Chapter 3: Solar Features PDF
Document Details
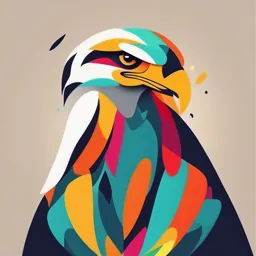
Uploaded by UnforgettableString
Tags
Summary
This document covers various features of the Sun, including photospheric, chromospheric, and coronal features. It details topics such as sunspots, faculae, granules, supergranules, filaments, prominences, and spicules. The document also references different articles related to these concepts.
Full Transcript
Chapter 3: SOLAR FEATURES Contents: 3.1 Photospheric features 3.1.1 Sunspots 3.1.2 Faculae 3.1.3 Granules 3.1.4 Supergranule 3.2 Chromospheric features 3.2.1 Chromospheric Network 3.2.2 Filaments 3....
Chapter 3: SOLAR FEATURES Contents: 3.1 Photospheric features 3.1.1 Sunspots 3.1.2 Faculae 3.1.3 Granules 3.1.4 Supergranule 3.2 Chromospheric features 3.2.1 Chromospheric Network 3.2.2 Filaments 3.2.3 Plage 3.2.4 Prominences 3.2.5 Spicules 3.3 Coronal features 3.4 Solar wind feature 3.1 Photospheric Features 3.1.1 Sunspots Sunspots appear as dark spots on the surface of the Sun. Temperatures in the dark centers of sunspots drop to about 3700 K (compared to 5700 K for the surrounding photosphere). They typically last for several days, although very large ones may live for several weeks. Sunspots are magnetic regions on the Sun with magnetic field strengths thousands of times stronger than the Earth's magnetic field. Sunspots usually come in groups with two sets of spots. One set will have positive or north magnetic field while the other set will have negative or south magnetic field. The field is strongest in the darker parts of the sunspots - the umbra. The field is weaker and more horizontal in the lighter part - the penumbra. 3.1.2 Faculae Faculae are bright areas that are usually most easily seen near the limb, or edge, of the solar disk. These are also magnetic areas but the magnetic field is concentrated in much smaller bundles than in sunspots. While the sunspots tend to make the Sun look darker, the faculae make it look brighter. During a sunspot cycle the faculae actually win out over the sunspots and make the Sun appear slightly (about 0.1%) brighter at sunspot maximum that at sunspot minimum. 3.1.3 Granules Granules are small (about 1000 km across) cellular features that cover the entire Sun except for those areas covered by sunspots. These features are the tops of convection cells where hot fluid rises up from the interior in the bright areas, spreads out across the surface, cools and then sinks inward along the dark lanes. Individual granules last for only about 20 minutes. The granulation pattern is continually evolving as old granules are pushed aside by newly emerging ones (show a 470 kB MPEG movie from the Swedish Vacuum Solar Telescope http://solarscience.msfc.nasa.gov/images /SVST_granulation.mpg). The flow within the granules can reach supersonic speeds of more than 7 km/s (15,000 mph) and produce sonic "booms" and other noise that generates waves on the Sun's surface. 3.1.4 Supergranule Supergranules are much larger versions of granules (about 35,000 km across) but are best seen in measurements of the "Doppler shift" where light from material moving toward us is shifted to the blue while light from material moving away from us is shifted to the red. These features also cover the entire Sun and are continually evolving. Individual supergranules last for a day or two and have flow speeds of about 0.5 km/s (1000 mph). The fluid flows observed in supergranules carry magnetic field bundles to the edges of the cells where they produce the chromospheric network. Photospheric Features Review Articles The Magnetic Structure of Sunspots by Juan M. Borrero and Kiyoshi Ichimoto Solar Surface Magneto-Convection by Robert F. Stein Solar Surface Convection by Åke Nordlund, Robert F. Stein, and Martin Asplund The Sun's Supergranulation by Michel Rieutord and François Rincon 3.2 Chromospheric Features 3.2.1 The Chromospheric Network The chromospheric network is a web-like pattern most easily seen in the emissions of the red line of hydrogen (H-alpha) and the ultraviolet line of calcium (Ca II K - from calcium atoms with one electron removed). The network outlines the supergranule cells and is due to the presence of bundles of magnetic field lines that are concentrated there by the fluid motions in the supergranules. 3.2.2 Filaments and 3.2.3 Plage Filaments are dark, thread-like features seen in the red light of hydrogen (H-alpha). These are dense, somewhat cooler, clouds of material that are suspended above the solar surface by loops of magnetic field. Plage, the French word for beach, are bright patches surrounding sunspots that are best seen in H-alpha. Plage are also associated with concentrations of magnetic fields and form a part of the network of bright emissions that characterize the chromospheres. 3.2.4 Prominences (http://www-solar.mcs.st- and.ac.uk/~alan/sun_course/Introduction/Prominences.html Prominences are dense clouds of material suspended above the surface of the Sun by loops of magnetic field. Prominences and filaments are actually the same things except that prominences are seen projecting out above the limb, or edge, of the Sun. Both filaments and prominences can remain in a quiet or quiescent state for days or weeks. However, as the magnetic loops that support them slowly change, filaments and prominences can erupt and rise off of the Sun over the course of a few minutes or hours (4.0 MB MPEG movie of the "Granddaddy" prominence eruption of 1945). Quiescent prominences I Prominence Feet SOLAR PROMINENCES Of the many fascinating phenomena associated with the Sun, solar prominences surely rate amongst the most incredible and bewildering of the observed features there. Situated in the Sun's extremely hot and tenuous upper atmosphere, the solar corona, these structures are basically comprised of cool, dense plasma confined