than localized irritation. Breakdown of chemical during storage, sometimes a compound that was pure when packaged may have undergone a chemical change to produce an entirely different species capable of causing symptoms of toxicity that are unrelated to those expected from the original chemical. Paraldehyde, a liquid hypnotic, is an example. Overdoses of paraldehyde are characterized by central nervous system(CNS) depression. If paraldehyde is exposed to light and air, it may partially decompose to acetaldehyde. When pure acetaldehyde is ingested, nausea, severe reddening of the skin, coughing, and pulmonary edema are characteristically noted. 2- Dose and Concentration Nearly anything can be toxic at a given dose and route of administration. Conversely, even the most toxic substances may not be harmful at extremely low concentrations. The intravenous LD50 of distilled water in the mouse is 44 mL/kg and that of isotonic saline is 68 mL/kg. Neither distilled water nor isotonic saline is considered toxic; at a high enough volume or dose, however, toxicity becomes evident. 3- Routes of Administration The manner in which a potentially toxic substance enters the body can influence time of onset, intensity, and duration of toxic effects. The route of administration may also predict the degree of toxicity and possibly the target systems that will most readily be affected. A toxic chemical injected by the intravenous route would be expected to result in the most rapid onset of toxicity and the greatest potential for multiple organ exposure. When administered by other routes, the approximate descending order of toxicity is inhalation > intraperitoneal > subcutaneous > intramuscular > intradermal > oral > topical. 4- Metabolism of the Toxic Agent Metabolism of a toxic compound is usually the primary mechanism of detoxification. The metabolite produced is generally a more polar compound that can be readily excreted by the kidney. Unfortunately, this is not always the situation. Some chemicals are metabolized to compounds that are equally as active or sometimes even more active. Methanol is an example of such a chemical; it must first be oxidized to its intermediate metabolites, formaldehyde and formic acid, before it produces its most toxic effects. Chloral hydrate (sedative, hypnotic) , has been used as a hypnotic drug since the 1870s, principally for the short-term treatment of insomnia. It was also used to allay anxiety and to induce sedation and/or sleep post-operatively, before electroencephalogram evaluations, and to treat the symptoms of withdrawal of alcohol and other drugs such as opiates and barbiturates. For many years chloral hydrate was widely used for the sedation of children before diagnostic, dental or medical procedures, but although still in use, it has largely been replaced by newer drugs with a lower risk of overdose. After oral administration, chloral hydrate is converted rapidly to trichloroethanol, which is largely responsible for its hypnotic action. In humans, chloral hydrate is rapidly absorbed and then either oxidized to TCA (8%) or reduced to TCOH (92%), mainly by the liver, but also by the kidney. TCOH may be conjugated with glucuronic acid to form trichloroethanol glucuronide (TCOG), an inactive metabolite. Additional TCA is formed during enterohepatic circulation of TCOH, such that 35% of the initial dose of chloral hydrate is converted to TCA. The erythrocytes also metabolize chloral hydrate to TCOH, mainly via alcohol dehydrogenase. -The liver is the primary target organ of short-term exposure to chloral hydrate. The liver has been confirmed as the primary target organ of chloral hydrate toxicity in several long-term and carcinogenicity studies. Both TCA and TCOH are the major metabolites of chloralhydrate, Cytochrome P-450 (possibly the isoenzyme CYP2E1) was the enzyme responsible for the metabolic activation of chloral hydrate and its metabolites to TCA and TCOH. 5- State of Health: The presence of hepatic or renal disease may significantly affect the pharmacokinetics and outcome of exposure to a particular toxicant. Acidosis, from any cause, potentiates the action of tubocurarine and decreases the activity of insulin. Hypertensive patients may respond more intensely to chemicals that have sympathomimetic activity. Opioids and other respiratory depressants have greater toxicity potential in patients with head injuries. Psychotomimetics may provoke the recurrence of mental diseases in patients with histories of psychiatric disease. Disease states that cause diarrhea or constipation may decrease or increase the time of contact between chemical and absorptive sites and, thus, reduce or enhance absorption. 