in the form of thin, vertical sheets. Typically they possess a temperature in the range of 5- 10,000 K and a particle density of about 10 m these quantities being approximately 100 times cooler and more dense than the corresponding values in the surrounding environment. Prominences may be broadly categorized into two classes: 'active' prominences and 'quiescent' prominences. The former class contains highly dynamic and eruptive events which last for only minutes or hours. These are generally associated with solar flares and are often fairly violent occurrences. Sometimes they are observed as material that is rapidly ejected into the corona (surges) with velocities of several hundred kilometers per second. On other occasions they are seen as well-organized loop-like structures. This occurs when they are trapped in closed magnetic loops and so the dense plasma is channeled along the magnetic field thus highlighting the loop. The plasma drains continuously down the legs of such loops towards the solar surface, the photosphere. Quiescent prominences II A Hedgerow prominence Quiescent prominences are extremely stable features and remain suspended in the corona where they slowly evolve and become more elongated over the course of several solar rotations (a timescale of a few months). This raises immediate questions concerned with their surprisingly prolonged equilibrium: What is keeping them up? Why don't these very dense structures simply fall back down to the photosphere under gravity? The clue to this equilibrium lies within the magnetic field, since all prominences are observed to lie along a magnetic polarity inversion line (P.I.L.). This is a location at which the magnetic field must be horizontal and so it is thought that the prominence is supported by the restoring forces of the magnetic field which acts in an upward direction to oppose and balance the downward force due to the weight of the prominence. If you have ever had the chance to sit in a hammock you may understand this effect by the following analogy. As you (attempt to) get into the hammock it will stretch and bow until the tension in the strings is just sufficient to balance your weight! The magnetic field in prominences is thought to provide a similar tension force on the dense plasma to hold it there in equilibrium. Another important question concerning prominences arises when considering the thermal equilibrium. How do they remain so (comparatively) cool despite the surrounding million degree temperatures of the corona? Again, the answer is probably related to the magnetic field which may act as a thermal blanket around the prominence and so shield it from the corona. Quiescent prominences III Polarity Inversion Line The magnetogram shows the photospheric magnetic field. The purple and pink colours represent different magnetic polarities and the boundary between north and south polarity it called the polarity inversion line. Not how the very long prominence in the H alpha image lines up exactly with the polarity inversion line on the magnetogram. Solar magnetogram The nature of sunspots was put on firmer physical grounds at the beginning of the 20 century, when it was determined spectroscopically that sunspots were the seat of strong, concentrated magnetic fields. The image on the right is a magnetogram. Magnetograms are synthetic images constructed by measuring the magnetic field along the line of sight at a given location on the solar surface, and assigning a color according to the field strength; the pink-red-yellow sequence corresponds to increasingly strong positive normal magnetic field component, or magnetic field-lines pointing away from the Sun, and the purple-blue-light blue sequence to increasingly strong negative field, i.e. magnetic field-lines pointing into the Sun. Upon comparing this magnetogram to the Calcium image on the left, one sees that regions of strongest magnetic fields (yellow and light blue on the magnetogram) always coincide with sunspots. Diffuse magnetic fields of lesser strengths are also present all over the solar surface, with moderately strong ( Gauss) fields most often associated with plages. Field strengths in sunspots are in the range 1000---4000 Gauss, with the stronger fields in the larger sunspots; this is much larger than the average 0.5 Gauss of the Earth's surface magnetic field. What is perhaps even more striking is the topological complexity of the solar magnetic field; Unlike on the Earth's surface where the essentially dipolar magnetic field defines rather unambiguously a magnetic north and south, on the solar surface a compass would be rather useless for orientation purposes. An erupting prominence I Although prominence equilibria are maintained for a long period of time, many quiescent prominences do ultimately erupt in a dramatic event lasting only a few hours. Incredibly, the eruption always occurs outwards, away from the surface, indicating that the magnetic field which originally supported the prominence has suddenly released its stored energy. This energy release is sufficient to drive the dense plasma outwards against the Sun's huge gravitational pull. After the eruption, most of the material does eventually fall back down towards the surface and the prominence will often reform again at the same location over the course of a few days. The prominence shown in the picture existed in a stable state for several months before erupting and disappearing from the solar corona in a matter of hours. This raises several questions for the theorist to answer. Firstly, why should a prominence exist for such a long period of time and secondly, why should it decide to erupt? We can attempt to answer these questions by looking at the stability properties of the magnetic field that is supporting the dense plasma against gravity. An erupting prominence II When a prominence erupts most of the prominence plasma itself is not lost from the Sun. However, a particularly violent eruption can drive overlying material outwards with enough force to escape the Sun in what is known as a 'coronal mass ejection'. This represents a major mass loss from the Sun and can lead to significant effects even at the Earth's orbit where, for example, an aurora may occur. These are just a few of the main observations and ideas connected with solar prominences but it should be noted that there are several other effects and questions relating to these features which need to be explained and answered before the theorist can even begin to understand the mysterious existence of these fascinating features! 