6- Age and Maturity The patient’s age must be considered as the extent of toxicity is assessed. Most anticipated toxic effects and reported prognoses are based on individuals who are neither too young nor too old. Unfortunately, not all victims of poisoning fall within this age group. The majority of accidental poisonings in fact occur in persons less than 5 years of age, and toxicity among the geriatric population is increasing. -Age as a major influence in determining extent of toxicity may be illustrated by considering the classic chloramphenicol-induced gray baby syndrome. In the early 1960s, it was a popular practice to administer chloramphenicol prophylactically to premature infants. It became apparent after several years that a number of infants receiving this antibiotic were showing signs of intoxication. Within days of treatment initiation, signs and symptoms of aplastic anemia—the “ gray baby’’ appearance, surfaced.( glucorunidation is not yet fully matured). 6-Nutritional State and Dietary Factors Certain nutritional factors, such as food or liquid contents of the stomach (e.g., acidic or alkaline, hot or cold, high fat or lean, high or low volume, and viscosity), are important to the absorption characteristics of many chemicals. In general, higher blood concentrations are achieved when drugs are taken on an empty as opposed to a full stomach. Certain foods may significantly increase or decrease drug absorption. For example, calcium may bind to tetracycline and reduce its absorption. Fatty foods, on the other hand, enhance griseofulvin absorption. -Individuals on starvation diets or those with low protein intake may have lower-than normal plasma levels of albumin.This may leave a proportionately greater amount of highly protein-bound drug in its free form. Since it is the free form of a drug (versus that which is protein bound) which causes toxicity, the drug will produce a greater degree of toxicity. Also, a low dietary protein intake may result in a decreased level of hepatic microsomal enzymes, resulting in decreased drug metabolism. 7-Genetics To illustrate the importance of pharmacogenetics as it relates to drug toxicity, consider the polymorphic expression of several enzymes that are linked to the metabolism of several compounds. Succinylcholine is a skeletal muscle relaxant that is often administered by infusion during the induction of general anesthesia. All skeletal muscle activity, including that of respiration, is depressed. Ordinarily the metabolism of succinylcholine proceeds. Most people quickly inactivate the drug by hydrolysis via plasma pseudocholinesterase to its first inactive metabolite, succinylmonocholine. The initial step proceeds rapidly and activity is lost within minutes. The toxicological problem arises because there is a segment of the population which exhibits unusual susceptibility to the effects of succinylcholine. The presence of an atypical pseudocholinesterase predisposes them to a prolonged and life- threatening paralysis of respiratory muscles because the initial detoxifying step in succinylcholine metabolism is hampered. Metabolism of succinylcholine in these individuals is slower, resulting in prolonged apnea and skeletal muscle relaxation that may last for several hours, even after discontinuation of infusion. 8-Gender (Sex): There were a sex related difference in absorption of erythromycin, resulting in less drug being absorbed by women after oral administration. Women were also reported to have lower serum phenytoin levels than did men because of an increased metabolic rate. Bioavailability of ethanol is greater in women than in men. This is associated with decreased gastric alcohol dehydrogenase activity in women, which contributes to reduced gastric oxidation of ethanol. This in turn may contribute to the enhanced vulnerability of women to acute and chronic complications of ethanol (IS). Although the pharmacologic activity and onset, as well as the severity of adverse reactions (e.g., nausea and vomiting), for these drugs may differ among the sexes, there is still little evidence that an acute toxic dose of either will produce significantly different toxic manifestations between men and women. Several other important differences should be considered. Men traditionally weigh more and have a greater blood volume and tissue mass than do women. Therefore, a given dose in a man would be expected to produce lower blood and tissue concentrations than the same dose taken by a woman. For substances injected intramuscularly, lower blood levels can be expected in individuals (usually men) with greater muscle mass. 9-Environmental Factors A- Temperature The response of a biologic system to a toxic agent generally decreases as environmental temperature is lowered, but the duration of overall response may be prolonged. This is related to a decreased rate of absorption and a lowered rate of metabolic degradation and excretion in colder environments. Additionally, some drugs are more toxic in certain environmental temperatures. Atropine like compounds may inhibit sweating and prevent cooling of the body. Anticholinergics may, therefore, produce significantly greater toxicity in a warm environment than in a colder one. Alternatively, drugs such as reserpine and chlorpromazine, which suppress the body’s thermal regulatory center, may be more toxic at certain temperatures. These drugs permit the body temperature to assume that of the environment. An individual’s body temperature will tend to rise in a warmer climate and decrease in a colder climate. B-Occupation Persons working in industries where organic compounds, such as chlorinated hydrocarbon pesticides or volatile substances, are used may have an enhanced ability to metabolize drugs and chemicals. The reason for this is that the chemical’s presence in the environment may have caused the induction of liver microsomal enzyme activity. The expected reaction to a toxic agent that is normally detoxified by the liver microsomal enzyme system would be reduced. C-Living Conditions The LD50 value for amphetamine is determined for mice placed individually in containers (such as coffee cans). If the number of animals placed in each container is increased, the LD50 decreases (i.e., the drug becomes more toxic). There seems to be a crowding factor related to living conditions that significantly affects the toxic dose of amphetamine. Animal studies have shown that amphetamines possess a property called “aggregation toxicity.” When an amphetamine is given in a low dose to a single animal such as a mouse, it induces little behavioral change. However, the same dose of drug given to a group of animals tends to produce hyperactive behavior, which may lead to death. Thus, amphetamines may affect the interaction between animals, and the circumstances of use can have an even greater effect than the dose. This may help to explain why some of these drugs have been considered as psychotherapeutic agents, and may be part of the reason why the mood-modifying amphetamines have come to be used as “dance drugs. Lecture One in Clinical Toxicology ''Fifth Year Students'' First Semester (2021-2022) Basic Principles of Toxicology: Part ''II'' 2. Principles in Management of the Poisoned Patient. By Dr. Kassim Al-Saedi BSc Pharm., MSc.(Pharmacology& Toxicology), PhD (Pharmacology) -Clinical Evaluation Of The Poisoned Patient A victim of poisoning must be carefully evaluated for extent of poisoning before a management plan can be initiated. The first step is to provide the patient with good supportive care. Is the patient breathing? Health care personnel may need to administer oxygen or start mechanical ventilation. Is the patient’s blood pressure stabilized? Shock is best treated with a fluid challenge and, if necessary, vasopressor agents. Is there normal sinus rhythm? Is the patient experiencing seizures or tremors? Is the patient comatose? Are there any other underlying medical problems, such as liver or renal disease? If so, the patient may require more aggressive therapy. After cardiorespiratory functions are supported, the next step is to obtain a history of the poisoning. 1- History of Poisoning An accurate history should include identification of the poison, amount and time of ingestion or length of contact, emergency first aid treatment already administered, and the patient’s psychologic profile. Obtaining the history is often difficult because the poisoned individual may be unconscious, unresponsive, or confused. Frequently, an accurate history may be impossible to obtain. Information obtained from relatives or friends is often (but not always) questionable. Symptoms may suggest one cause of poisoning, but the circumstances of poisoning may strongly indicate an entirely different cause. As a result, emergency medical personnel must make quick decisions about what to do and where to start in treating many victims. 2- Clinical Assessment Some poisons produce clinical characteristics that strongly suggest the involvement of a particular drug or chemical. For example, with cholinesterase-inhibiting organophosphorous insecticides, cholinergic effects such as miosis, excessive salivation, and gastrointestinal hyperactivity will predominate. In tricyclic antidepressant overdose, anticholinergic effects, such as mydriasis, loss of consciousness, absent bowel sounds, and cardiac arrhythmias, will dominate the clinical picture. Clinical assessment generally begins with recording of vital signs, such as blood pressure, heart rate, respirations, and body temperature. Assessment of neurologic function and level of consciousness is the next priority. Once emergency procedures have been performed and the poisoned patient is stabilized or at least is out of immediate danger, additional steps can be taken to remove the poison, prevent or delay absorption, enhance excretion, or administer