3.2.5 Spicules Spicules are small, jet-like eruptions seen throughout the chromospheric network. They appear as short dark streaks in the H-alpha image above (National Solar Observatory/Sacramento Peak). They last but a few minutes but in the process eject material off of the surface and outward into the hot corona at speeds of 20 to 30 km/s. 3.3 Coronal Features 3.3.1 Helmet Streamers Helmet streamers are large cap-like coronal structures with long pointed peaks that usually overlie sunspots and active regions. We often find a prominence or filament lying at the base of these structures. Helmet streamers are formed by a network of magnetic loops that connect the sunspots in active regions and help suspend the prominence material above the solar surface. The closed magnetic field lines trap the electrically charged coronal gases to form these relatively dense structures. The pointed peaks are formed by the action of the solar wind blowing away from the Sun in the spaces between the streamers. 3.3.2 Polar Plumes Polar plumes are long thin streamers that project outward from the Sun's north and south poles. We often find bright areas at the footpoints of these features that are associated with small magnetic regions on the solar surface. These structures are associated with the "open" magnetic field lines at the Sun's poles. The plumes are formed by the action of the solar wind in much the same way as the peaks on the helmet streamers. 3.3.3 Coronal Loops Coronal loops are found around sunspots and in active regions. These structures are associated with the closed magnetic field lines that connect magnetic regions on the solar surface. Many coronal loops last for days or weeks but most change quite rapidly (10 Mb Quicktime movie from TRACE observations). Some loops, however, are associated with solar flares and are visible for much shorter periods. These loops contain denser material than their surroundings. The three-dimensional structure and the dynamics of these loops is an area of active research. Fig. 1.2 in (The Sun from space, Kenneth R. Lang, 2nd edition, Springer-Verlag Berlin Heidelberg 2009, p 3) Coronal loops. The corona is stitched together with the ubiquitous coronal loops that are created when upwelling magnetic fields generated inside the Sun push through the photosphere into the overlying chromosphere and corona. These closed magnetic structures are anchored in the photosphere at footpoints of opposite magnetic polarity. Coronal loops can be filled with hot gas that shines brightly at extreme ultraviolet and X-ray wavelengths. Driven by motions in the underling photosphere and below, the coronal loops twist, rise, shear, writhe, and interact, releasing magnetic energy that can heat the solar corona and power intense solar flares or coronal mass ejections. Large coronal loops are found in the bulb-like base of coronal steamers, whose long, thin stalks extend out into space. Magnetic fields that are anchored in the photosphere at one end can also be carried by the solar wind into interplanetary space, resulting in open magnetic fields and a channel for the fast solar wind. 3.3.4 Coronal Holes Coronal holes are regions where the corona is dark. These features were discovered when X-ray telescopes were first flown above the earth's atmosphere to reveal the structure of the corona across the solar disc. Coronal holes are associated with "open" magnetic field lines and are often found at the Sun's poles. The high-speed solar wind is known to originate in coronal holes. 3.4 Solar Wind Features 3.4.1 Magnetic Clouds Magnetic Clouds are produced in the solar wind when solar eruptions (flares and coronal mass ejections) carry material off of the Sun along with embedded magnetic fields. These magnetic clouds can be detected in the solar wind through observations of the solar wind characteristics - wind speed, density, and magnetic field strength and direction. 3.4.2 Corotating Interactive Regions Co-rotating Interactive Regions (CIRs) are regions within the solar wind where streams of material moving at different speeds collide and interact with each other. The speed of the solar wind varies from less than 300 km/s (about half a million miles per hour) to over 800 km/s depending upon the conditions in the corona where the solar wind has its source. Low speed winds come from the regions above helmet streamers while high speed winds come from coronal holes. As the Sun rotates these various streams rotate as well (co-rotation) and produce a pattern in the solar wind much like that of a rotating lawn sprinkler. However, if a slow moving stream is followed by a fast moving stream the faster moving material will catch-up to the slower material and plow into it. This interaction produces shock waves that can accelerate particles to very high speeds. Composition Variations The chemical composition of the solar wind has several interesting aspects that hint at physical processes that occur in the solar wind source regions. The solar wind composition is different from the composition of the solar surface and shows variations that are associated with solar activity and solar features.