a specific antidote. Another point to stress is the value in using blood, urine, and vomitus for toxicologic analysis. Qualitative and quantitative assays can quickly identify a toxic substance. The results of these tests can be used to aid diagnosis of the poisoned patient, evaluate the progress of treatment, and predict outcomes of therapy - Methods To Reduce Or Prevent Absorption: Gastrointestinal Decontamination Once the patient is stabilized, attention should be directed to removing any unabsorbed poison from the GI tract and other sites such as the skin. Severity of intoxication in many instances will be proportional to the length of time an unabsorbed toxic agent remains in the body. Gastric decontamination methods (emesis, gastric lavage, catharsis, and adsorption with activated charcoal) are undergoing critical scrutiny: A- Dilution The initial procedure often recommended whenever ingestion of a poison is suspected is dilution with water. The amount recommended is generally 1 to 2 cupfuls for a child and 2 to 3 cupfuls for an adult. However, fluids should never be forced. Offer the patient a quantity that can be comfortably swallowed. Excessive liquid may distend the stomach wall, causing premature evacuation of its contents into the duodenum and making it more difficult to remove the poison. A general rule is that nothing should be administered orally to an unconscious patient or if the gag reflex is absent. In cases of ingestion of solid dosage forms, such as tablets or capsules, dilution is not universally recommended. In this case dilution can promote dissolution of the medication, which favors absorption. Most chemicals and household products, such as cleaning agents, are best managed by dilution. Dilution with water accomplishes at least two functions. First, it helps reduce the gastric irritation induced by many ingested poisons. Second and more importantly, it adds bulk to the stomach that may be needed later for emesis. Ipecac-induced emesis is more effective if there is fluid or bulk present in the stomach. There is still some controversy over the use of milk as a diluent and demulcent with ipecac. Previously, a study involving adult volunteers demonstrated that milk delayed the onset of emesis when given with ipecac. More recently, studies have demonstrated that milk does not interfere significantly with the onset of emesis when given with ipecac. B- Emesis For many years emesis has been a mainstay for treating the ingestion of toxic agents. Emetics have been used since earliest recorded history. Chemically induced emesis is generally accepted to be a first-line procedure in the management of poisonings because it can be easily done at home. The American Academy of Pediatrics recommends that ipecac syrup be available in all homes with young children to be used only after consultation with a poison information specialist. Certain precautions and contraindications for emesis are listed in table below. If the victim is unconscious, there is danger that vomitus may be aspirated into the lung and cause chemical pneumonitis. If the poison is a convulsant, forced emesis may precipitate seizures. In alert, conscious patients who have swallowed liquid hydrocarbons such as petroleum distillates (gasoline, etc.), the recommendation to induce emesis is dependent on the amount ingested and the relative toxicity of the hydrocarbon. Petroleum or pine distillates, for example, have a low viscosity and surface tension. These substances can be readily aspirated into the lungs during emesis to cause chemical pneumonitis. If the ingested poison is corrosive, emesis should be avoided because it may cause further damage to the esophagus and oral mucosa as the substance is brought up. The tissue damage induced by these substances is related, in part, to contact time between poison and tissue. Children under 6 months of age should not receive syrup of ipecac unless supervised by a physician. Their gag reflex is poorly developed , and emetics may cause choking with aspiration. For children under 6 months, lavage is preferred. Emetics should be used with care in patients with severe cardiovascular disease, aneurysms, severe emphysema, or other conditions where blood vessels are weakened and there is risk of hemorrhage. Vomiting should be induced only if there is sufficient bulk (fluid) in the stomach to serve as a carrier for the ingested poison. Adequate dilution with water increases the efficacy of emetics, as was stated earlier. -Types of Emetic Agents Used to Induce Emesis: 1-Ipecac is derived from the root of Cephaelis ipecacuanha or C. acuminata. The principal active alkaloids are emetine and cephaeline. Ipecac causes emesis through both early and late phases of vomiting (SO). Early vomiting usually occurs within 30 min and is due to direct stimulant action on the GI tract. A second phase occurs after 30 min, resulting from direct stimulant action on the chemoreceptor trigger zone that activates the vomiting center located in the reticular formation. This effectiveness coordinated somatic and visceral motor activity to produce vomiting. Therefore, if vomiting does not occur within the first 15 to 20 min, the drug should not be discounted as ineffective. Syrup of ipecac is generally safe and well tolerated, and toxicity is rarely seen in doses.Some of its adverse effects may include protracted vomiting, diarrhea, lethargy, diaphoresis, and fever. The toxic component of ipecac is emetine, a cardiotoxin. Chronic abuse of ipecac in patients with anorexia nervosa or bulimia has resulted in peripheral myopathies and sometimes fatal cardiomyopathies. There are reports of serious adverse effects associated with long-term abuse of syrup of ipecac. These cases demonstrate that significant cumulative toxicity can result from repeated ingestion of a substance that is ordinarily not toxic in doses normally ingested acutely. There is no evidence that routinely associates therapeutic doses of ipecac with cardiovascular or neurotoxicity. - General Considerations for Using Syrup of Ipecac: Ipecac can be given at home by parents after they receive proper instructions from a qualified health professional. Because it may take approximately 20 to 30 min after administration for vomiting to begin, early administration is essential (?). Emergency medical vehicles usually stock syrup of ipecac. If it takes 10 min for the vehicle to reach a home, plus another 20 to 30 min to induce vomiting, serious poisoning may occur within this latent period. Worse, if treatment is not instituted until the victim arrives at the hospital, even more valuable time will be lost and the potential for damage accentuated. Therefore, parents of small children should be strongly urged to keep syrup of ipecac on hand at all times but use it only when directed by the poison control center. Another reason for early use of syrup of ipecac in poisonings is that induced emesis is generally less traumatic to the patient than gastric lavage. The insertion of a lavage tube is unpleasant to most people, especially children, and must be undertaken only by qualified personnel. Lavage is slow, is relatively inefficient, and may result in forcing material out of the stomach into the intestine, where it cannot be easily recovered. Chemical emetics can also recover particles of material that are too large to pass through the opening of a lavage tube. 2- Apomorphine Apomorphine is a morphine derivative that produces rapid emesis, usually within 3 to 5 min, through direct stimulation of the chemoreceptor trigger zone. Onset of emesis is more rapid, but recovery of gastric contents is essentially the same as with ipecac. It is no longer recommended. 3- Soap Solution When rapid emesis is indicated and syrup of ipecac is not available, one alternative is to administer a dish-washing liquid detergent. Two to three table spoonful should be mixed with 180 ml to 240 ml of water. (Detergents are believed to produce emesis by direct stimulation of the gastrointestinal mucosa. In one study, the mean time to emesis was less than 10 min, compared to 15 to 20 min for syrup of ipecac. The solution is difficult to swallow, so this method usually has poor patient acceptance. 4- Mechanical Stimulation Stimulation of the back of the tongue or pharynx has been recommended as a means to evoke emesis. This involves placing the victim in a spanking position and gently stroking the area with a blunt object such as a spoon or tongue depressor. Fingers are not advised, since the victim may reflexively bite down as he or she is gagged, causing injury to the individual’s finger. The advantage of this procedure is its availability. Its major disadvantage is lack of effectiveness; gagging is not the same as vomiting. In a study of 30 poisoned children, only 2 children vomited after mechanical stimulation, and the volume was insignificant (2 mL and 4 mL, respectively). All victims had previously been given 6 to 8 ounces of fluid. Consequently, mechanical stimulation is not recommended to induce vomiting. 5- Lavage Lavage (Fr. laver = to wash) is a process of washing out the stomach with solutions, including water, saline, sodium bicarbonate, calcium salts, tannic acid, and potassium permanganate. Lavage is sometimes indicated when poisons must be quickly removed from the stomach or when emesis is contraindicated. The patient’s airway should be protected by intubation, using a cuffed endotracheal or nasotracheal tube. Intubation should precede gastric lavage in the unconscious or convulsing patient. Any patient who can tolerate intubation without trauma should be intubated. Indications for intubation of poisoned patients are listed in Table 3.7. The patient is placed in the left lateral decubitus position to permit pooling of gastric contents and to reduce the risk of aspiration of gastric contents into the lungs. Also, the patient’s head should be slightly lower than the rest of the body. A large-volume reservoir is attached to the gastric hose. In some instances, aspiration of stomach contents with a syringe will remove large amounts of solid dosage forms. The aspirate should be saved for toxicologic analysis. Lavage is usually initiated using aliquots of tap water or normal saline. Saline is recommended in children to prevent electrolyte imbalance.Aliquots of 50 to 100 mL in children and 200 to 3(X) mL in adults should be instilled, allowed to mix, and then drained into a collection bag positioned below the patient. A minimum of 2 L are required to wash out most of the stomach contents. The consensus is to lavage the stomach until returned fluid is clear (i.e., keep introducing lavage solution into the stomach and withdrawing it until the return solution is clear). Caution must be exercised! There is always the possibility that, even though lavage was performec' until the returned fluid was clear, there may still be particles or clumps of chemicals [concretions (see chapter 2)] remaining in the stomach. This point will be illustrated in later chapters. Lavage is not always a procedure of first choice for removing ingested poisons and may be associated with numerous risks and complications. For example, the tube may be accidentally inserted into the trachea. Improperly used lavage can create a greater risk for pulmonary aspiration, esophageal perforation, or hastened gastric emptying time into the intestine. If a cool lavage solution is used too rapidly, the body’s core temperature may be dangerously lowered. Gastric lavage is contraindicated for the ingestion of most hydrocarbons, acids, alkali, and sharp objects. 6- Adsorbents Another means to reduce absorption of an ingested poison is by use of an adsorbent. Although several adsorptive substances, including kaolin, cholestyramine, pectin, and are occasionally recommended, they are not always effective binders of ingested chemicals. Activated charcoal is used for routine adsorption of gastrointestinal poisons. Others are useful with selected poisons. Fuller’s earth, for example, has a high affinity for the herbicide paraquat. Activated charcoal is a finely divided, black powder that is sparingly soluble in water. It is prepared by pyrolysis of organic materials, such as wood pulp. The activation process occurs when charcoal fragments are exposed to an oxidizing gas composed of steam or oxygen at temperatures of 600° to 900°C. Activated charcoal reduces absorption of a wide variety of poisons but has little or no effect on others (Table 3.8). In the stomach and intestine, poisons diffuse through the numerous pores on its surface to form tight chemical bonds. This charcoal-chemical complex then passes through the intestinal tract to reduce the chance of the chemical being absorbed. Effectiveness of adsorption is dependent on the quality of activated charcoal used and the time between ingestion and charcoal administration. Table Showing Properties Of Activated Charcoal Dose: Adult, 5 0 -1 0 0 g Child. 2 5 -5 0 g Infant, 1 g/kg Factors affecting efficacy Time since ingestion Charcoal :drug ratio Drug dose Stomach contents (pH, composition) Binds poorly to; Elemental metals (lead, lithium, boron, mercury) Boric acid Cyanide Electrolytes Ferrous sulfate Pesticides (malathion, DDT ,N-methylcarbamate) Petroleum distillates Ethanol Methanol Mineral acids, alkali Multiple oral doses useful with: Carbamazepine Dapsone Oigitoxin Nadolol Phenobarbital Phenylbutazone Theophylline NOTE: DDT, dichlorodiphenyltrichloroethane. Administration Of Activated Charcoal Activated charcoal is unsightly and readily adsorbs materials from the air and water when mixed and allowed to stand. Therefore, it should be mixed immediately before use with sufficient water, preferably in a dark container to keep youngsters from viewing it! Several commercial products contain a premeasured quantity in a tightly closed, opaque (nontransparent) plastic bottle. Water is added to the powder, the bottle is shaken vigorously, and the mixture is then administered directly from its container. Other preparations are ready-to use with sorbitol added. The use of multiple doses of 1 g of activated charcoal per kg every 2 to 4 hr has been repotted to enhance elimination of certain drugs, including aspirin, carbamazepine, cyclosporine, dextropropoxyphene, nadolol, phenobarbital, phenytoin, piroxicam, theophylline, digoxin, digitoxin, and tricyclic antidepressants.Proposed mechanisms for this effect include interruption of enterohepatic recirculation, adsorption of drug secreted across gastric membranes. 7- Cathartics Saline cathartics such as Magnesium sulfate, Magnesium citrate, Sodium sulfate, Sodium sulfate/sodium phosphate, and Sorbitol are preferred by most experts when catharsis is desired to remove toxic substances from the GI tract, although there have been few studies to evaluate their effectiveness. A rule of thumb is that, whenever contact time between the poison and absorption sites is reduced, the potential for toxicity will likewise be lessened. 8- Whole Bowel Irrigation The procedure is also used to cleanse the entire gastrointestinal tract before surgery. The solution most commonly used is a sodium sulfate and polyethylene glycol electrolyte solution that is not absorbed and does not lead to fluid or electrolyte imbalance. Experimental studies have shown decreased absorption for salicylates, lithium, and ampicillin. Whole bowel irrigation has also been used in overdoses of iron and zinc sulfate and in removing ingestions of cocaine packets. Whole bowel irrigation seems to be safe in children. 9- Demulcents Occasionally a demulcent is all that is needed to treat a poisoning. Many plants and chemicals cause oral and gastric mucosal irritation but no serious toxicity. Management of these acute ingestions may include ice cream, milk, or another soothing demulcent to reduce irritation. Egg whites , which serve as a source of readily available protein, have been given for corrosive intoxications and can be unofficially classed as a demulcent. 10- Topical Decontamination Numerous lipid-soluble chemicals can be absorbed through the skin and cause systemic toxicity within minutes. After dermal exposures, all contaminated clothing should be removed. Skin should be thoroughly flushed with water and washed with mild soap. -Methods To Increase Elimination Of Toxic Agents It was previously stated that repeated dosing with activated charcoal can reduce blood levels of certain drugs. Other methods of enhancing the elimination of a toxic agent include forced diuresis and pH alteration, peritoneal dialysis, hemodialysis, hemoperfusion, and the use of specific antibodies. After gastric decontamination, the next logical step in the management of a poisoned patient is to address methods for eliminating the toxic agent that has been absorbed into the blood. 1- Forced Diuresis and pH Alteration Forced diuresis was once recommended to help remove chemicals and drugs from blood. It is useful when compounds or active metabolites are eliminated by the kidney and diuresis enhances their excretion. Although many diuretic agents have been recommended over the years, either mannitol or furosemide was generally used. The use of these drugs in overdoses was fraught with complications, such as pulmonary and cerebral edema.. At best, forced diuresis may increase excretion of a chemical twofold. Many toxic compounds are weak acids or weak bases and in solution become ionized. Nonionized (nonpolar) compounds move easily across cell membranes, whereas ionized (polar) compounds are much less diffusible. The goal of urinary pH manipulation is to enhance renal excretion of a compound by increasing the amount of the ionized (polar) form in the kidney. As toxic agents pass through the kidney, they are Altered, secreted, and reabsorbed across the tubular membrane. The ionized (polar) form is trapped in the renal tubule and excreted; the nonionized form is reabsorbed in the blood. The purpose of pH manipulation is to present to the kidney the compound in its ionized (polar) form so that renal elimination of that compound is enhanced. Ideally, increased elimination of weak acids will occur when urinary pH is more alkaline. Conversely, enhanced elimination of weak bases will occur when urinary pH is non-acidic. Alkaline Diuresis is achieved by administration of sodium bicarbonate, 1 to 2 tnEq/kg every 3 to 4 hr. The objective is to increase urinary pH to between 7 and 8. This will be virtually impossible unless any potassium chloride deficiency has been corrected. The potential uses of urine alkalinization have been with weak acids, such as salicylates,& phenobarbital. Acid Diuresis is possible by using ammonium chloride, 75 mg/kg/24 hr. The endpoint for acidification is a urinary pH between 5.5 and 6.0. At one time, acid diuresis was favored to increase the elimination of weak bases, such as amphetamines, phencyclidine, and quinidine. 2- Dialysis (Hemodialysis, & peritoneal dialysis),and Hemoperfusion The following procedures are limited in scope and not routinely performed for every toxic ingestion. They are used as adjuncts to management of severely intoxicated patients. Dialysis and perfusion methods should never replace the use of more specific treatment or antidotes. A patient with an acute opioid overdose, for example, is best treated with naloxone, the specific antidote. These procedures would be of little value in treating acute ingestion of cytotoxic poisons, such as cyanide, which produce toxic effects very rapidly, often within minutes. Furthermore, if the ingested drug has a high therapeutic index, these procedures would have questionable value. Dialysis is governed by the laws of osmosis. A diffusible chemical dissolved in water partitions across a semipermeable membrane, and the solution moves from an area of higher concentration (i.e., the blood) to one of lower concentration (i.e., a dialyzing solution). The utility of dialysis in treating drug and chemical intoxications has expanded over the past decade. Hemodialysis The same basic principles apply to hemodialysis (extracorporeal dialysis). For peritoneal dialysis, an in vivo (peritoneal) membrane is utilized; in hemodialysis, however, a cellophane bag (artificial kidney) forms the semipermeable membrane. Two catheters are inserted into the patient’s femoral vein, about 2 inches apart. Blood is pumped from one catheter through the dialysis unit, across the semipermeable membrane, and back through the other catheter. The procedure is usually continued for 6 to 8 hr. The solubilized chemical diffuses across the semipermeable membrane into the dialysis solution. Clearance of the toxic agent is based on differences in osmotic and concentration gradients. Peritoneal Dialysis is the most easily performed method and is associated with the lowest risk for complications. However, it is also the least effective method for removing most poisons. The procedure is undertaken by inserting a tube through a small incision made in the midabdominal area into the peritoneum. The peritoneal membrane serves as the semipermeable (dialyzing) membrane. In this way, the dialyzable chemical diffuses from blood across the peritoneal membrane into the dialyzing fluid (moves from an area of higher to lower concentration). A warmed sterile dialyzing solution (up to 2 L for adults and 1 L for children) is introduced into the peritoneal cavity over a period of 15 to 20 min. The fluid is left in place for 45 to 60 min for equilibration to occur and then removed. A fresh solution is reintroduced and the process repeated. Up to 30 L or more of dialysis fluid may be used. Hemoperfusion Hemoperfusion is significantly more effective than peritoneal dialysis and hemodialysis for removing intoxicating compounds, particularly those that are lipid soluble or protein bound or those that, for other reasons, are poorly dialyzable. Indications for hemoperfusion in severe intoxications are evaluated by two important criteria. The first consideration is whether the adsorbent will eliminate the chemical from the blood. Second, the volume of distribution must be small and the half-life of the intoxicant relatively long, so that the drug can continue to be drawn from the tissues to the blood and consequently removed. Administration Specific Antidotes Generally antidotes are classified into: 1- Chemical antidotes React with the poisonous chemical to produce a compound of lesser toxicity or one that is absorbed to lesser degree than the parent compound. In oxalic acid poisoning, absorption produces renal damage. Calcium salts react with oxalic acid to yield a poorly soluble compound, calcium oxalate, which passes through the intestines without being absorbed. 2- Receptor Antidotes compete with the poison for receptor sites. Examples include naloxone reversal of morphine-induced respiratory depression and cholinergic blockade by atropine. For atropine or other anticholinergic poisons, physostigmine is a specific antidote. Physostigmine is a reversible cholinesterase inhibitor. Although it does not directly block atropine’s effects by competing with it for muscarinic receptor sites, it inhibits the activity of plasma pseudocholinesterase. This inhibition results in increased acetylcholine levels, which then compete with atropine for its receptor sites. 3- Dispositional Antagonism Involves alteration of absorption, metabolism, distribution, or excretion of toxic agents to reduce the amount available to tissues. In acetaminophen overdose, for example, a toxic metabolite causes hepatotoxicity. Conversion of this toxic intermediate to a nontoxic form proceeds by conjugation with glutathione, a sulfhydiyl (-SH) group donor. When glutathione reserves are depleted, as occurs after massive overdoses of acetaminophen, toxic manifestations appear. N-Acetylcysteine is also a source of sulfhydryl groups, which serve the same function as endogenous glutathione. 4- Functional (physiologic) antagonist Acts on one biochemical system to produce effects that are opposite from those produced on another system. For example, during an anaphylactic reaction after administration of a drug, the individual experiences severe breathing difficulties, due in part to intense bronchoconstriction. Epinephrine reverses this effect, and breathing is normalized.